Abstract
Ferredoxin (Fd) in higher plants is encoded by a nuclear gene, synthesized in the cytoplasm as a larger precursor, and imported into the chloroplast, where it is proteolytically processed, and assembled with the [2Fe-2S] cluster. The final step in the biosynthetic pathway of Fd can be analyzed by a reconstitution system composed of isolated chloroplasts and [35S]cysteine, in which [35S]sulfide and iron are incorporated into Fd to build up the 35S-labeled Fe-S cluster. Although a lysed chloroplast system shows obligate requirements for ATP and NADPH, in vitro chemical reconstitution of the Fe-S cluster is generally thought to be energy-independent. The present study investigated whether ATP and NADPH in the chloroplast system of spinach (Spinacia oleracea) are involved in the supply of [35S]sulfide or iron, or in Fe-S cluster formation itself. [35S]Sulfide was liberated from [35S] cysteine in an NADPH-dependent manner, whereas ATP was not necessary for this process. This desulfhydration of [35S]cysteine occurred before the formation of the 35S-labeled Fe-S cluster, and the amount of radioactivity in [35S]sulfide was greater than that in 35S-labeled holo-Fd by a factor of more than 20. Addition of nonradioactive sulfide (Na2S) inhibited competitively formation of the 35S-labeled Fe-S cluster along with the addition of nonradioactive cysteine, indicating that some of the inorganic sulfide released from cysteine is incorporated into the Fe-S cluster of Fd. ATP hydrolysis was not involved in the production of inorganic sulfide or in the supply of iron for assembly into the Fe-S cluster. However, ATP-dependent Fe-S cluster formation was observed even in the presence of sufficient amounts of [35S]sulfide and iron. These results suggest a novel type of ATP-dependent in vivo Fe-S cluster formation that is distinct from in vitro chemical reconstitution. The implications of these results for the possible mechanisms of ATP-dependent Fe-S cluster formation are discussed.
Full text
PDF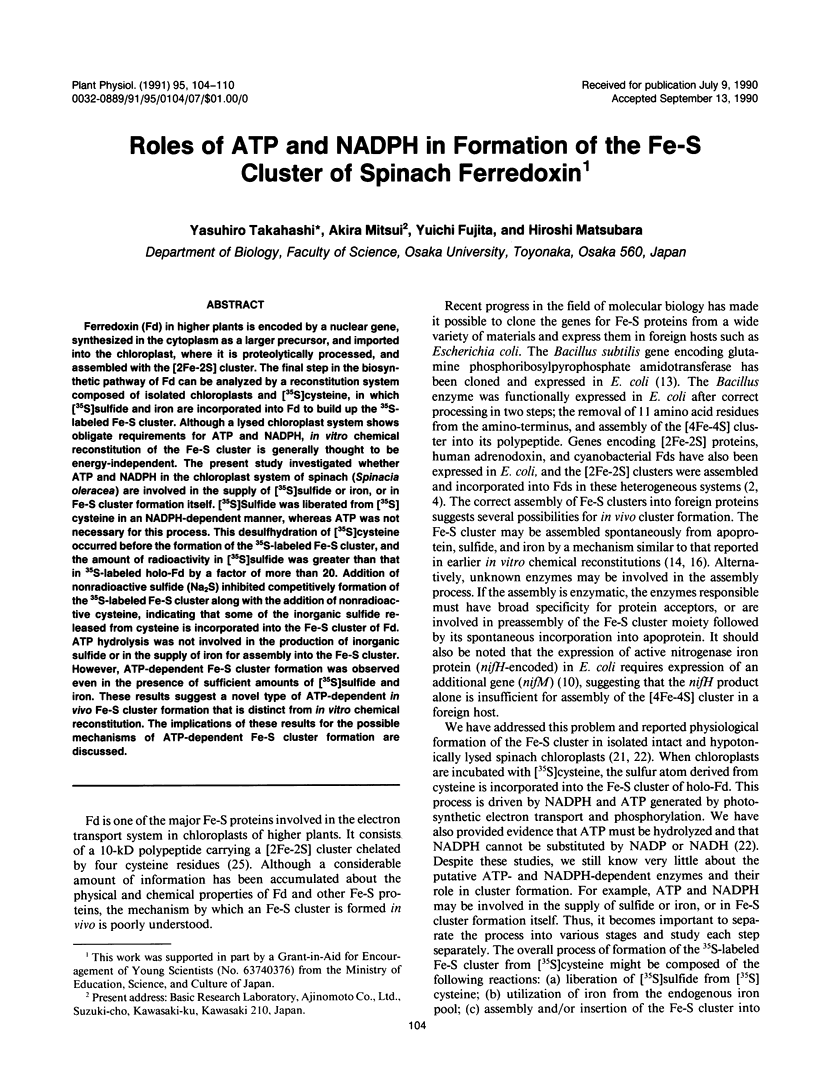
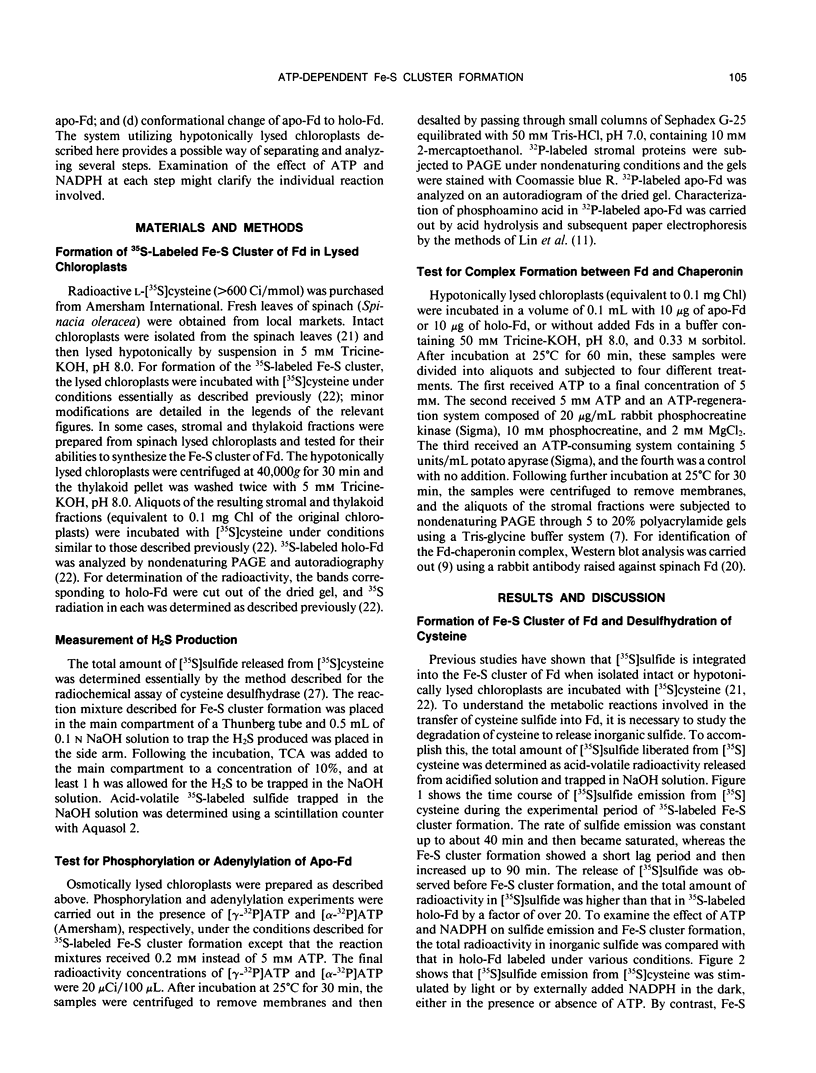
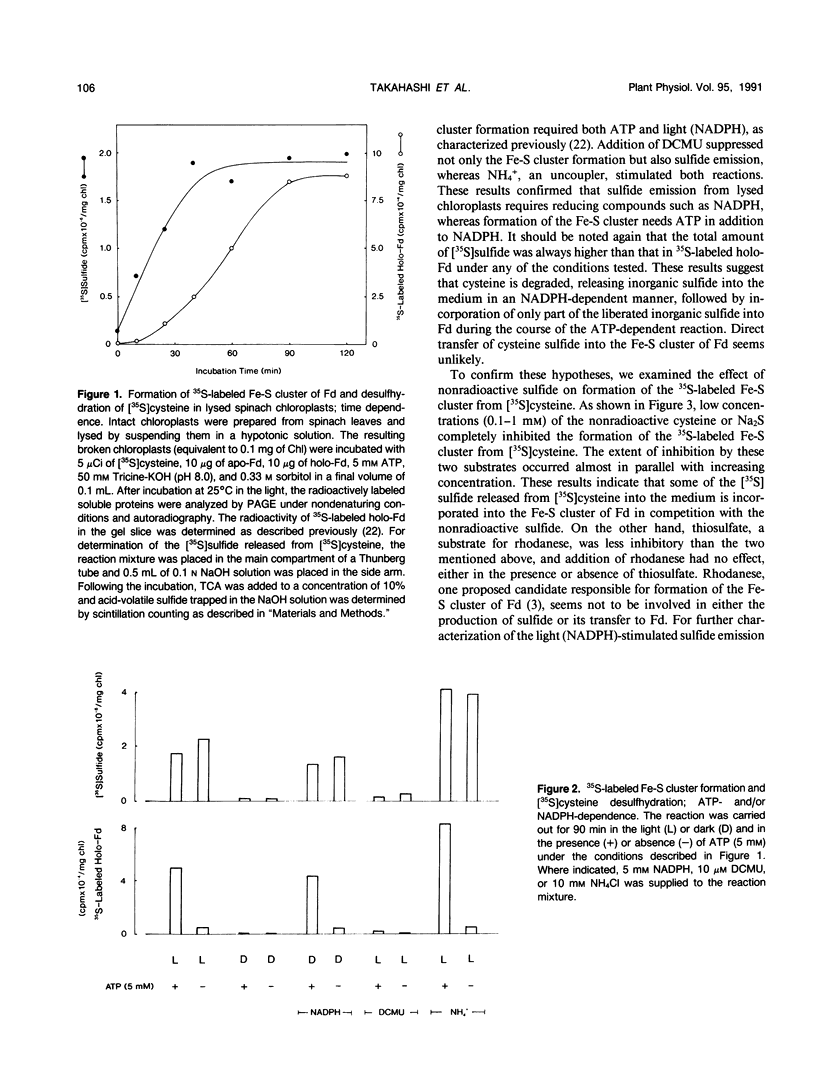
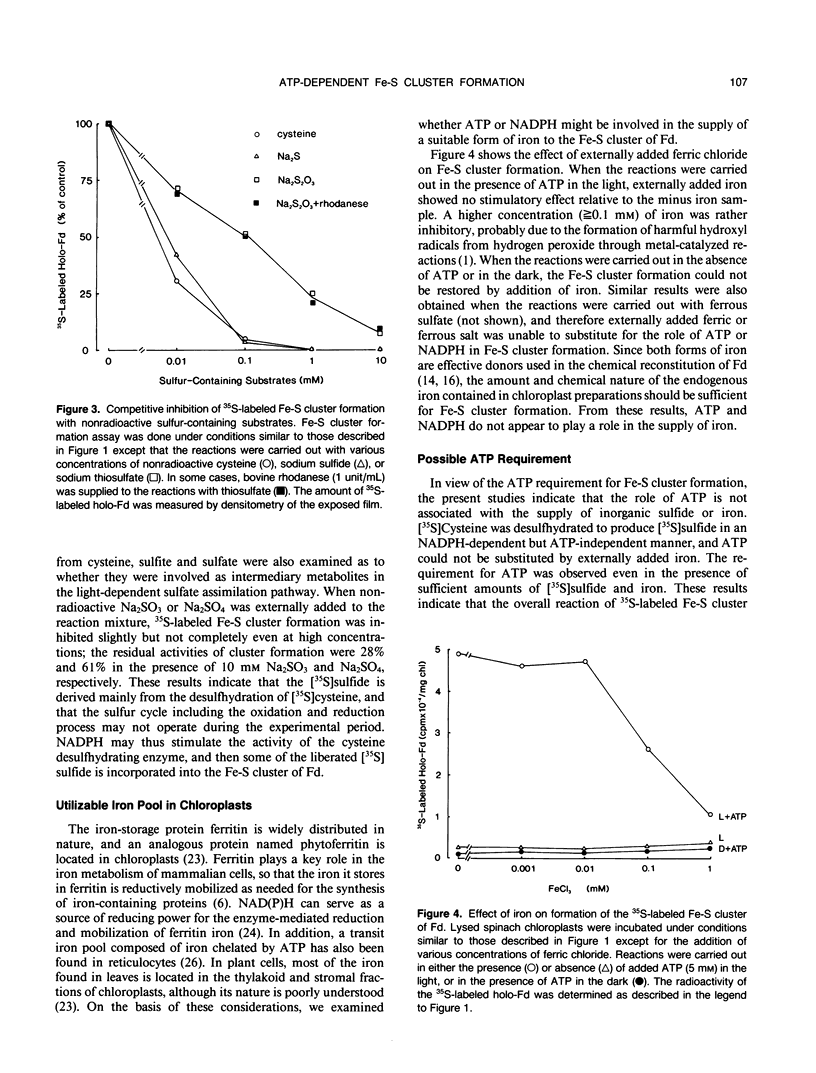
