Abstract
Separation of the wound exudate from Acacia senegal (L.) Willd., “gum arabic,” on a preparative Superose-6 column gave two major fractions: a high molecular weight gum arabic glycoprotein (GAGP) containing about 90% carbohydrate and a lower molecular weight heterogenous gum arabic polysaccharide fraction. Hydrogen fluoride-deglycosylation of GAGP gave a large (∼400 residue) hydroxyproline-rich polypeptide backbone (dGAGP). Alkaline hydrolysis of GAGP showed that most of the carbohydrate was attached to the polypeptide backbone as small (∼30 residue) hydroxyproline (Hyp)-polysaccharide substituents. After partial acid hydrolysis of the Hyp-polysaccharide fraction we identified O-galactosylhydroxyproline as the glycopeptide linkage, identical with that of hydroxyproline-rich arabinogalactan-proteins (AGPs). However, unlike the acidic alanine-rich AGPs, GAGP is basic and notably deficient in alanine. Thus, while the GAGP polypeptide backbone more closely resembles that of the Hyp-rich cell wall protein extensin, the GAGP polysaccharide sidechains resemble AGPs. Possibly all three proteins comprise a phylogenetically related extensin superfamily of extended rod-like macromolecules. The “wattle-blossom” model for AGP and gum arabic predicts a few large polysaccharide substituents along the polypeptide