Abstract
The deep-sea tube worm Lamellibrachia, belonging to the Phylum Vestimentifera, contains two giant extracellular haemoglobins, a 3000 kDa haemoglobin and a 440 kDa haemoglobin. The former consists of four haem-containing chains (AI-AIV) and two linker chains (AV and AVI) for the assembly of the haem-containing chains [Suzuki, Takagi & Ohta (1988) Biochem. J. 255, 541-545]. The tube-worm haemoglobins are believed to have a function of transporting sulphide (H2S) to internal bacterial symbionts, as well as of facilitating O2 transport [Arp & Childress (1983) Science 219, 295-297]. We have determined the complete amino acid sequence of Lamellibrachia chain AIII by automated or manual Edman sequencing. The chain is composed of 144 amino acid residues, has three cysteine residues at positions 3, 74 and 133, and has a molecular mass of 16,620 Da, including a haem group. The sequence showed significant homology (30-50% identity) with those of haem-containing chains of annelid giant haemoglobins. Two of the three cysteine residues are located at the positions where an intrachain disulphide bridge is formed in all annelid chains, but the remaining one (Cys-74) was located at a unique position, compared with annelid chains. Since the chain AIII was shown to have a reactive thiol group in the intact 3000 kDa molecule by preliminary experiments, the cysteine residue at position 74 appears to be one of the most probable candidates for the sulphide-binding sites. A phylogenetic tree was constructed from nine chains of annelid giant haemoglobins and one chain of vestimentiferan tube-worm haemoglobin now determined. The tree clearly showed that Lamellibrachia chain AIII belongs to the family of strain A of annelid giant haemoglobins, and that the two classes of Annelida, polychaete and oligochaete, and the vestimentiferan tube worm diverged at almost the same time. H.p.l.c. patterns of peptides (Figs. 4-7), amino acid compositions of peptides (Table 2) and amino acid sequences of intact protein and peptides (Table 3) have been deposited as Supplementary Publication SUP 50154 (13 pages) at the British Library Document Supply Centre, Boston Spa, Wetherby, West Yorkshire LS23 7BQ, U.K., from whom copies can be obtained on the terms indicated in Biochem. J. (1990) 265, 5.
Full text
PDF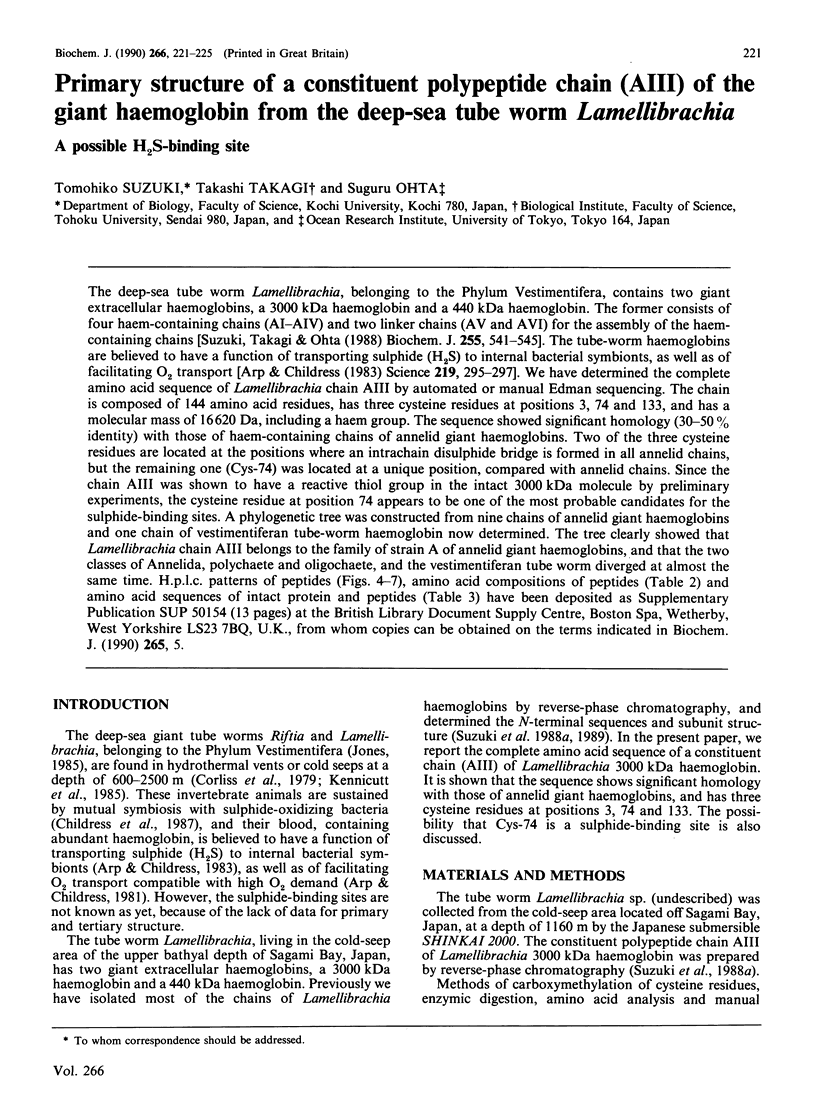
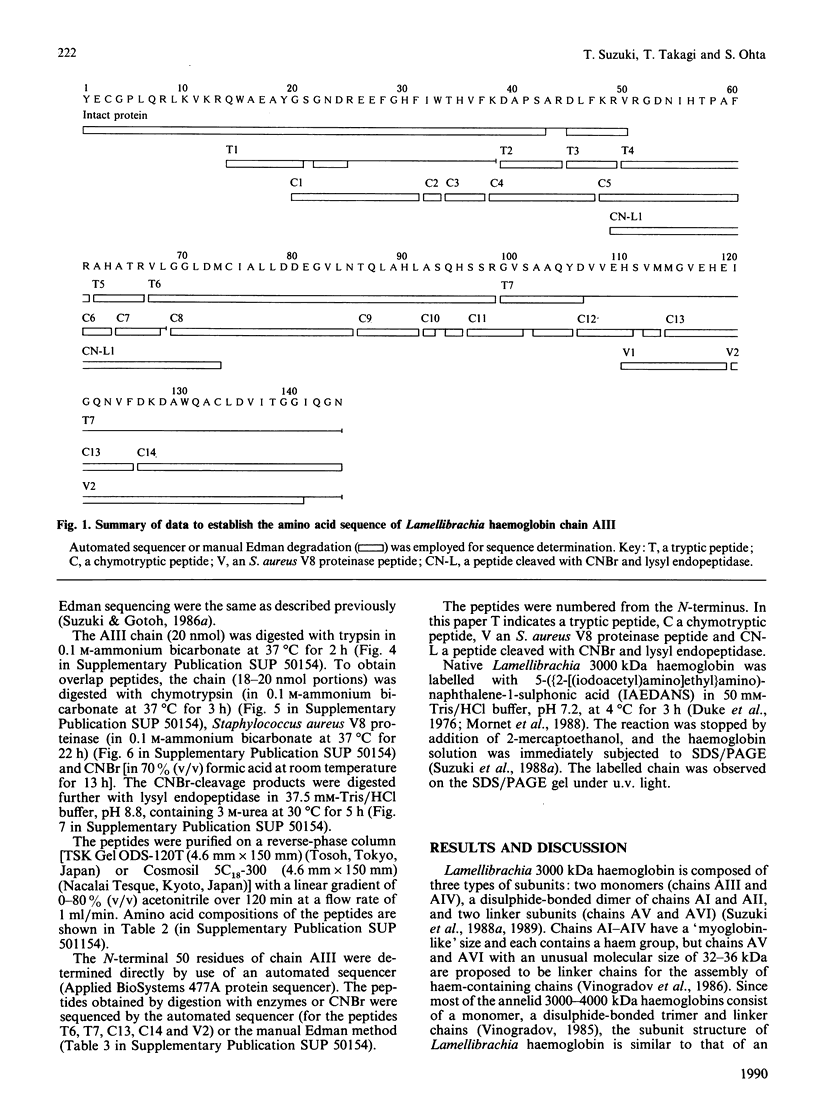
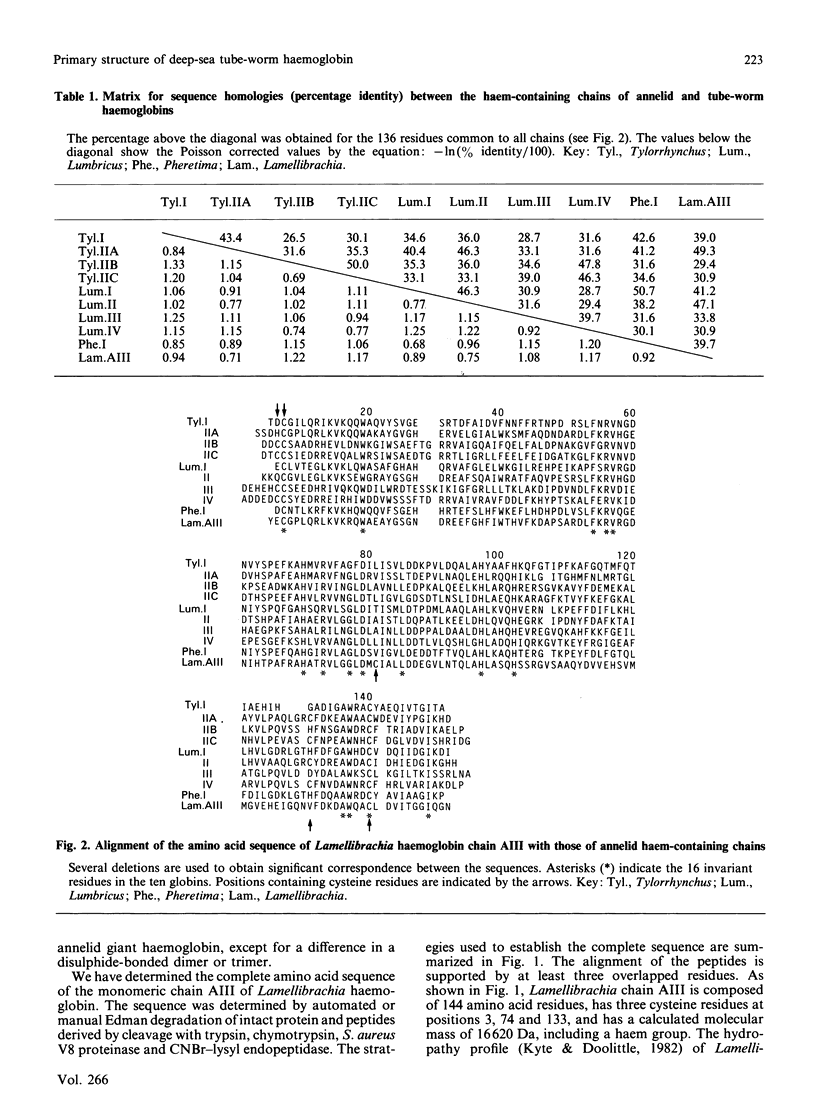
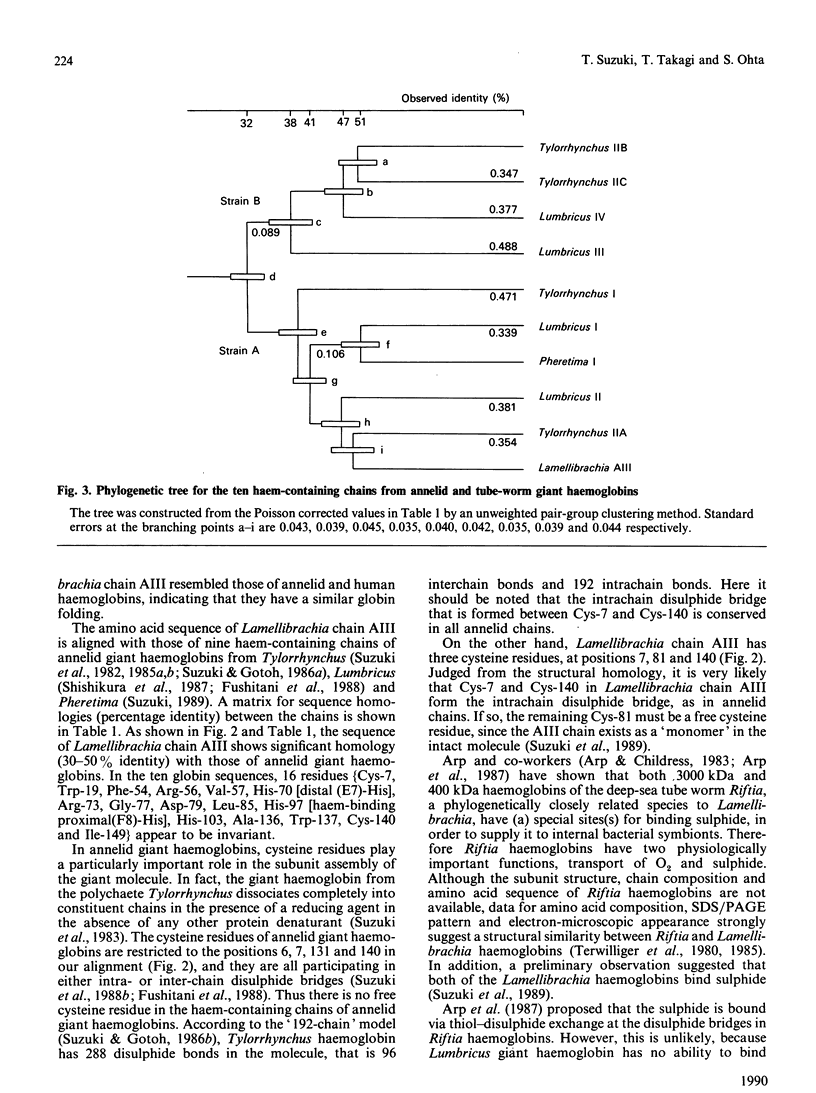
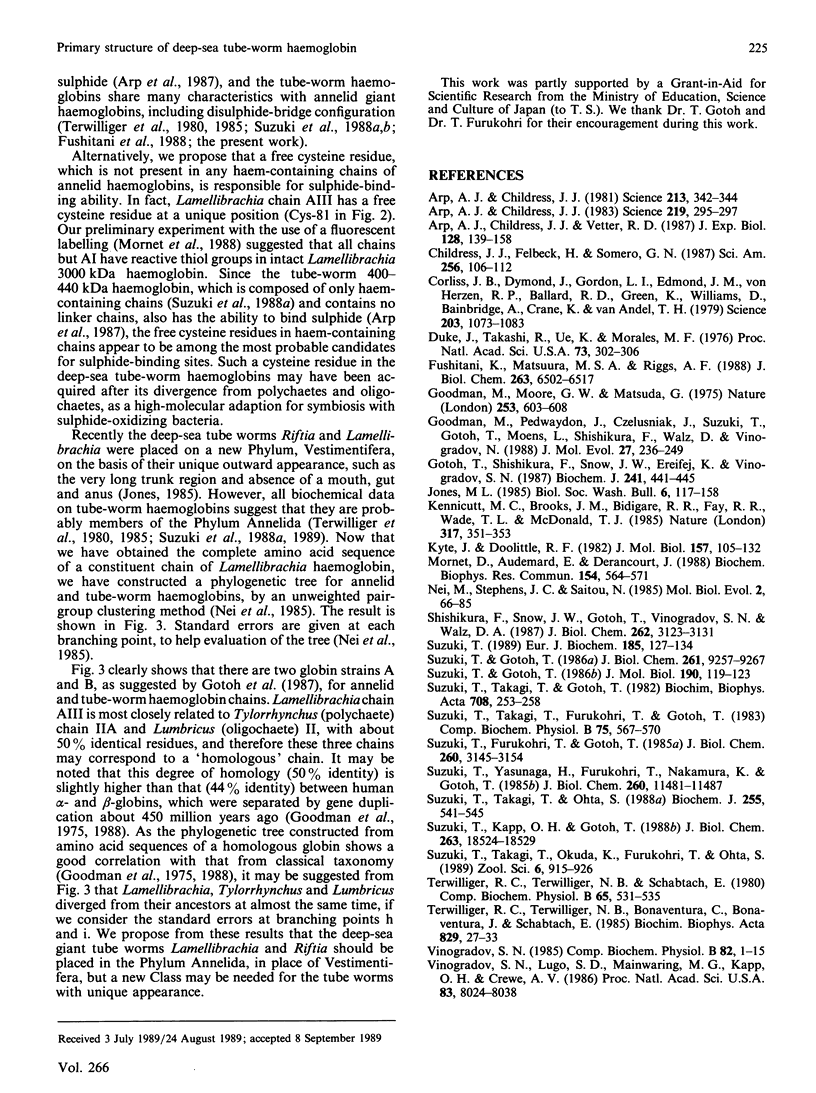
Selected References
