Abstract
Uptake of the lipophilic cation triphenylmethylphosphonium into mesophyll protoplasts of oat (Avena sativa L. cv. “Garry”) approaches equilibrium at 3 to 4 hours. The resulting external and internal concentrations are then used with the Nernst equation to obtain a membrane potential of −62 millivolts, inside negative. Potentials calculated in this manner are depolarized by adding 2 mm sodium azide and 50 μm carbonyl cyanide m-chlorophenylhydrazone as well as by increasing the external proton and potassium concentrations. The depolarizations are qualitatively similar to those seen when oat mesoyphll cells are measured in situ with microelectrodes. It is concluded that due to the lack of turgor and fragility of protoplasts, estimations of their membrane potential may be made more reliably, under some conditions, with lipophilic cations than with microelectrodes.
Full text
PDF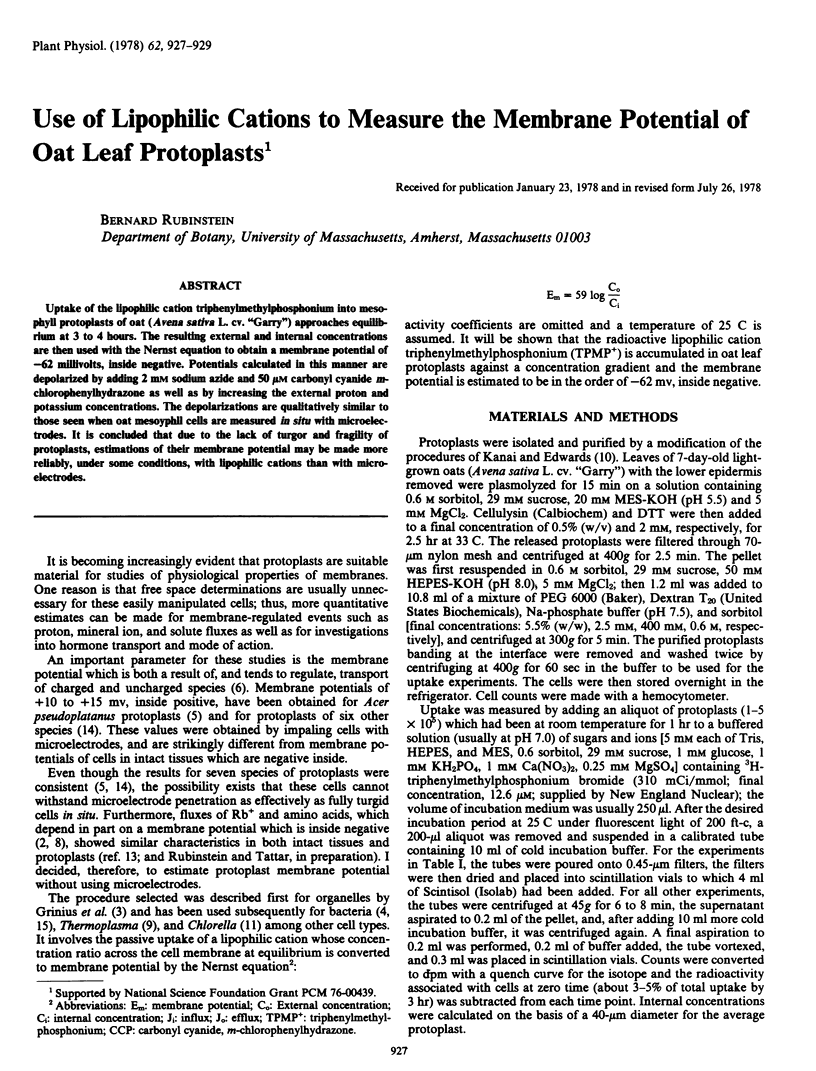
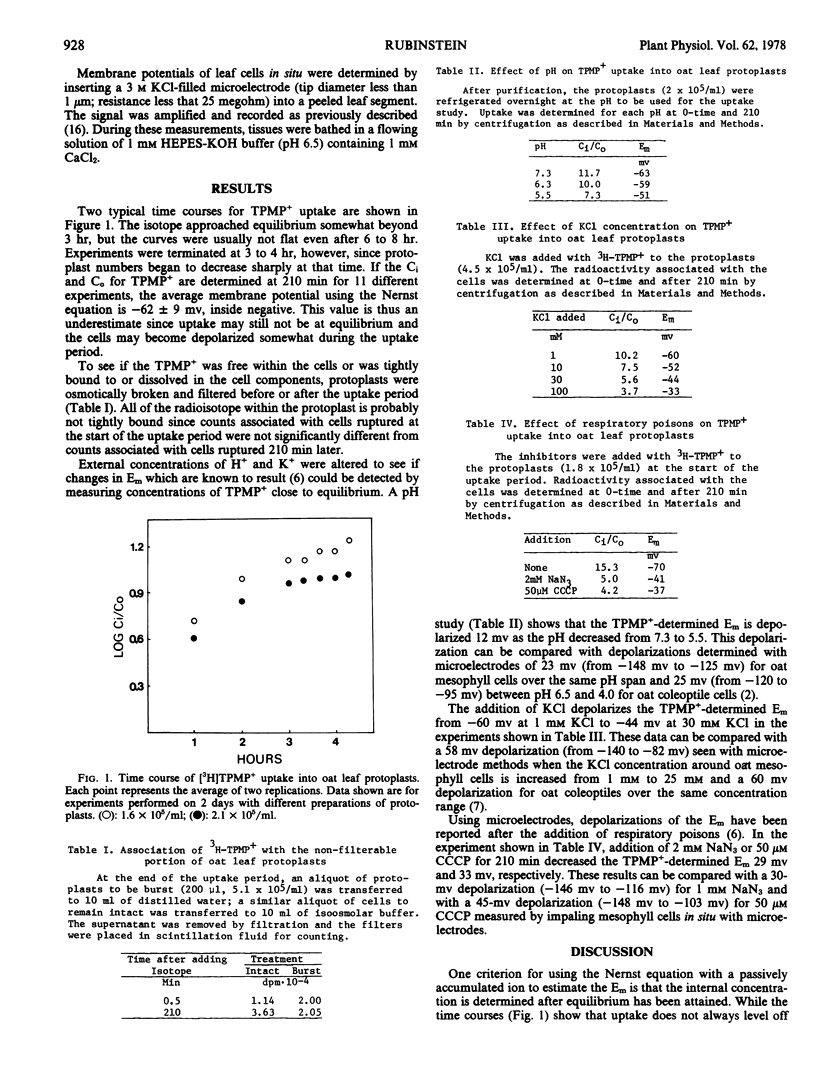
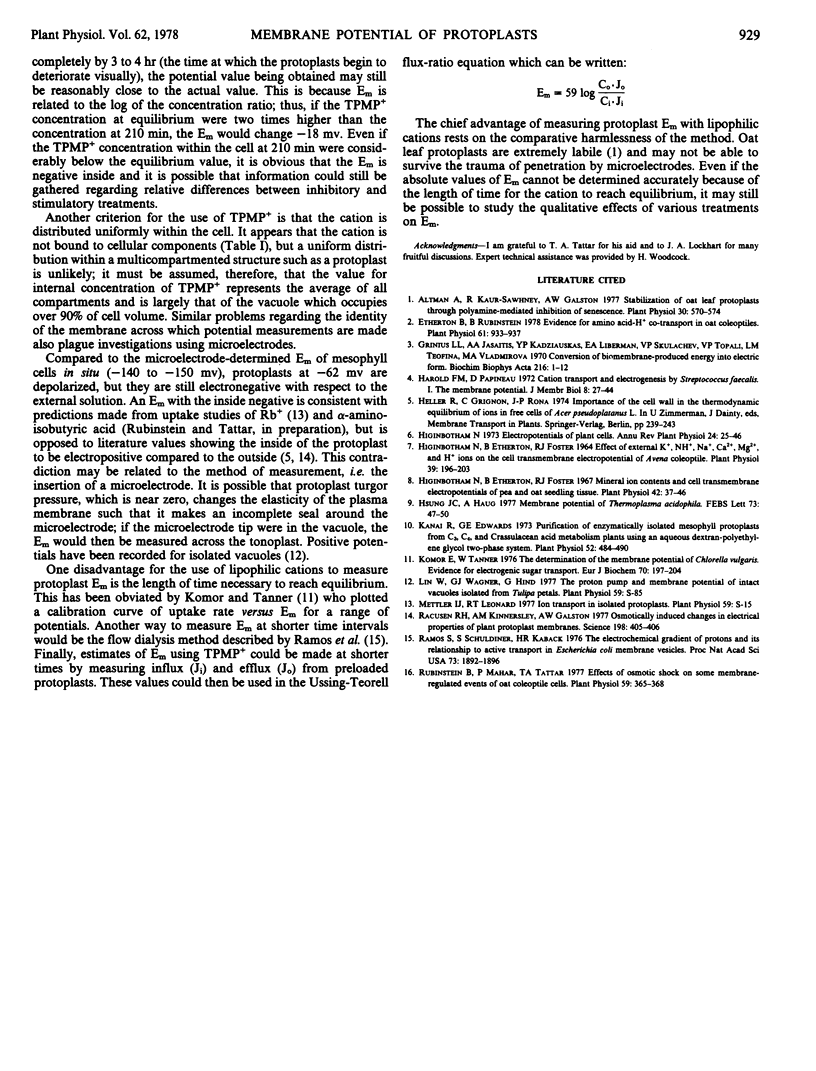
Selected References
These references are in PubMed. This may not be the complete list of references from this article.