backbone of a spheroidal macromolecule. On the contrary, our data imply a rodlike molecule with numerous small polysaccharide substituents (attached to 24% of the Hyp residues), regularly arranged along a highly periodic polypeptide backbone based, hypothetically, on a 10 to 12 residue repetitive peptide motif. Thus, a simple statistical model of the gum arabic glycoprotein predicts a repeating polysaccharide-peptide subunit of about 7 kilodaltons. The small polysaccharide substituents will maximize intramolecular hydrogen bonding if aligned along the long axis of the molecule, forming in effect a twisted hairy rope. Electron micrographs of rotary shadowed GAGP molecules support that prediction and may also explain how such apparently large molecules can exit the cell by endwise reptation through the small pores of the primary cell wall.
Full text
PDF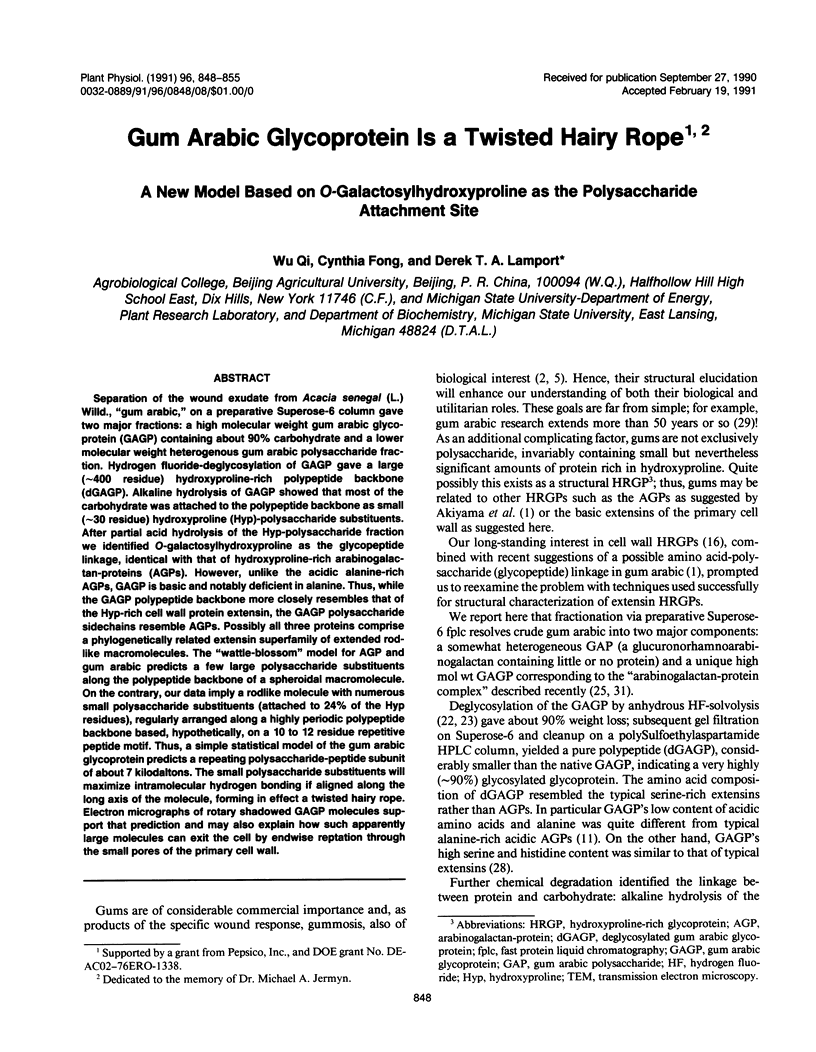