These references are in PubMed. This may not be the complete list of references from this article.
- Arp A. J., Childress J. J. Blood function in the hydrothermal vent vestimentiferan tube worm. Science. 1981 Jul 17;213(4505):342–344. doi: 10.1126/science.213.4505.342. [DOI] [PubMed] [Google Scholar]
- Arp A. J., Childress J. J. Sulfide Binding by the Blood of the Hydrothermal Vent Tube Worm Riftia pachyptila. Science. 1983 Jan 21;219(4582):295–297. doi: 10.1126/science.219.4582.295. [DOI] [PubMed] [Google Scholar]
- Corliss J. B., Dymond J., Gordon L. I., Edmond J. M., von Herzen R. P., Ballard R. D., Green K., Williams D., Bainbridge A., Crane K., van Andel T. H. Submarine thermal sprirngs on the galapagos rift. Science. 1979 Mar 16;203(4385):1073–1083. doi: 10.1126/science.203.4385.1073. [DOI] [PubMed] [Google Scholar]
- Duke J., Takashi R., Ue K., Morales M. F. Reciprocal reactivities of specific thiols when actin binds to myosin. Proc Natl Acad Sci U S A. 1976 Feb;73(2):302–306. doi: 10.1073/pnas.73.2.302. [DOI] [PMC free article] [PubMed] [Google Scholar]
- Fushitani K., Matsuura M. S., Riggs A. F. The amino acid sequences of chains a, b, and c that form the trimer subunit of the extracellular hemoglobin from Lumbricus terrestris. J Biol Chem. 1988 May 15;263(14):6502–6517. [PubMed] [Google Scholar]