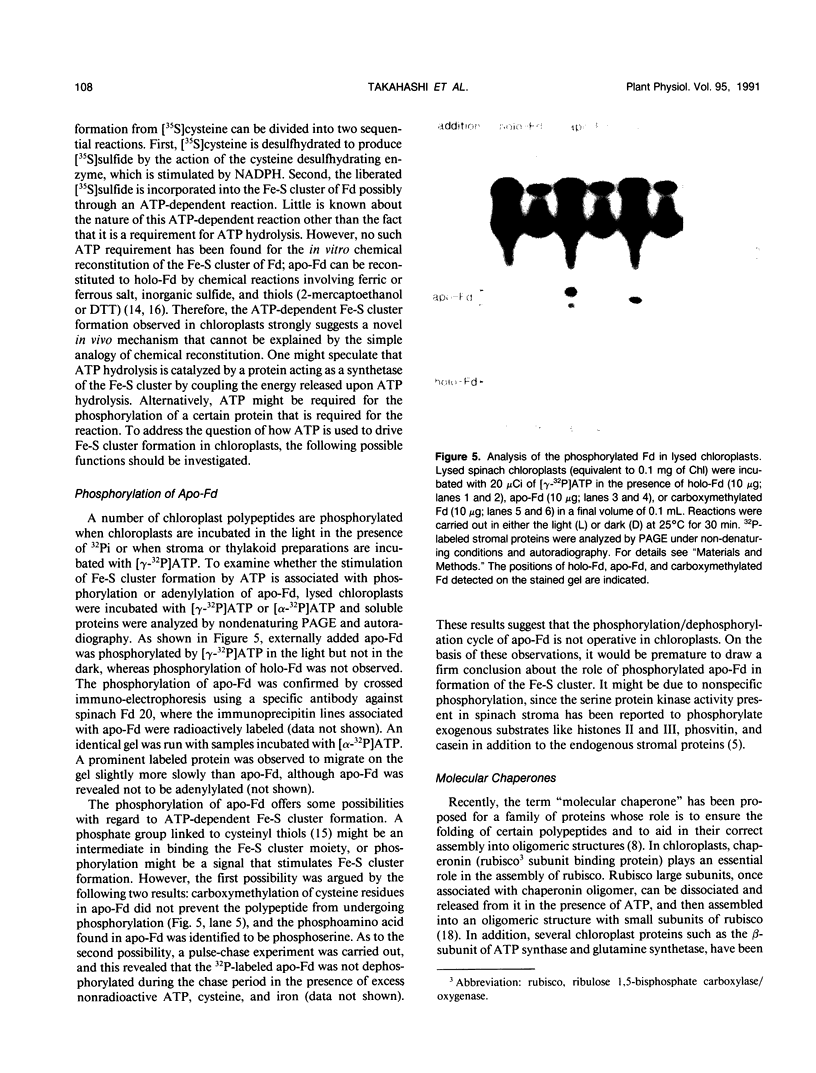
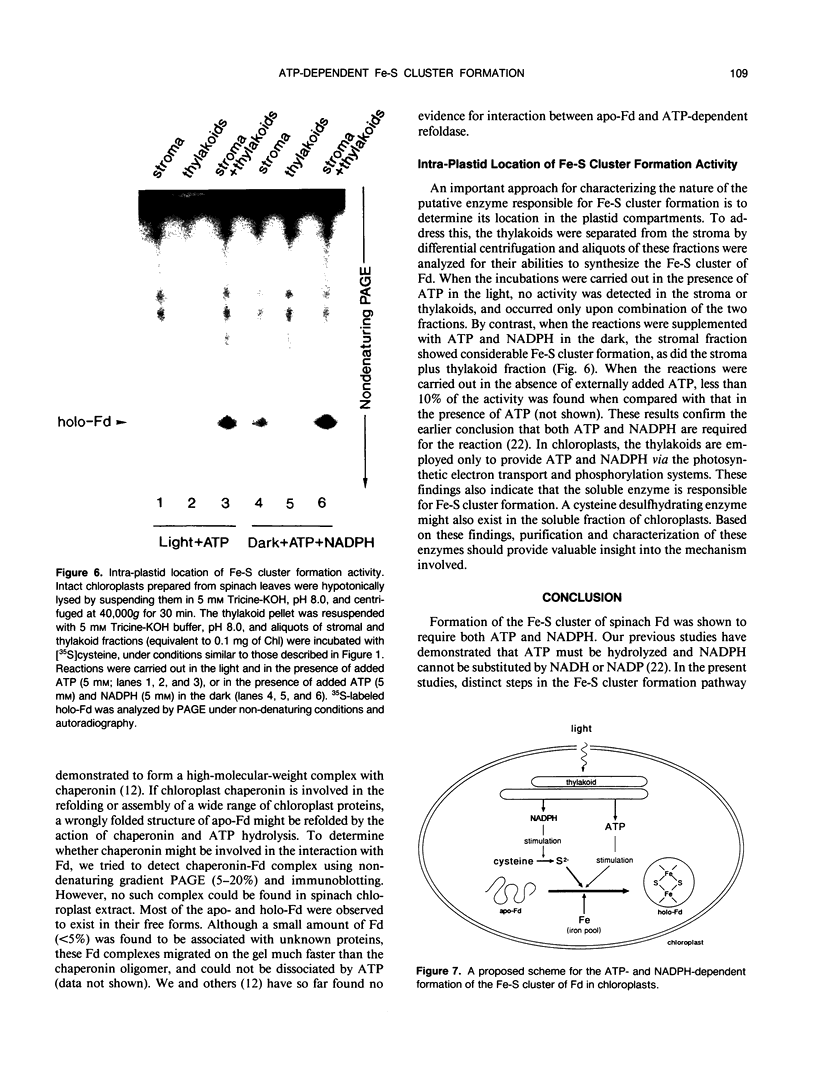
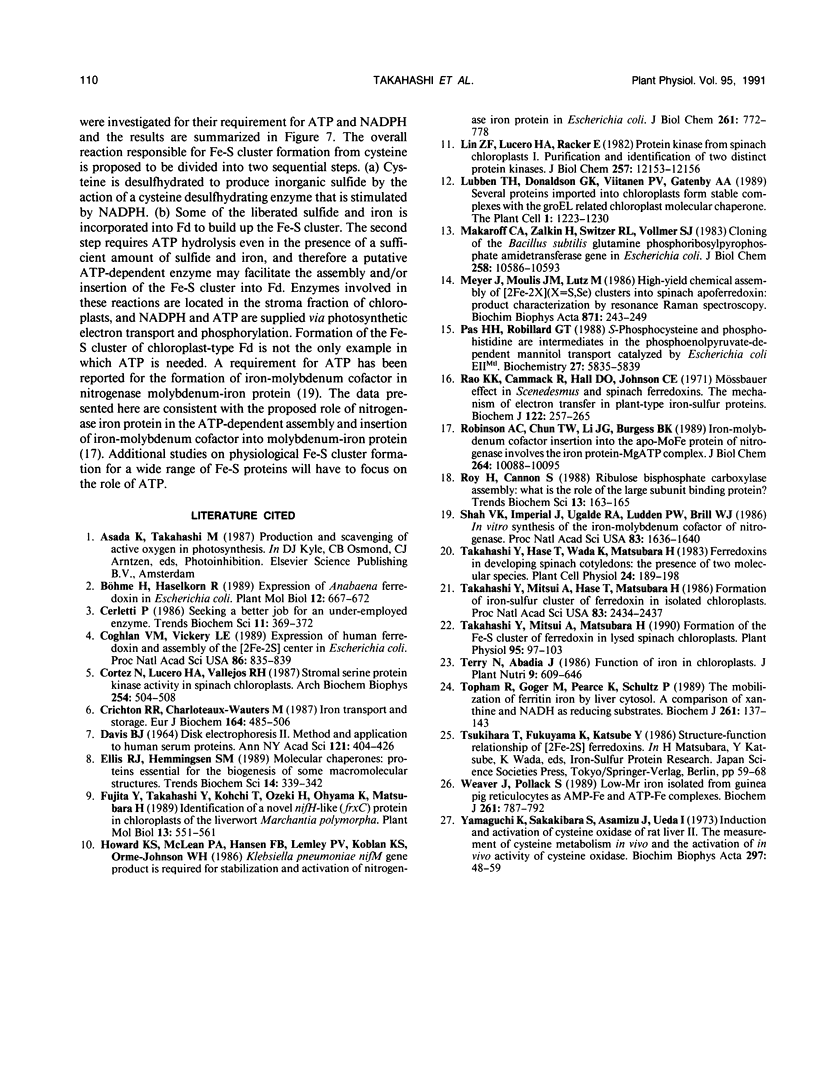
Images in this article
Selected References
These references are in PubMed. This may not be the complete list of references from this article.
- Coghlan V. M., Vickery L. E. Expression of human ferredoxin and assembly of the [2Fe-2S] center in Escherichia coli. Proc Natl Acad Sci U S A. 1989 Feb;86(3):835–839. doi: 10.1073/pnas.86.3.835. [DOI] [PMC free article] [PubMed] [Google Scholar]
- Cortez N., Lucero H. A., Vallejos R. H. Stromal serine protein kinase activity in spinach chloroplasts. Arch Biochem Biophys. 1987 May 1;254(2):504–508. doi: 10.1016/0003-9861(87)90130-5. [DOI] [PubMed] [Google Scholar]
- Crichton R. R., Charloteaux-Wauters M. Iron transport and storage. Eur J Biochem. 1987 May 4;164(3):485–506. doi: 10.1111/j.1432-1033.1987.tb11155.x. [DOI] [PubMed] [Google Scholar]
- DAVIS B. J. DISC ELECTROPHORESIS. II. METHOD AND APPLICATION TO HUMAN SERUM PROTEINS. Ann N Y Acad Sci. 1964 Dec 28;121:404–427. doi: 10.1111/j.1749-6632.1964.tb14213.x. [DOI] [PubMed] [Google Scholar]
