Abstract
[35S]Methionine labeling of intact barley roots (Hordeum vulgare cv Klondike) after short (6-12 h) and longer (18-24 and 90-96 h) periods of K+ deprivation revealed that several membrane polypeptides were synthesized in significantly increased amounts following withdrawal of K+ from nutrient solutions. One of these, a 43-kD polypeptide localized in plasma membrane- and tonoplast-enriched fractions, accounted for a large part of 35S incorporation into membranes when [35S]methionine was administered for 6 h following 6 h of K+ deprivation. With increasing duration of K+ deprivation, 35S incorporation into this 43-kD polypeptide decreased. This polypeptide, referred to as KR43, was not synthesized when NO3− or inorganic phosphate was removed or when Rb+ was substituted for K+. However, it was synthesized when K+ was removed and replaced by an equivalent concentration of Na+. The intrinsic nature of this polypeptide and the time course of changes in its expression, which correspond with changes of K+(86Rb) influx associated with K+ deprivation, provide evidence that this polypeptide may form part of the high-affinity K+ transport system in barley roots. A possible role for this polypeptide is discussed in the context of changes in the subcellular distribution of K+ in barley roots following interruption of K+ supply. A 45-kD microsomal polypeptide, identified in earlier studies as a response to K+ deprivation, is suggested to be an extrinsic protein, readily displaced from membranes by exposure to ethylenediaminetetraacetate.
Full text
PDF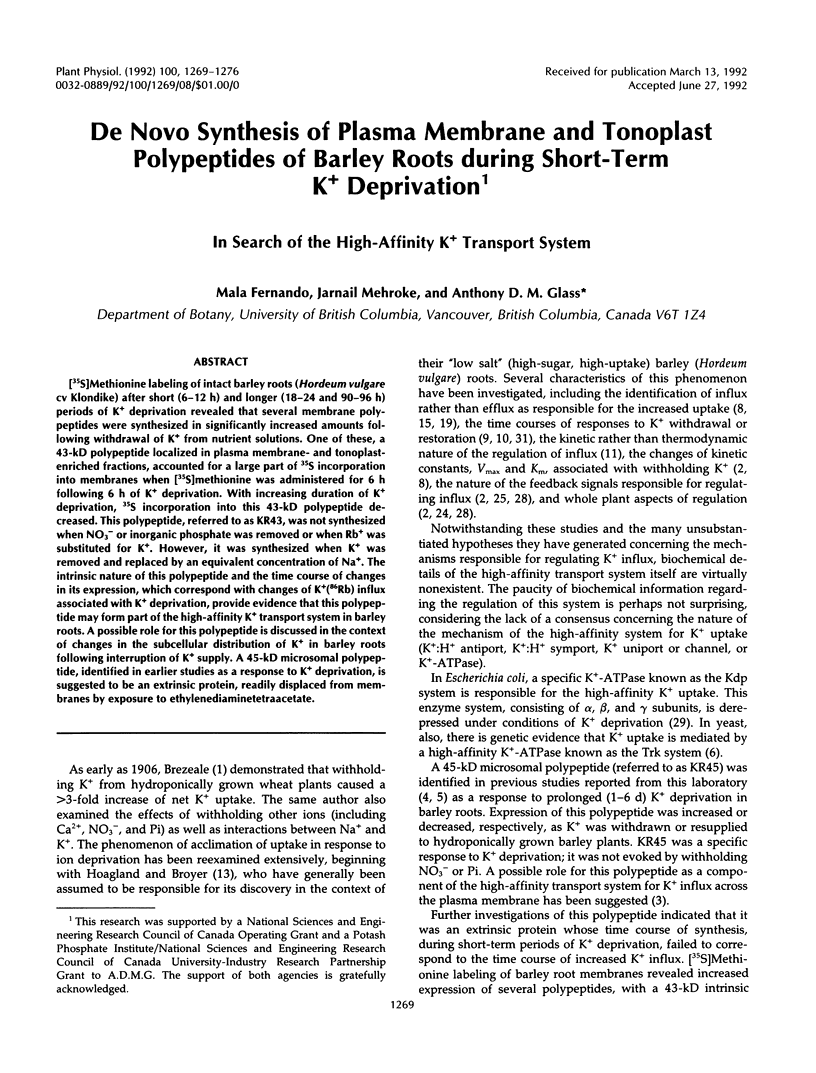
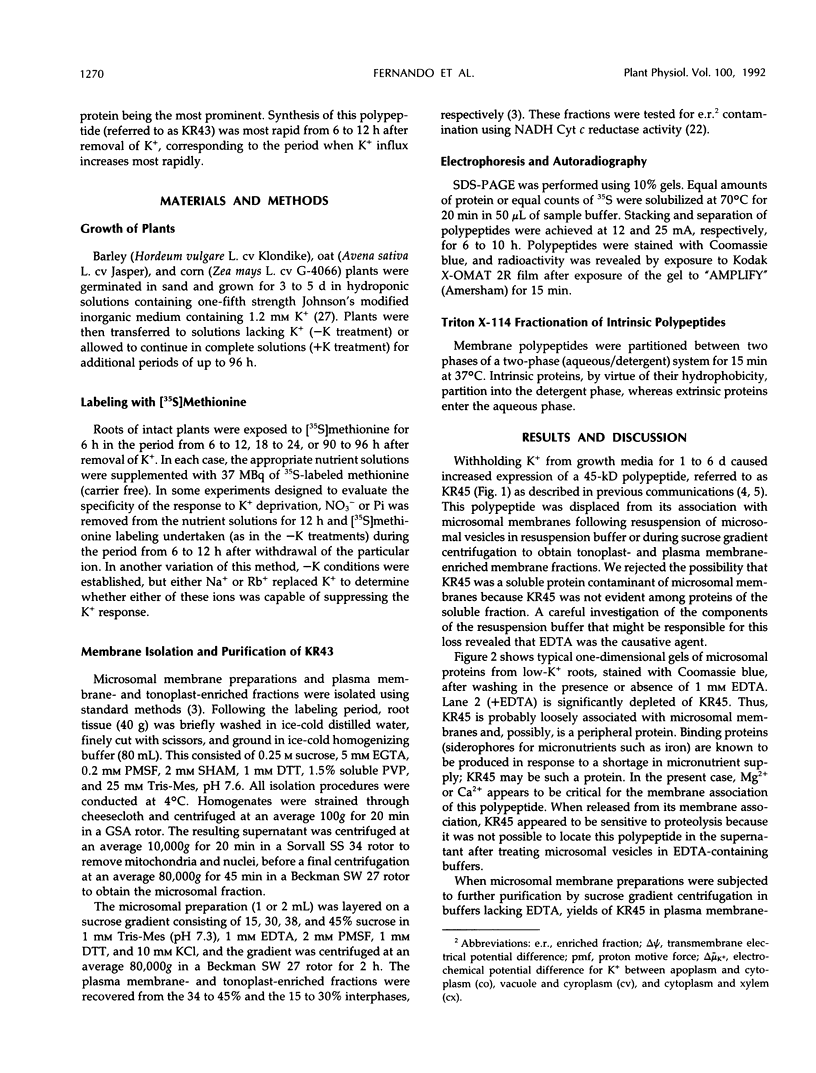
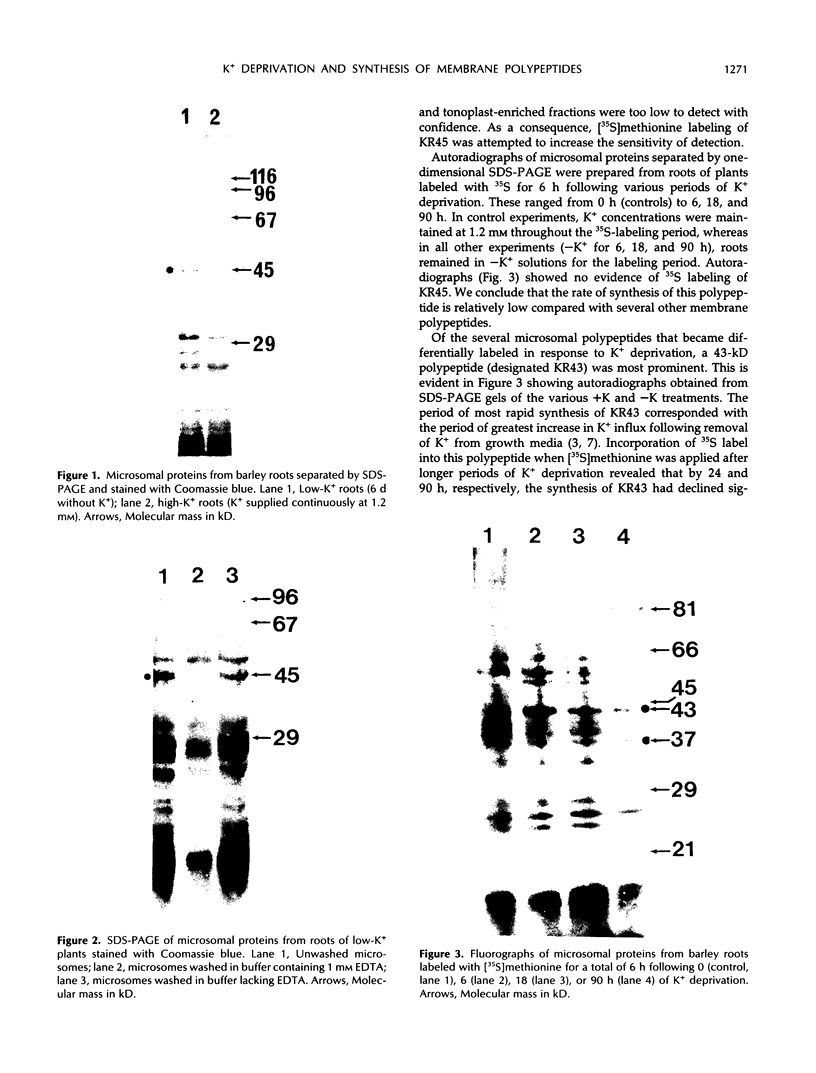