- Goodman M., Moore G. W., Matsuda G. Darwinian evolution in the genealogy of haemoglobin. Nature. 1975 Feb 20;253(5493):603–608. doi: 10.1038/253603a0. [DOI] [PubMed] [Google Scholar]
- Goodman M., Pedwaydon J., Czelusniak J., Suzuki T., Gotoh T., Moens L., Shishikura F., Walz D., Vinogradov S. An evolutionary tree for invertebrate globin sequences. J Mol Evol. 1988;27(3):236–249. doi: 10.1007/BF02100080. [DOI] [PubMed] [Google Scholar]
- Gotoh T., Shishikura F., Snow J. W., Ereifej K. I., Vinogradov S. N., Walz D. A. Two globin strains in the giant annelid extracellular haemoglobins. Biochem J. 1987 Jan 15;241(2):441–445. doi: 10.1042/bj2410441. [DOI] [PMC free article] [PubMed] [Google Scholar]
- Kyte J., Doolittle R. F. A simple method for displaying the hydropathic character of a protein. J Mol Biol. 1982 May 5;157(1):105–132. doi: 10.1016/0022-2836(82)90515-0. [DOI] [PubMed] [Google Scholar]
- Mornet D., Audemard E., Derancourt J. Identification of a 15 kilodalton actin binding region on gizzard caldesmon probed by chemical cross-linking. Biochem Biophys Res Commun. 1988 Jul 29;154(2):564–571. doi: 10.1016/0006-291x(88)90177-5. [DOI] [PubMed] [Google Scholar]
