Abstract
1. Values of the kinetic specificity constant, kcat./Km, for the hydrolysis of N-acetyl-L-phenylalanylglycine 4-nitroanilide (I) and of its D-enantiomer (II) catalysed by ficin (EC 3.4.22.3) and by actinidin (EC 3.4.22.14) at pH 6.0, I 0.1 mol/l, 8.3% (v/v) NN-dimethylformamide and 25 degrees C were determined by using initial-rate data with [S] much less than Km and weighted nonlinear regression analysis as: for ficin, (kcat./Km)L = 271 +/- 6 M-1.s-1, (kcat./Km)D = 2.9 +/- 0.1 M-1.s-1, and for actinidin (kcat./Km)L = 13.3 +/- 0.7 M-1.s-1, (kcat/Km)D = 0.34 +/- 0.01 M-1.s-1.2. These data and analogous values for the corresponding reactions catalysed by papain (EC 3.4.22.2), (kcat./Km)L = 2064 +/- 31 M-1.s-1, (kcat./Km)D = 5.5 +/- 0.1 M-1.s-1, demonstrate marked variation in stereochemical selectivity for substrates (I) and (II) among the three cysteine proteinases with the following values for the index of stereochemical selectivity Iss = (kcat./Km)L/(kcat./Km)D: for papain, 375; for ficin 93; for actinidin 39. 3. Model building suggests ways in which, for the papain-catalysed reactions, binding interactions involving the extended acyl groups of the substrates