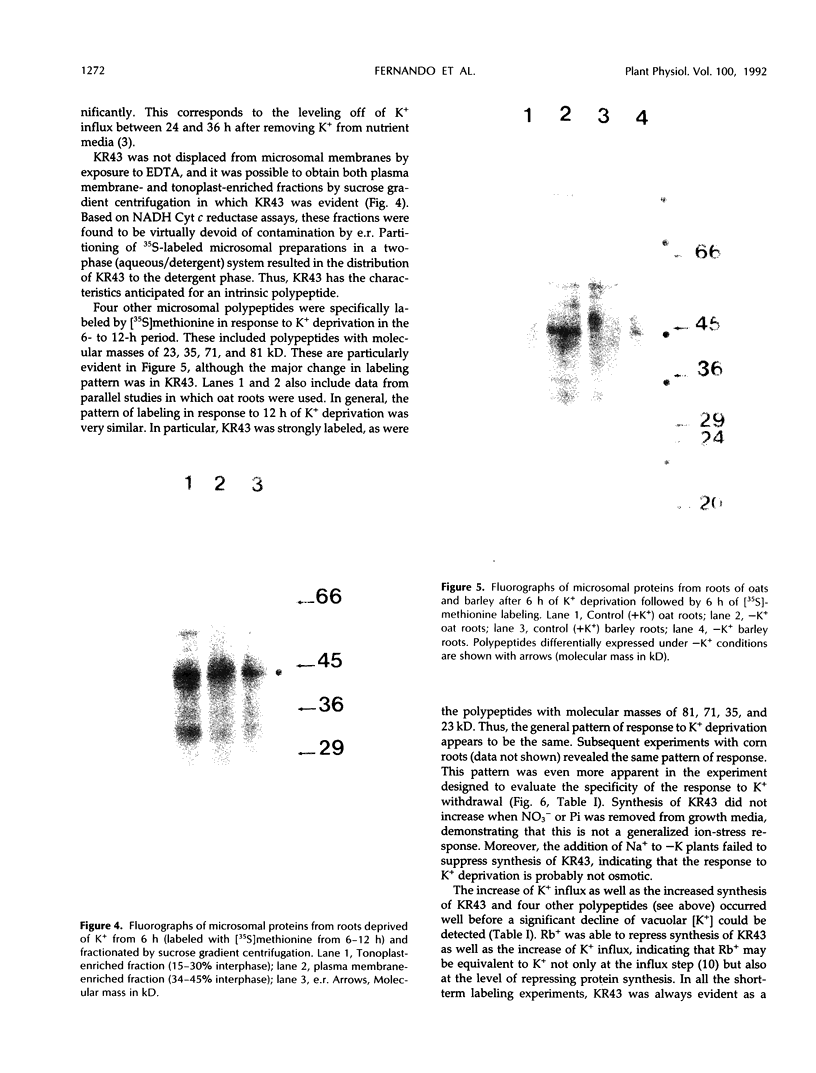
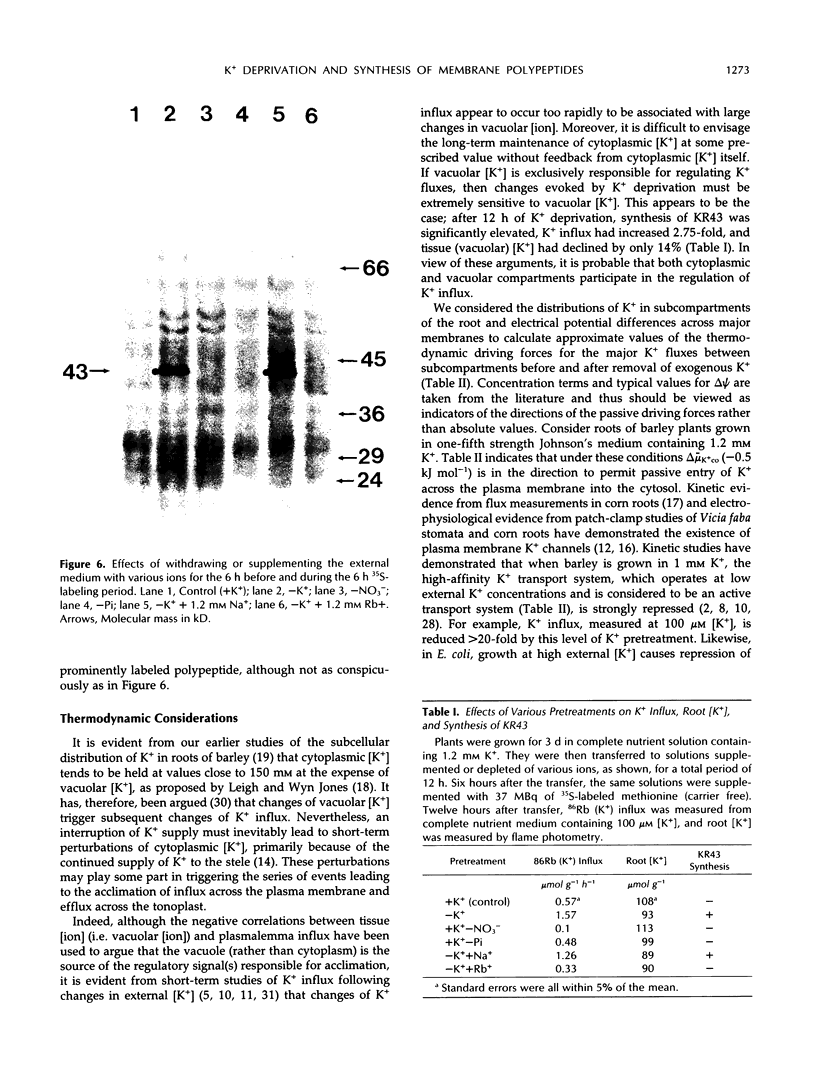
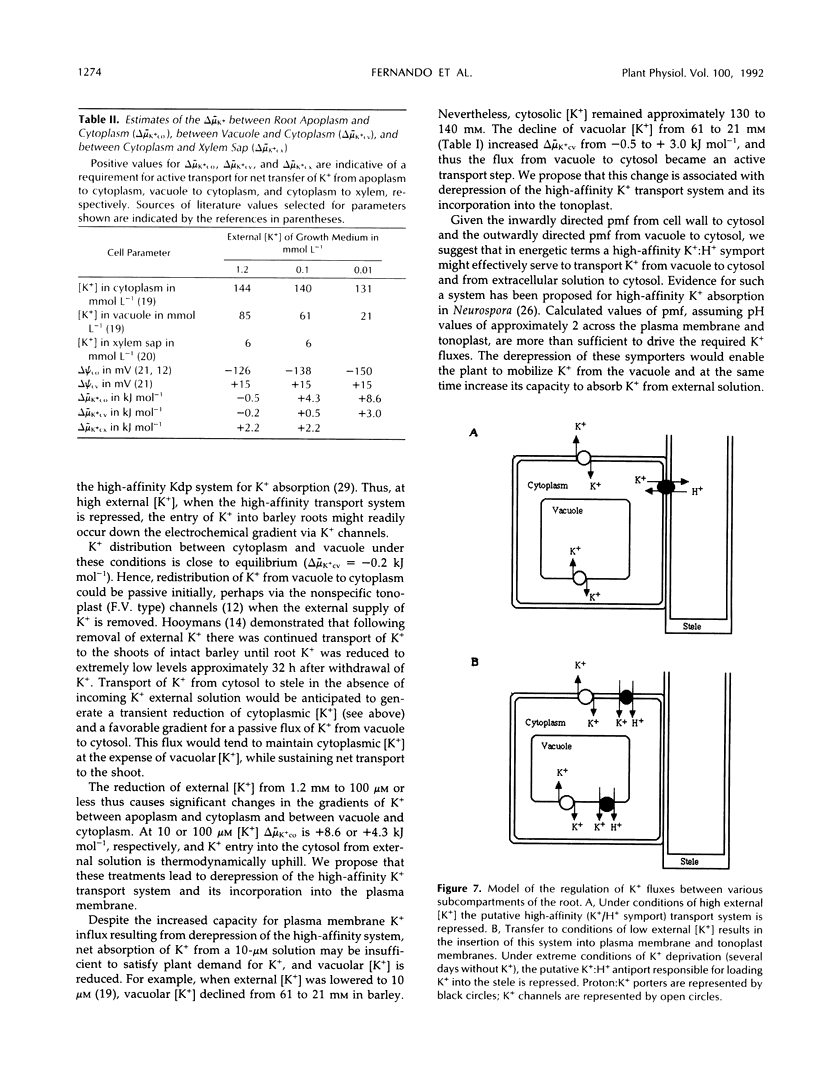
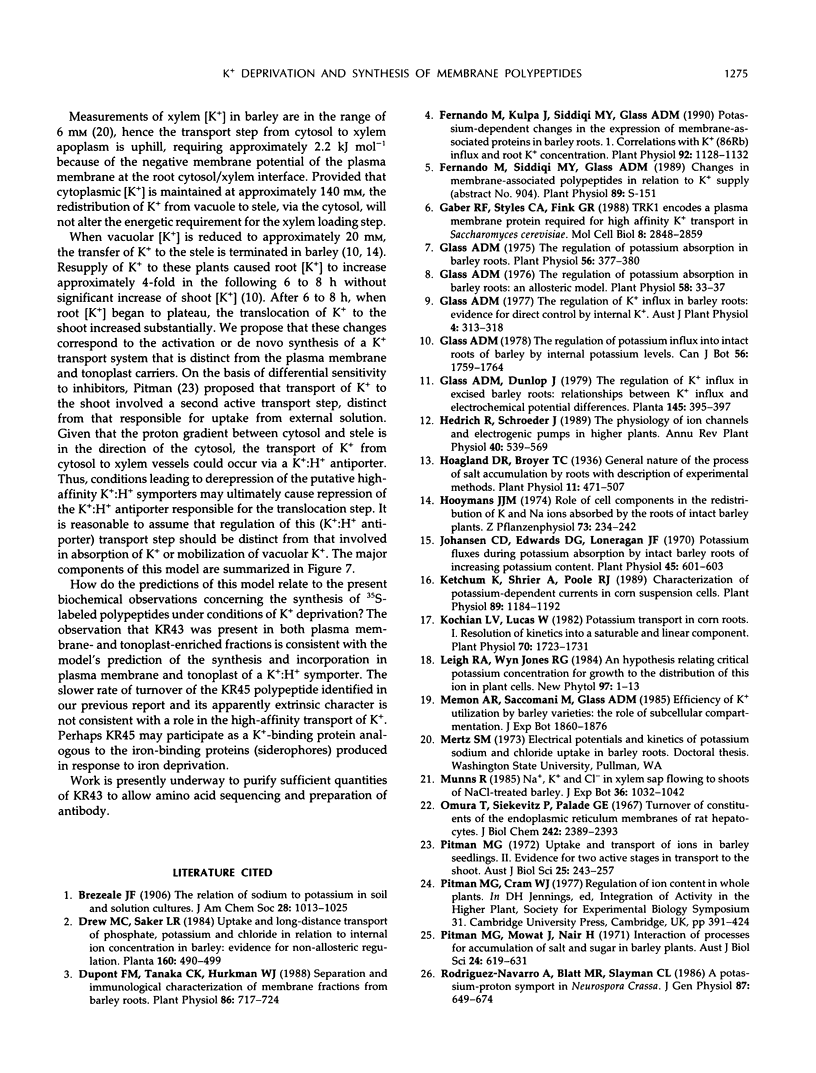
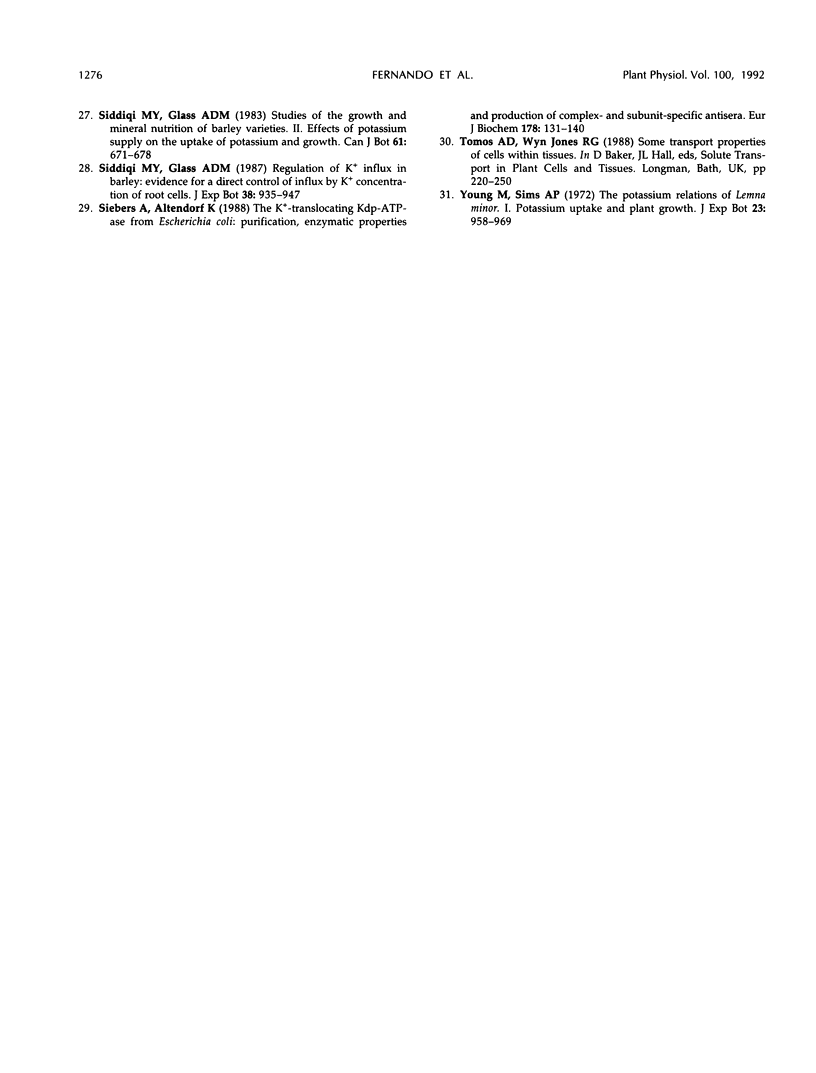
Images in this article
Selected References
These references are in PubMed. This may not be the complete list of references from this article.