- Nei M., Stephens J. C., Saitou N. Methods for computing the standard errors of branching points in an evolutionary tree and their application to molecular data from humans and apes. Mol Biol Evol. 1985 Jan;2(1):66–85. doi: 10.1093/oxfordjournals.molbev.a040333. [DOI] [PubMed] [Google Scholar]
- Shishikura F., Snow J. W., Gotoh T., Vinogradov S. N., Walz D. A. Amino acid sequence of the monomer subunit of the extracellular hemoglobin of Lumbricus terrestris. J Biol Chem. 1987 Mar 5;262(7):3123–3131. [PubMed] [Google Scholar]
- Suzuki T. Amino acid sequence of the monomer subunit of the giant multisubunit hemoglobin from the earthworm Pheretima sieboldi. Eur J Biochem. 1989 Oct 20;185(1):127–134. doi: 10.1111/j.1432-1033.1989.tb15092.x. [DOI] [PubMed] [Google Scholar]
- Suzuki T., Furukohri T., Gotoh T. Subunit structure of extracellular hemoglobin from the polychaete Tylorrhynchus heterochaetus and amino acid sequence of the constituent polypeptide chain (IIC). J Biol Chem. 1985 Mar 10;260(5):3145–3154. [PubMed] [Google Scholar]
- Suzuki T., Gotoh T. Subunit assembly of giant haemoglobin from the polychaete Tylorrhynchus heterochaetus. J Mol Biol. 1986 Jul 5;190(1):119–123. doi: 10.1016/0022-2836(86)90081-1. [DOI] [PubMed] [Google Scholar]
- Suzuki T., Gotoh T. The complete amino acid sequence of giant multisubunit hemoglobin from the polychaete Tylorrhynchus heterochaetus. J Biol Chem. 1986 Jul 15;261(20):9257–9267. [PubMed] [Google Scholar]