may need to change as the reaction proceeds from adsorptive complex (ES) to tetrahedral intermediate (THI) before its rate-determining, general acid-catalysed collapse to acylenzyme intermediate. In particular, satisfactory alignment in the catalytic site at the THI stage of the acylation process appears to demand rotation of the substrate moiety about its long axis. 4. The different consequences of this rotation for the L- and D-enantiomers suggest that for closely related systems the greater the extent of this rotational adjustment the greater would be the value of Iss.5. For the actinidin-substrate combinations, model building suggests that even at the ES complex stage of catalysis it is not possible to approach optimized P2-S2 contacts and the three hydrogen-bonding interactions deduced for papain-ligand complexes in the absence of significant movement of protein conformation. Possible binding modes in which some of the interactions deduced for papain are relaxed are discussed. Consideration of postulated binding modes in the various transition states is shown to account for the order of reactivity reflected in values kcat./Km for the four reactions involving papain (Pap) and actinidin (Act) with the L- and D-enantiomeric substrates: Pap-L much greater than Act-L greater than Pap-D much greater than Act-D.(ABSTRACT TRUNCATED AT 400 WORDS)
Full text
PDF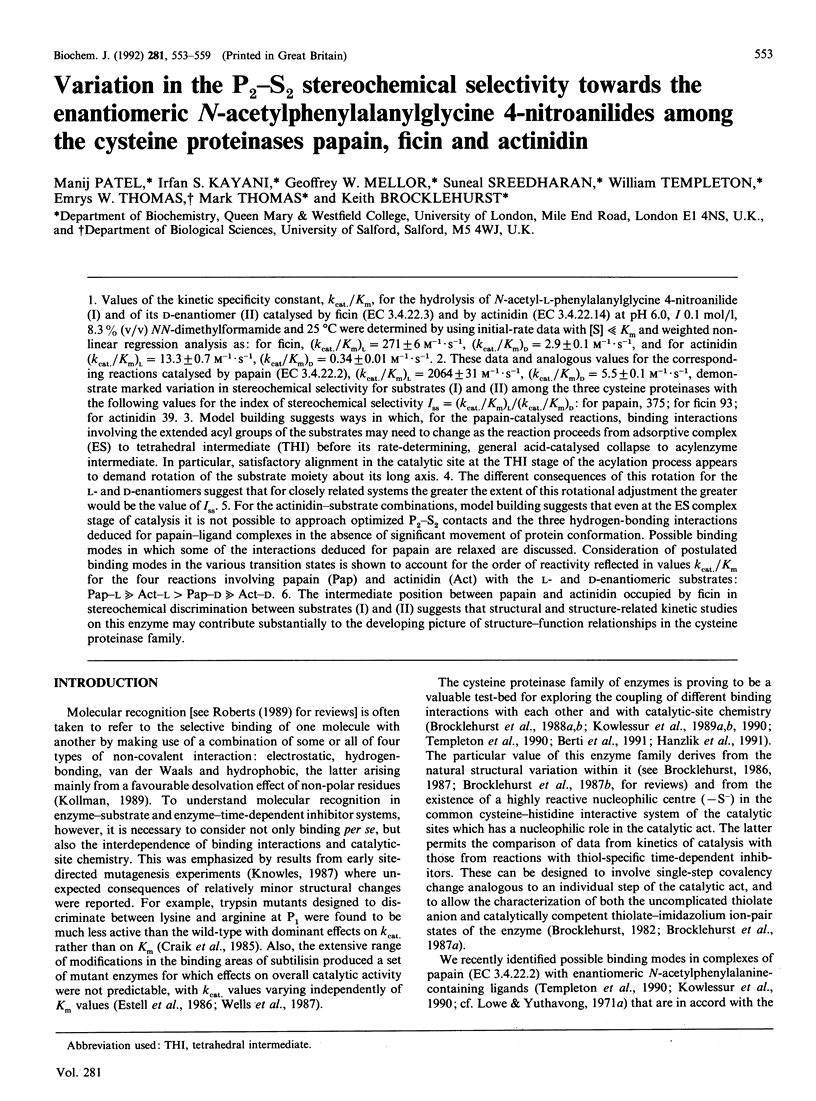
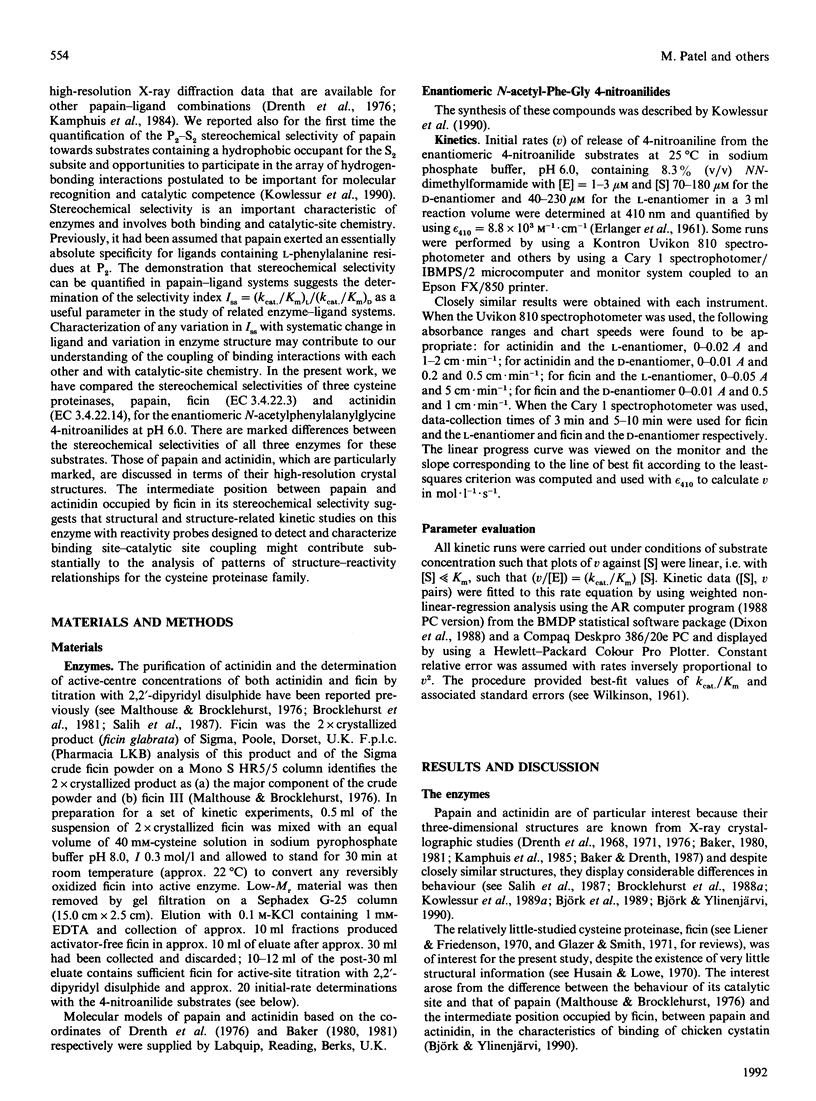
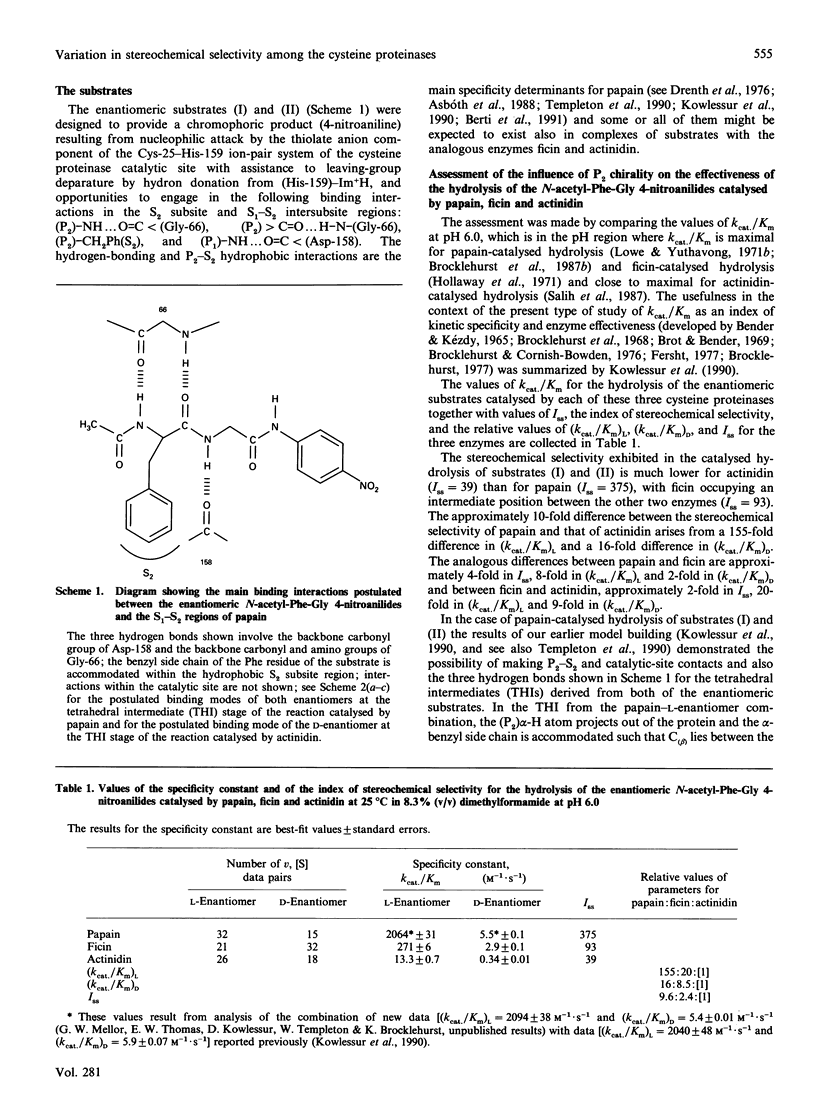
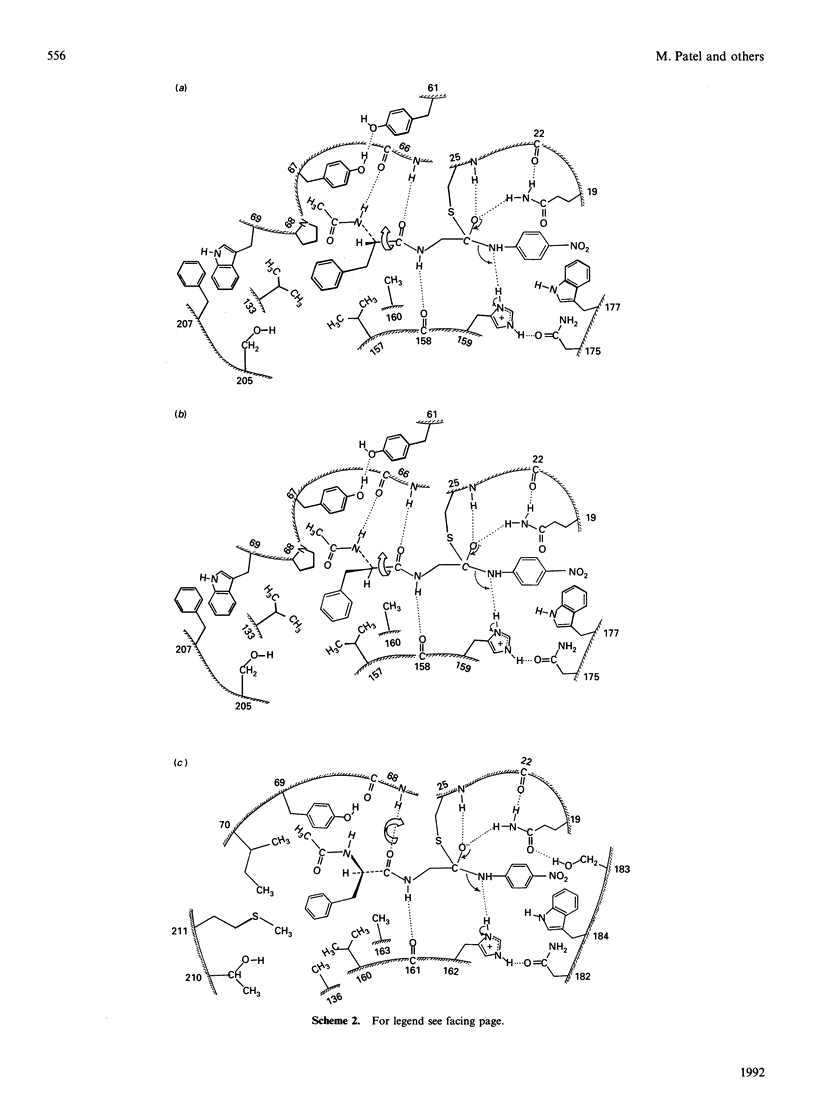
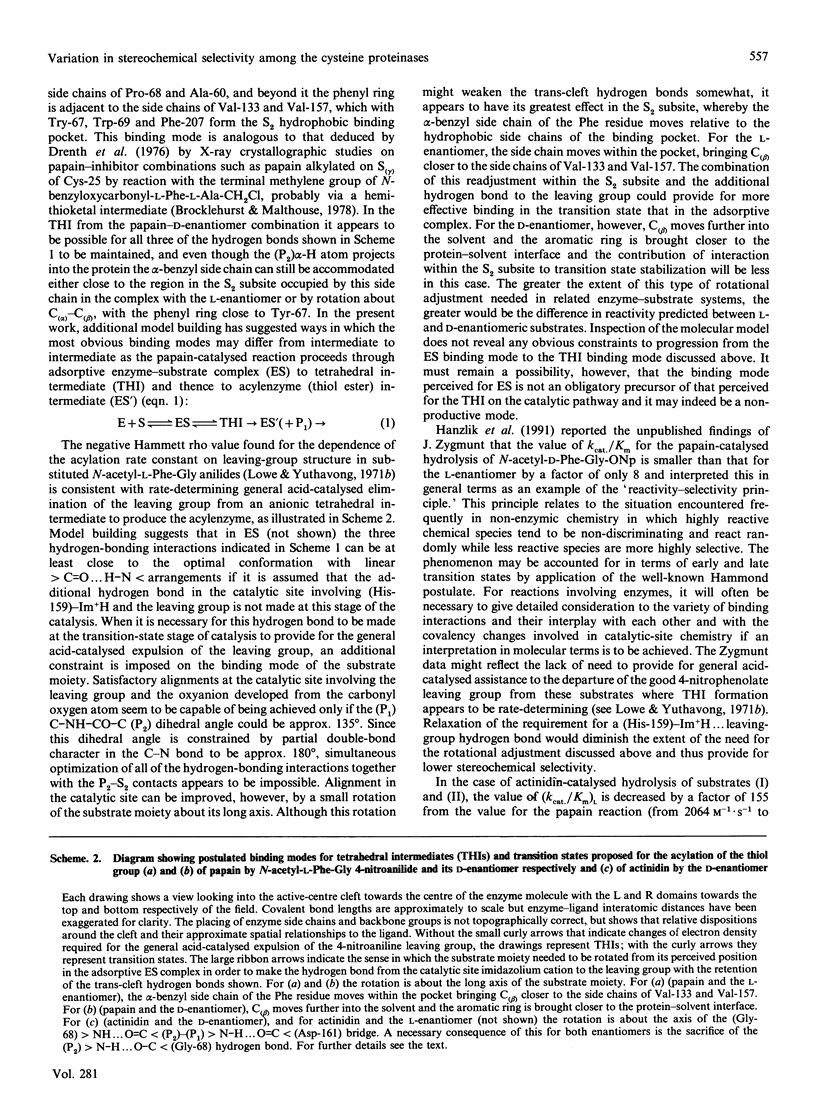
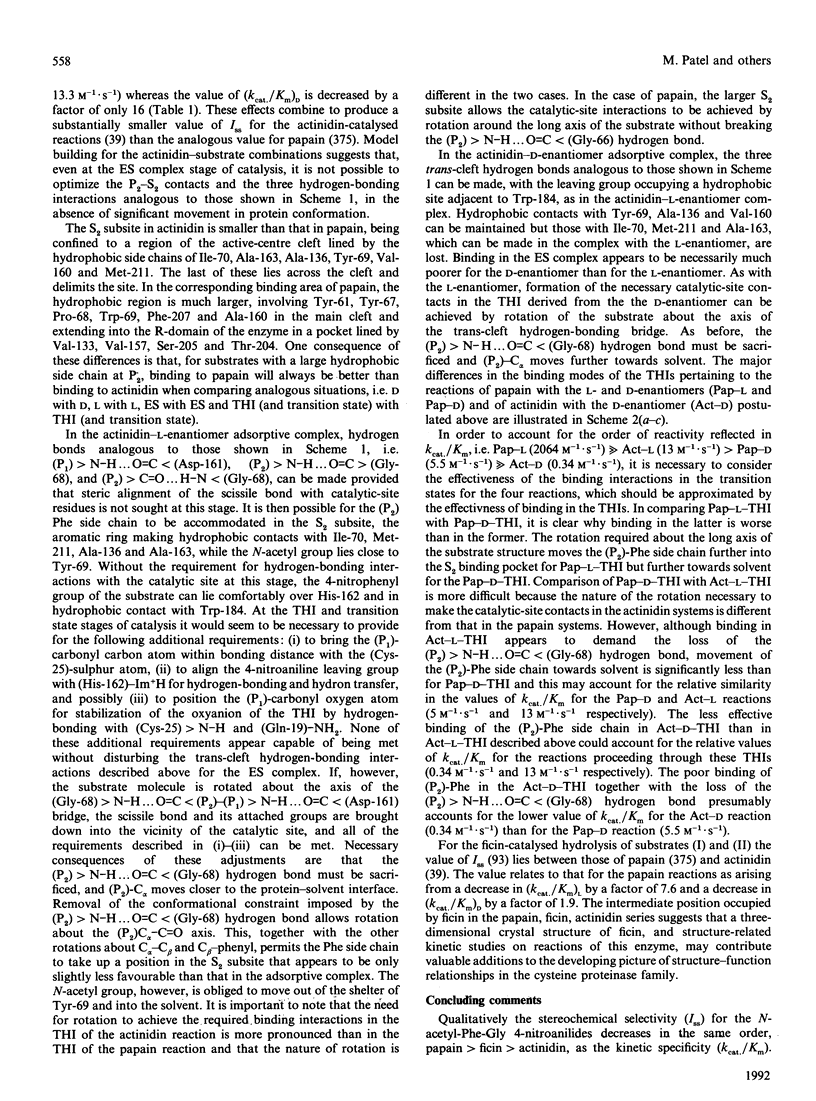
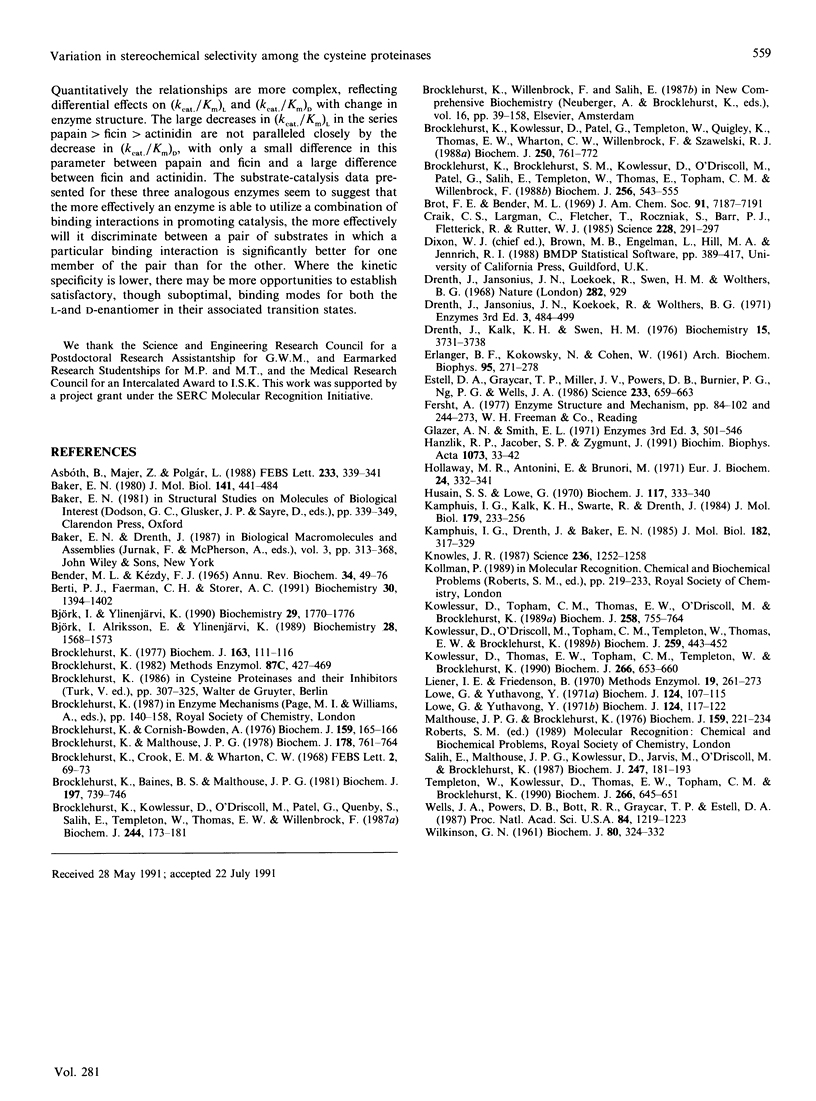
Selected References
These references are in PubMed. This may not be the complete list of references from this article.