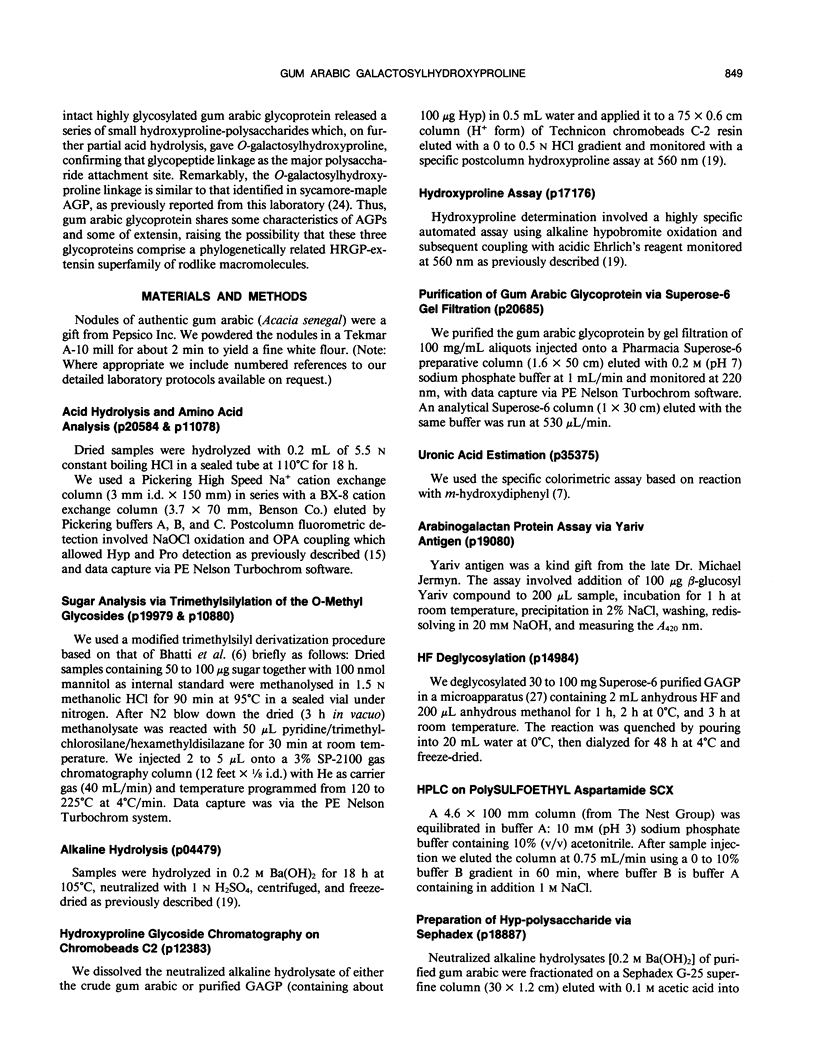
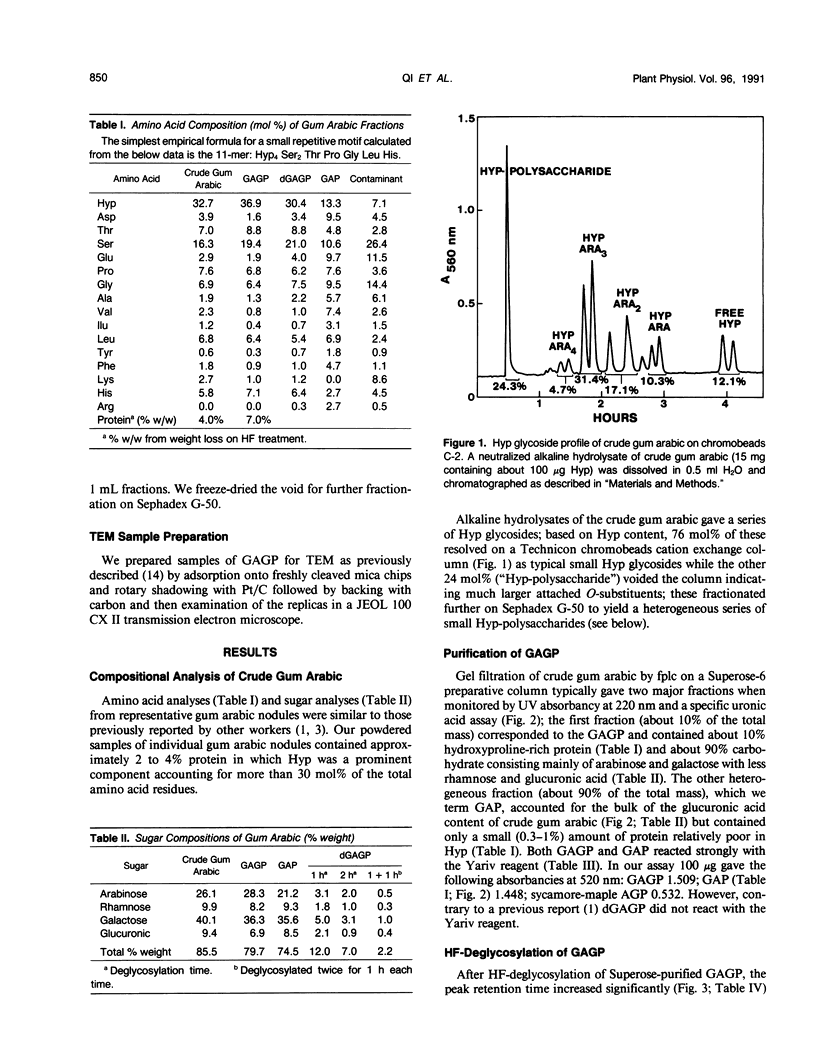
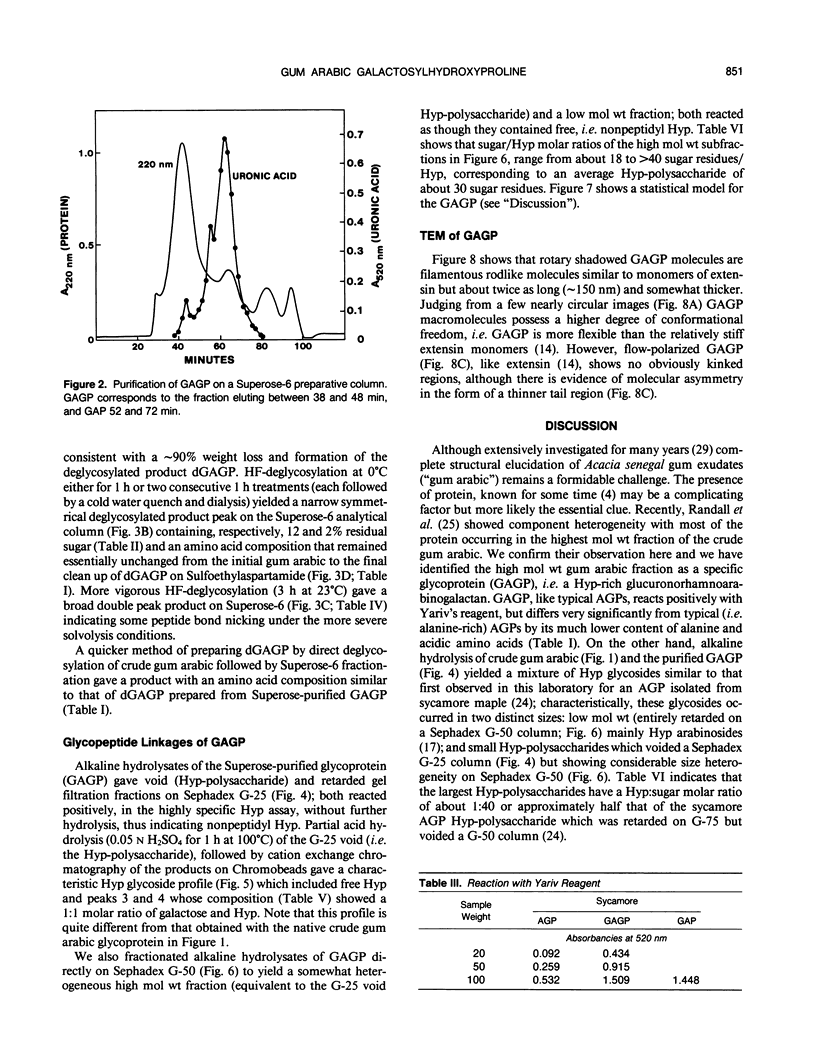
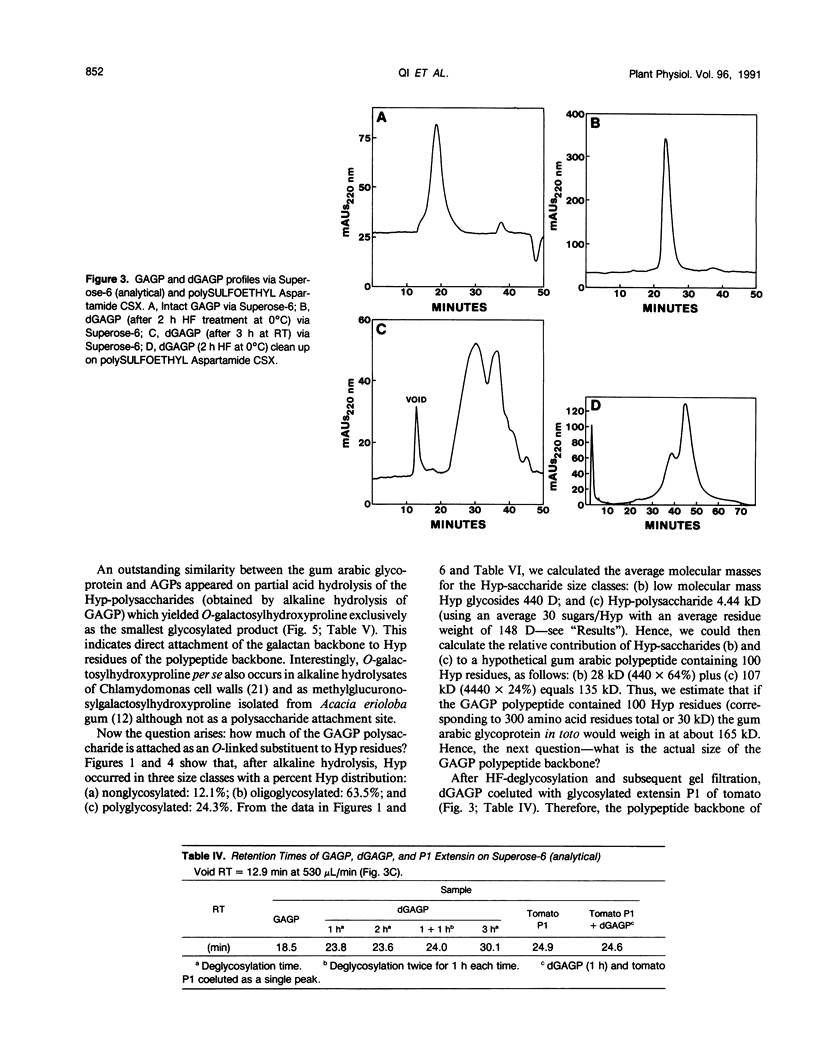
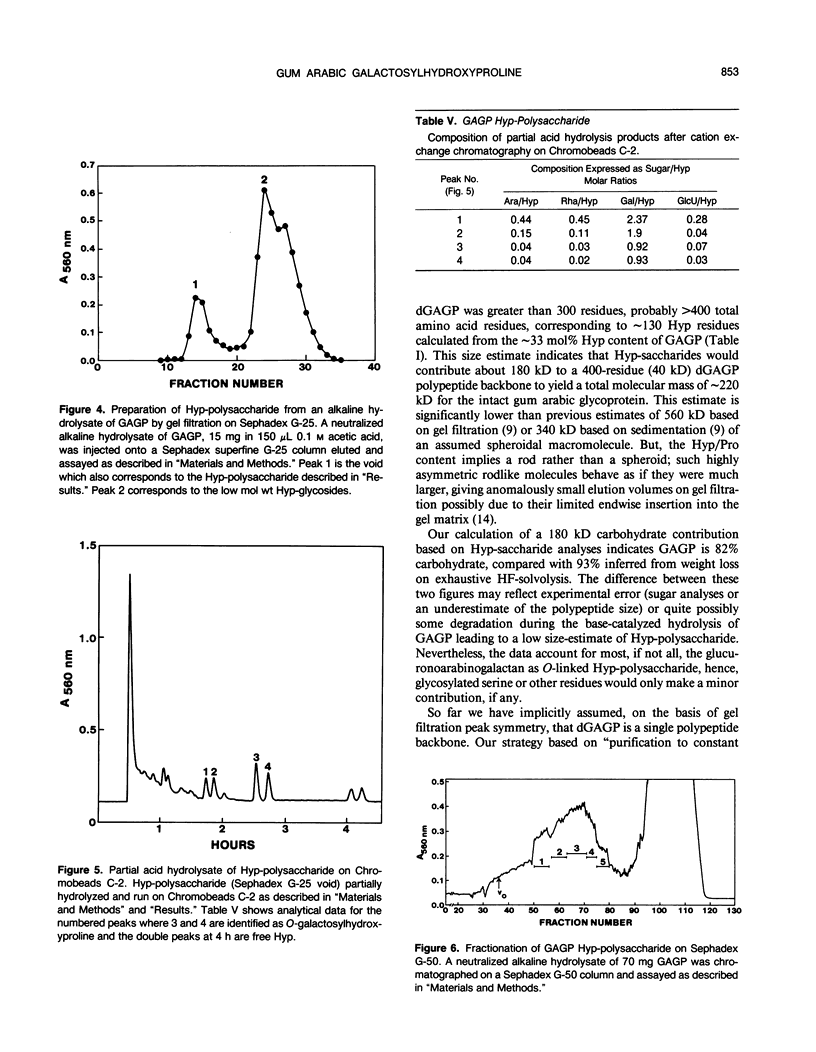
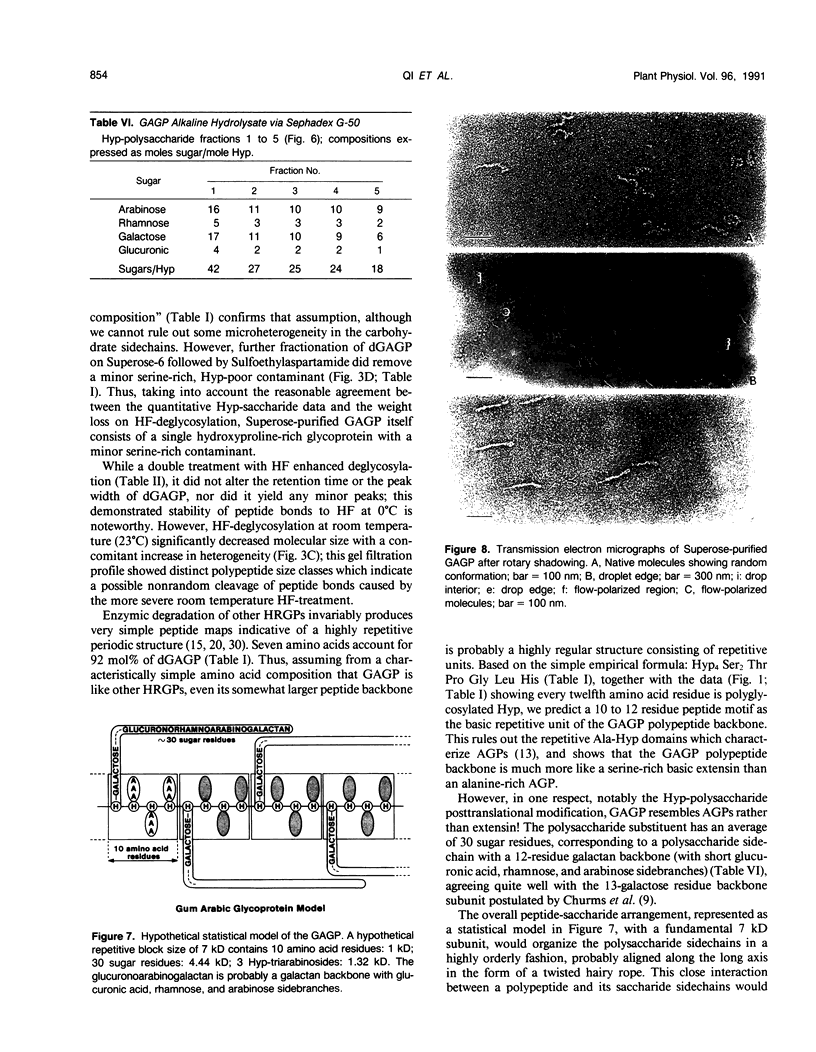
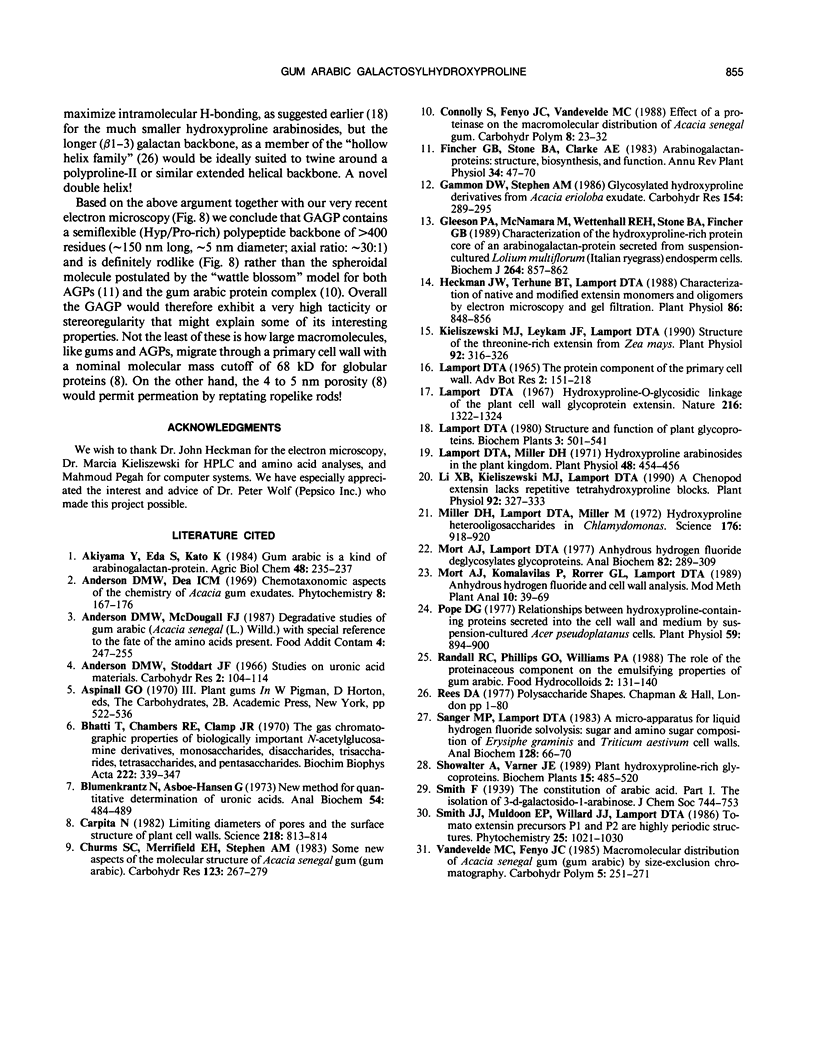
Images in this article
Selected References
These references are in PubMed. This may not be the complete list of references from this article.