- Ellis R. J., Hemmingsen S. M. Molecular chaperones: proteins essential for the biogenesis of some macromolecular structures. Trends Biochem Sci. 1989 Aug;14(8):339–342. doi: 10.1016/0968-0004(89)90168-0. [DOI] [PubMed] [Google Scholar]
- Fujita Y., Takahashi Y., Kohchi T., Ozeki H., Ohyama K., Matsubara H. Identification of a novel nifH-like (frxC) protein in chloroplasts of the liverwort Marchantia polymorpha. Plant Mol Biol. 1989 Nov;13(5):551–561. doi: 10.1007/BF00027315. [DOI] [PubMed] [Google Scholar]
- Howard K. S., McLean P. A., Hansen F. B., Lemley P. V., Koblan K. S., Orme-Johnson W. H. Klebsiella pneumoniae nifM gene product is required for stabilization and activation of nitrogenase iron protein in Escherichia coli. J Biol Chem. 1986 Jan 15;261(2):772–778. [PubMed] [Google Scholar]
- Lin Z. F., Lucero H. A., Racker E. Protein kinases from spinach chloroplasts. I. Purification and identification of two distinct protein kinases. J Biol Chem. 1982 Oct 25;257(20):12153–12156. [PubMed] [Google Scholar]
- Lubben T. H., Donaldson G. K., Viitanen P. V., Gatenby A. A. Several proteins imported into chloroplasts form stable complexes with the GroEL-related chloroplast molecular chaperone. Plant Cell. 1989 Dec;1(12):1223–1230. doi: 10.1105/tpc.1.12.1223. [DOI] [PMC free article] [PubMed] [Google Scholar]
- Makaroff C. A., Zalkin H., Switzer R. L., Vollmer S. J. Cloning of the Bacillus subtilis glutamine phosphoribosylpyrophosphate amidotransferase gene in Escherichia coli. Nucleotide sequence determination and properties of the plasmid-encoded enzyme. J Biol Chem. 1983 Sep 10;258(17):10586–10593. [PubMed] [Google Scholar]
- Pas H. H., Robillard G. T. S-phosphocysteine and phosphohistidine are intermediates in the phosphoenolpyruvate-dependent mannitol transport catalyzed by Escherichia coli EIIMtl. Biochemistry. 1988 Aug 9;27(16):5835–5839. doi: 10.1021/bi00416a002. [DOI] [PubMed] [Google Scholar]
- Rao K. K., Cammack R., Hall D. O., Johnson C. E. Mössbauer effect in Scenedesmus and spinach ferredoxins. The mechanism of electron transfer in plant-type iron-sulphur proteins. Biochem J. 1971 Apr;122(3):257–265. doi: 10.1042/bj1220257. [DOI] [PMC free article] [PubMed] [Google Scholar]