- Suzuki T., Kapp O. H., Gotoh T. Novel S-S loops in the giant hemoglobin of Tylorrhynchus heterochaetus. J Biol Chem. 1988 Dec 5;263(34):18524–18529. [PubMed] [Google Scholar]
- Suzuki T., Takagi T., Ohta S. N-terminal amino acid sequence of the deep-sea tube worm haemoglobin remarkably resembles that of annelid haemoglobin. Biochem J. 1988 Oct 15;255(2):541–545. [PMC free article] [PubMed] [Google Scholar]
- Suzuki T., Yasunaga H., Furukohri T., Nakamura K., Gotoh T. Amino acid sequence of polypeptide chain IIB of extracellular hemoglobin from the polychaete Tylorrhynchus heterochaetus. J Biol Chem. 1985 Sep 25;260(21):11481–11487. [PubMed] [Google Scholar]
- Vinogradov S. N., Lugo S. D., Mainwaring M. G., Kapp O. H., Crewe A. V. Bracelet protein: a quaternary structure proposed for the giant extracellular hemoglobin of Lumbricus terrestris. Proc Natl Acad Sci U S A. 1986 Nov;83(21):8034–8038. doi: 10.1073/pnas.83.21.8034. [DOI] [PMC free article] [PubMed] [Google Scholar]
- Vinogradov S. N. The structure of invertebrate extracellular hemoglobins (erythrocruorins and chlorocruorins). Comp Biochem Physiol B. 1985;82(1):1–15. doi: 10.1016/0305-0491(85)90120-8. [DOI] [PubMed] [Google Scholar]