- Asbóth B., Majer Z., Polgár L. Cysteine proteases: the S2P2 hydrogen bond is more important for catalysis than is the analogous S1P1 bond. FEBS Lett. 1988 Jun 20;233(2):339–341. doi: 10.1016/0014-5793(88)80455-1. [DOI] [PubMed] [Google Scholar]
- BENDER M. L., KEZDY J. MECHANISM OF ACTION OF PROTEOLYTIC ENZYMES. Annu Rev Biochem. 1965;34:49–76. doi: 10.1146/annurev.bi.34.070165.000405. [DOI] [PubMed] [Google Scholar]
- Baker E. N. Structure of actinidin, after refinement at 1.7 A resolution. J Mol Biol. 1980 Aug 25;141(4):441–484. doi: 10.1016/0022-2836(80)90255-7. [DOI] [PubMed] [Google Scholar]
- Baker E. N. Structure of actinidin, after refinement at 1.7 A resolution. J Mol Biol. 1980 Aug 25;141(4):441–484. doi: 10.1016/0022-2836(80)90255-7. [DOI] [PubMed] [Google Scholar]
- Berti P. J., Faerman C. H., Storer A. C. Cooperativity of papain-substrate interaction energies in the S2 to S2' subsites. Biochemistry. 1991 Feb 5;30(5):1394–1402. doi: 10.1021/bi00219a033. [DOI] [PubMed] [Google Scholar]
- Björk I., Alriksson E., Ylinenjärvi K. Kinetics of binding of chicken cystatin to papain. Biochemistry. 1989 Feb 21;28(4):1568–1573. doi: 10.1021/bi00430a022. [DOI] [PubMed] [Google Scholar]
- Björk I., Ylinenjärvi K. Interaction between chicken cystatin and the cysteine proteinases actinidin, chymopapain A, and ficin. Biochemistry. 1990 Feb 20;29(7):1770–1776. doi: 10.1021/bi00459a016. [DOI] [PubMed] [Google Scholar]
- Brocklehurst K., Baines B. S., Malthouse J. P. Differences in the interaction of the catalytic groups of the active centres of actinidin and papain. Rapid purification of fully active actinidin by covalent chromatography and characterization of its active centre by use of two-protonic-state reactivity probes. Biochem J. 1981 Sep 1;197(3):739–746. doi: 10.1042/bj1970739. [DOI] [PMC free article] [PubMed] [Google Scholar]
- Brocklehurst K., Brocklehurst S. M., Kowlessur D., O'Driscoll M., Patel G., Salih E., Templeton W., Thomas E., Topham C. M., Willenbrock F. Supracrystallographic resolution of interactions contributing to enzyme catalysis by use of natural structural variants and reactivity-probe kinetics. Biochem J. 1988 Dec 1;256(2):543–558. doi: 10.1042/bj2560543. [DOI] [PMC free article] [PubMed] [Google Scholar]
- Brocklehurst K., Cornish-Bowden A. The pre-eminence of k(cat) in the manifestation of optimal enzymic activity delineated by using the Briggs-Haldane two-step irreversible kinetic model. Biochem J. 1976 Oct 1;159(1):165–166. doi: 10.1042/bj1590165. [DOI] [PMC free article] [PubMed] [Google Scholar]
- Brocklehurst K., Crook E. M., Wharton C. W. The kinetic analysis of hydrolytic enzyme catalyses: Consequences of non-productive binding. FEBS Lett. 1968 Nov;2(1):69–73. doi: 10.1016/0014-5793(68)80103-6. [DOI] [PubMed] [Google Scholar]
- Brocklehurst K. Evolution of enzyme catalytic power. Characteristics of optimal catalysis evaluated for the simplest plausible kinetic model. Biochem J. 1977 Apr 1;163(1):111–116. doi: 10.1042/bj1630111. [DOI] [PMC free article] [PubMed] [Google Scholar]
- Brocklehurst K., Kowlessur D., O'Driscoll M., Patel G., Quenby S., Salih E., Templeton W., Thomas E. W., Willenbrock F. Substrate-derived two-protonic-state electrophiles as sensitive kinetic specificity probes for cysteine proteinases. Activation of 2-pyridyl disulphides by hydrogen-bonding. Biochem J. 1987 May 15;244(1):173–181. doi: 10.1042/bj2440173. [DOI] [PMC free article] [PubMed] [Google Scholar]