- Robinson A. C., Chun T. W., Li J. G., Burgess B. K. Iron-molybdenum cofactor insertion into the Apo-MoFe protein of nitrogenase involves the iron protein-MgATP complex. J Biol Chem. 1989 Jun 15;264(17):10088–10095. [PubMed] [Google Scholar]
- Roy H., Cannon S. Ribulose bisphosphate carboxylase assembly: what is the role of the large subunit binding protein? Trends Biochem Sci. 1988 May;13(5):163–165. doi: 10.1016/0968-0004(88)90139-9. [DOI] [PubMed] [Google Scholar]
- Shah V. K., Imperial J., Ugalde R. A., Ludden P. W., Brill W. J. In vitro synthesis of the iron-molybdenum cofactor of nitrogenase. Proc Natl Acad Sci U S A. 1986 Mar;83(6):1636–1640. doi: 10.1073/pnas.83.6.1636. [DOI] [PMC free article] [PubMed] [Google Scholar]
- Takahashi Y., Mitsui A., Hase T., Matsubara H. Formation of the iron-sulfur cluster of ferredoxin in isolated chloroplasts. Proc Natl Acad Sci U S A. 1986 Apr;83(8):2434–2437. doi: 10.1073/pnas.83.8.2434. [DOI] [PMC free article] [PubMed] [Google Scholar]