- Bhatti T., Chambers R. E., Clamp J. R. The gas chromatographic properties of biologically important N-acetylglucosamine derivatives, monosaccharides, disaccharides, trisaccharides, tetrasaccharides and pentasaccharides. Biochim Biophys Acta. 1970 Nov 24;222(2):339–347. doi: 10.1016/0304-4165(70)90122-4. [DOI] [PubMed] [Google Scholar]
- Blumenkrantz N., Asboe-Hansen G. New method for quantitative determination of uronic acids. Anal Biochem. 1973 Aug;54(2):484–489. doi: 10.1016/0003-2697(73)90377-1. [DOI] [PubMed] [Google Scholar]
- Carpita N. C. Limiting diameters of pores and the surface structure of plant cell walls. Science. 1982 Nov 19;218(4574):813–814. doi: 10.1126/science.218.4574.813. [DOI] [PubMed] [Google Scholar]
- Gleeson P. A., McNamara M., Wettenhall R. E., Stone B. A., Fincher G. B. Characterization of the hydroxyproline-rich protein core of an arabinogalactan-protein secreted from suspension-cultured Lolium multiflorum (Italian ryegrass) endosperm cells. Biochem J. 1989 Dec 15;264(3):857–862. doi: 10.1042/bj2640857. [DOI] [PMC free article] [PubMed] [Google Scholar]
- Heckman J. W., Terhune B. T., Lamport D. T. Characterization of native and modified extensin monomers and oligomers by electron microscopy and gel filtration. Plant Physiol. 1988 Mar;86(3):848–856. doi: 10.1104/pp.86.3.848. [DOI] [PMC free article] [PubMed] [Google Scholar]
- Kieliszewski M. J., Leykam J. F., Lamport D. T. Structure of the Threonine-Rich Extensin from Zea mays. Plant Physiol. 1990 Feb;92(2):316–326. doi: 10.1104/pp.92.2.316. [DOI] [PMC free article] [PubMed] [Google Scholar]
- Lamport D. T., Miller D. H. Hydroxyproline arabinosides in the plant kingdom. Plant Physiol. 1971 Oct;48(4):454–456. doi: 10.1104/pp.48.4.454. [DOI] [PMC free article] [PubMed] [Google Scholar]
- Li X. B., Kieliszewski M., Lamport D. T. A chenopod extensin lacks repetitive tetrahydroxyproline blocks. Plant Physiol. 1990 Feb;92(2):327–333. doi: 10.1104/pp.92.2.327. [DOI] [PMC free article] [PubMed] [Google Scholar]
- Miller D. H., Lamport D. T., Miller M. Hydroxyproline heterooligosaccharides in Chlamydomonas. Science. 1972 May 26;176(4037):918–920. doi: 10.1126/science.176.4037.918. [DOI] [PubMed] [Google Scholar]
- Mort A. J., Lamport D. T. Anhydrous hydrogen fluoride deglycosylates glycoproteins. Anal Biochem. 1977 Oct;82(2):289–309. doi: 10.1016/0003-2697(77)90165-8. [DOI] [PubMed] [Google Scholar]
- Pope D. G. Relationships between Hydroxyproline-containing Proteins Secreted into the Cell Wall and Medium by Suspension-cultured Acer pseudoplatanus Cells. Plant Physiol. 1977 May;59(5):894–900. doi: 10.1104/pp.59.5.894. [DOI] [PMC free article] [PubMed] [Google Scholar]
- Sanger M. P., Lamport D. T. A microapparatus for liquid hydrogen fluoride solvolysis: sugar and amino sugar composition of Erysiphe graminis and Triticum aestivum cell walls. Anal Biochem. 1983 Jan;128(1):66–70. doi: 10.1016/0003-2697(83)90345-7. [DOI] [PubMed] [Google Scholar]