- Brocklehurst K., Kowlessur D., Patel G., Templeton W., Quigley K., Thomas E. W., Wharton C. W., Willenbrock F., Szawelski R. J. Consequences of molecular recognition in the S1-S2 intersubsite region of papain for catalytic-site chemistry. Change in pH-dependence characteristics and generation of an inverse solvent kinetic isotope effect by introduction of a P1-P2 amide bond into a two-protonic-state reactivity probe. Biochem J. 1988 Mar 15;250(3):761–772. doi: 10.1042/bj2500761. [DOI] [PMC free article] [PubMed] [Google Scholar]
- Brocklehurst K., Malthouse J. P. Mechanism of the reaction of papain with substrate-derived diazomethyl ketones. Implications for the difference in site specificity of halomethyl ketones for serine proteinases and cysteine proteinases and for stereoelectronic requirements in the papain catalytic mechanism. Biochem J. 1978 Nov 1;175(2):761–764. doi: 10.1042/bj1750761. [DOI] [PMC free article] [PubMed] [Google Scholar]
- Brocklehurst K. Two-protonic-state electrophiles as probes of enzyme mechanisms. Methods Enzymol. 1982;87:427–469. doi: 10.1016/s0076-6879(82)87026-2. [DOI] [PubMed] [Google Scholar]
- Craik C. S., Largman C., Fletcher T., Roczniak S., Barr P. J., Fletterick R., Rutter W. J. Redesigning trypsin: alteration of substrate specificity. Science. 1985 Apr 19;228(4697):291–297. doi: 10.1126/science.3838593. [DOI] [PubMed] [Google Scholar]
- Drenth J., Jansonius J. N., Koekoek R., Swen H. M., Wolthers B. G. Structure of papain. Nature. 1968 Jun 8;218(5145):929–932. doi: 10.1038/218929a0. [DOI] [PubMed] [Google Scholar]
- Drenth J., Kalk K. H., Swen H. M. Binding of chloromethyl ketone substrate analogues to crystalline papain. Biochemistry. 1976 Aug 24;15(17):3731–3738. doi: 10.1021/bi00662a014. [DOI] [PubMed] [Google Scholar]
- ERLANGER B. F., KOKOWSKY N., COHEN W. The preparation and properties of two new chromogenic substrates of trypsin. Arch Biochem Biophys. 1961 Nov;95:271–278. doi: 10.1016/0003-9861(61)90145-x. [DOI] [PubMed] [Google Scholar]
- Estell D. A., Graycar T. P., Miller J. V., Powers D. B., Wells J. A., Burnier J. P., Ng P. G. Probing steric and hydrophobic effects on enzyme-substrate interactions by protein engineering. Science. 1986 Aug 8;233(4764):659–663. doi: 10.1126/science.233.4764.659. [DOI] [PubMed] [Google Scholar]
- Hanzlik R. P., Jacober S. P., Zygmunt J. Reversible binding of peptide aldehydes to papain. Structure-activity relationships. Biochim Biophys Acta. 1991 Jan 23;1073(1):33–42. doi: 10.1016/0304-4165(91)90179-k. [DOI] [PubMed] [Google Scholar]
- Hollaway M. R., Antonini E., Brunori M. The pH-dependence of rates of individual steps in ficin catalysis. Eur J Biochem. 1971 Dec;24(2):332–341. doi: 10.1111/j.1432-1033.1971.tb19691.x. [DOI] [PubMed] [Google Scholar]
- Husain S. S., Lowe G. The amino acid sequence around the active-site cysteine and histidine residues, and the buried cysteine residue in ficin. Biochem J. 1970 Apr;117(2):333–340. doi: 10.1042/bj1170333. [DOI] [PMC free article] [PubMed] [Google Scholar]
- Kamphuis I. G., Drenth J., Baker E. N. Thiol proteases. Comparative studies based on the high-resolution structures of papain and actinidin, and on amino acid sequence information for cathepsins B and H, and stem bromelain. J Mol Biol. 1985 Mar 20;182(2):317–329. doi: 10.1016/0022-2836(85)90348-1. [DOI] [PubMed] [Google Scholar]
- Kamphuis I. G., Kalk K. H., Swarte M. B., Drenth J. Structure of papain refined at 1.65 A resolution. J Mol Biol. 1984 Oct 25;179(2):233–256. doi: 10.1016/0022-2836(84)90467-4. [DOI] [PubMed] [Google Scholar]