- Dupont F. M., Tanaka C. K., Hurkman W. J. separation and Immunological Characterization of Membrane Fractions from Barley Roots. Plant Physiol. 1988 Mar;86(3):717–724. doi: 10.1104/pp.86.3.717. [DOI] [PMC free article] [PubMed] [Google Scholar]
- Fernando M., Kulpa J., Siddiqi M. Y., Glass A. D. Potassium-dependent changes in the expression of membrane-associated proteins in barley roots : I. Correlations with k(rb) influx and root k concentration. Plant Physiol. 1990 Apr;92(4):1128–1132. doi: 10.1104/pp.92.4.1128. [DOI] [PMC free article] [PubMed] [Google Scholar]
- Fett W. F., Dunn M. F. Exopolysaccharides Produced by Phytopathogenic Pseudomonas syringae Pathovars in Infected Leaves of Susceptible Hosts. Plant Physiol. 1989 Jan;89(1):5–9. doi: 10.1104/pp.89.1.5. [DOI] [PMC free article] [PubMed] [Google Scholar]
- Gaber R. F., Styles C. A., Fink G. R. TRK1 encodes a plasma membrane protein required for high-affinity potassium transport in Saccharomyces cerevisiae. Mol Cell Biol. 1988 Jul;8(7):2848–2859. doi: 10.1128/mcb.8.7.2848. [DOI] [PMC free article] [PubMed] [Google Scholar]
- Glass A. D. Regulation of potassium absorption in barley roots: an allosteric model. Plant Physiol. 1976 Jul;58(1):33–37. doi: 10.1104/pp.58.1.33. [DOI] [PMC free article] [PubMed] [Google Scholar]
- Glass A. The regulation of potassium absorption in barley roots. Plant Physiol. 1975 Sep;56(3):377–380. doi: 10.1104/pp.56.3.377. [DOI] [PMC free article] [PubMed] [Google Scholar]
- Hoagland D. R., Broyer T. C. GENERAL NATURE OF THE PROCESS OF SALT ACCUMULATION BY ROOTS WITH DESCRIPTION OF EXPERIMENTAL METHODS. Plant Physiol. 1936 Jul;11(3):471–507. doi: 10.1104/pp.11.3.471. [DOI] [PMC free article] [PubMed] [Google Scholar]
- Johansen C., Edwards D. G., Loneragan J. F. Potassium Fluxes during Potassium Absorption by Intact Barley Plants of Increasing Potassium Content. Plant Physiol. 1970 May;45(5):601–603. doi: 10.1104/pp.45.5.601. [DOI] [PMC free article] [PubMed] [Google Scholar]
- Ketchum K. A., Shrier A., Poole R. J. Characterization of potassium-dependent currents in protoplasts of corn suspension cells. Plant Physiol. 1989 Apr;89(4):1184–1192. doi: 10.1104/pp.89.4.1184. [DOI] [PMC free article] [PubMed] [Google Scholar]
- Kochian L. V., Lucas W. J. Potassium transport in corn roots : I. Resolution of kinetics into a saturable and linear component. Plant Physiol. 1982 Dec;70(6):1723–1731. doi: 10.1104/pp.70.6.1723. [DOI] [PMC free article] [PubMed] [Google Scholar]
- Omura T., Siekevitz P., Palade G. E. Turnover of constituents of the endoplasmic reticulum membranes of rat hepatocytes. J Biol Chem. 1967 May 25;242(10):2389–2396. [PubMed] [Google Scholar]
- Rodriguez-Navarro A., Blatt M. R., Slayman C. L. A potassium-proton symport in Neurospora crassa. J Gen Physiol. 1986 May;87(5):649–674. doi: 10.1085/jgp.87.5.649. [DOI] [PMC free article] [PubMed] [Google Scholar]