- Takahashi Y., Mitsui A., Matsubara H. Formation of the fe-s cluster of ferredoxin in lysed spinach chloroplasts. Plant Physiol. 1991 Jan;95(1):97–103. doi: 10.1104/pp.95.1.97. [DOI] [PMC free article] [PubMed] [Google Scholar]
- Topham R., Goger M., Pearce K., Schultz P. The mobilization of ferritin iron by liver cytosol. A comparison of xanthine and NADH as reducing substrates. Biochem J. 1989 Jul 1;261(1):137–143. doi: 10.1042/bj2610137. [DOI] [PMC free article] [PubMed] [Google Scholar]
- Weaver J., Pollack S. Low-Mr iron isolated from guinea pig reticulocytes as AMP-Fe and ATP-Fe complexes. Biochem J. 1989 Aug 1;261(3):787–792. doi: 10.1042/bj2610787. [DOI] [PMC free article] [PubMed] [Google Scholar]
- Yamaguchi K., Sakakibara S., Asamizu J., Ueda I. Induction and activation of cysteine oxidase of rat liver. II. The measurement of cysteine metabolism in vivo and the activation of in vivo activity of cysteine oxidase. Biochim Biophys Acta. 1973 Jan 24;297(1):48–59. doi: 10.1016/0304-4165(73)90048-2. [DOI] [PubMed] [Google Scholar]