- Knowles J. R. Tinkering with enzymes: what are we learning? Science. 1987 Jun 5;236(4806):1252–1258. doi: 10.1126/science.3296192. [DOI] [PubMed] [Google Scholar]
- Kowlessur D., O'Driscoll M., Topham C. M., Templeton W., Thomas E. W., Brocklehurst K. The interplay of electrostatic fields and binding interactions determining catalytic-site reactivity in actinidin. A possible origin of differences in the behaviour of actinidin and papain. Biochem J. 1989 Apr 15;259(2):443–452. doi: 10.1042/bj2590443. [DOI] [PMC free article] [PubMed] [Google Scholar]
- Kowlessur D., Thomas E. W., Topham C. M., Templeton W., Brocklehurst K. Dependence of the P2-S2 stereochemical selectivity of papain on the nature of the catalytic-site chemistry. Quantification of selectivity in the catalysed hydrolysis of the enantiomeric N-acetylphenylalanylglycine 4-nitroanilides. Biochem J. 1990 Mar 15;266(3):653–660. doi: 10.1042/bj2660653. [DOI] [PMC free article] [PubMed] [Google Scholar]
- Kowlessur D., Topham C. M., Thomas E. W., O'Driscoll M., Templeton W., Brocklehurst K. Identification of signalling and non-signalling binding contributions to enzyme reactivity. Alternative combinations of binding interactions provide for change in transition-state geometry in reactions of papain. Biochem J. 1989 Mar 15;258(3):755–764. doi: 10.1042/bj2580755. [DOI] [PMC free article] [PubMed] [Google Scholar]
- Lowe G., Yuthavong Y. Kinetic specificity in papain-catalysed hydrolyses. Biochem J. 1971 Aug;124(1):107–115. doi: 10.1042/bj1240107. [DOI] [PMC free article] [PubMed] [Google Scholar]
- Lowe G., Yuthavong Y. pH-dependence and structure-activity relationships in the papain-catalysed hydrolysis of anilides. Biochem J. 1971 Aug;124(1):117–122. doi: 10.1042/bj1240117. [DOI] [PMC free article] [PubMed] [Google Scholar]
- Malthouse J. P., Brocklehurst K. Preparation of fully active ficin from Ficus glabrata by covalent chromatography and characterization of its active centre by using 2,2'-depyridyl disulphide as a reactivity probe. Biochem J. 1976 Nov;159(2):221–234. doi: 10.1042/bj1590221. [DOI] [PMC free article] [PubMed] [Google Scholar]
- Salih E., Malthouse J. P., Kowlessur D., Jarvis M., O'Driscoll M., Brocklehurst K. Differences in the chemical and catalytic characteristics of two crystallographically 'identical' enzyme catalytic sites. Characterization of actinidin and papain by a combination of pH-dependent substrate catalysis kinetics and reactivity probe studies targeted on the catalytic-site thiol group and its immediate microenvironment. Biochem J. 1987 Oct 1;247(1):181–193. doi: 10.1042/bj2470181. [DOI] [PMC free article] [PubMed] [Google Scholar]
- Templeton W., Kowlessur D., Thomas E. W., Topham C. M., Brocklehurst K. A re-appraisal of the structural basis of stereochemical recognition in papain. Insensitivity of binding-site-catalytic-site signalling to P2-chirality in a time-dependent inhibition. Biochem J. 1990 Mar 15;266(3):645–651. doi: 10.1042/bj2660645. [DOI] [PMC free article] [PubMed] [Google Scholar]
- WILKINSON G. N. Statistical estimations in enzyme kinetics. Biochem J. 1961 Aug;80:324–332. doi: 10.1042/bj0800324. [DOI] [PMC free article] [PubMed] [Google Scholar]
- Wells J. A., Powers D. B., Bott R. R., Graycar T. P., Estell D. A. Designing substrate specificity by protein engineering of electrostatic interactions. Proc Natl Acad Sci U S A. 1987 Mar;84(5):1219–1223. doi: 10.1073/pnas.84.5.1219. [DOI] [PMC free article] [PubMed] [Google Scholar]