- Altman A., Kaur-Sawhney R., Galston A. W. Stabilization of Oat Leaf Protoplasts through Polyamine-mediated Inhibition of Senescence. Plant Physiol. 1977 Oct;60(4):570–574. doi: 10.1104/pp.60.4.570. [DOI] [PMC free article] [PubMed] [Google Scholar]
- Etherton B. Evidence for amino Acid-h co-transport in oat coleoptiles. Plant Physiol. 1978 Jun;61(6):933–937. doi: 10.1104/pp.61.6.933. [DOI] [PMC free article] [PubMed] [Google Scholar]
- Grinius L. L., Jasaitis A. A., Kadziauskas Y. P., Liberman E. A., Skulachev V. P., Topali V. P., Tsofina L. M., Vladimirova M. A. Conversion of biomembrane-produced energy into electric form. I. Submitochondrial particles. Biochim Biophys Acta. 1970 Aug 4;216(1):1–12. doi: 10.1016/0005-2728(70)90153-2. [DOI] [PubMed] [Google Scholar]
- Harold F. M., Papineau D. Cation transport and electrogenesis by Streptococcus faecalis. I. The membrane potential. J Membr Biol. 1972;8(1):27–44. doi: 10.1007/BF01868093. [DOI] [PubMed] [Google Scholar]
- Higinbotham N., Etherton B., Foster R. J. Effect of External K, NH(4), Na, Ca, Mg, and H Ions on the Cell Transmembrane Electropotential of Avena Coleoptile. Plant Physiol. 1964 Mar;39(2):196–203. doi: 10.1104/pp.39.2.196. [DOI] [PMC free article] [PubMed] [Google Scholar]
- Hsung J. C., Haug A. Membrane potential of Thermoplasma acidophila. FEBS Lett. 1977 Jan 15;73(1):47–50. doi: 10.1016/0014-5793(77)80011-2. [DOI] [PubMed] [Google Scholar]
- Kanai R., Edwards G. E. Purification of enzymatically isolated mesophyll protoplasts from c(3), c(4), and crassulacean Acid metabolism plants using an aqueous dextran-polyethylene glycol two-phase system. Plant Physiol. 1973 Nov;52(5):484–490. doi: 10.1104/pp.52.5.484. [DOI] [PMC free article] [PubMed] [Google Scholar]
- Komor E., Tanner W. The determination of the membrane ptoential of Chlorella vulgaris. Evidence for electrogenic sugar transport. Eur J Biochem. 1976 Nov 1;70(1):197–204. doi: 10.1111/j.1432-1033.1976.tb10970.x. [DOI] [PubMed] [Google Scholar]
- Racusen R. H., Kinnersley A. M., Galston A. W. Osmotically induced changes in electrical properties of plant protoplast membranes. Science. 1977 Oct 28;198(4315):405–407. doi: 10.1126/science.198.4315.405. [DOI] [PubMed] [Google Scholar]
- Ramos S., Schuldiner S., Kaback H. R. The electrochemical gradient of protons and its relationship to active transport in Escherichia coli membrane vesicles. Proc Natl Acad Sci U S A. 1976 Jun;73(6):1892–1896. doi: 10.1073/pnas.73.6.1892. [DOI] [PMC free article] [PubMed] [Google Scholar]