Abstract
The present study characterized total and myofibrillar protein breakdown rates in a muscle preparation frequently used in vitro, i.e. incubated extensor digitorum longus (EDL) and soleus (SOL) muscles of young rats. Total and myofibrillar protein breakdown rates were assessed by determining net production by the incubated muscles of tyrosine and 3-methylhistidine (3-MH) respectively. Both amino acids were determined by h.p.l.c. Both total and myofibrillar protein breakdown rates were higher in SOL than in EDL muscles and were decreased by incubating the muscles maintained at resting length, rather than flaccid. After fasting for 72 h, total protein breakdown (i.e. tyrosine release) was increased by 73% and 138% in EDL muscles incubated flaccid and at resting length respectively. Net production of tyrosine by SOL muscle was not significantly altered by fasting. In contrast, myofibrillar protein degradation (i.e. 3-MH release) was markedly increased by fasting in both muscles. When tissue was incubated in the presence of 1 munit of insulin/ml, total protein breakdown rate was inhibited by 17-20%, and the response to the hormone was similar in muscles incubated flaccid or at resting length. In contrast, myofibrillar protein breakdown rate was not altered by insulin in any of the muscle preparations. The results support the concepts of individual regulation of myofibrillar and non-myofibrillar proteins and of different effects of various conditions on protein breakdown in different types of skeletal muscle. Thus determination of both tyrosine and 3-MH production in red and white muscle is important for a more complete understanding of protein regulation in skeletal muscle.
Full text
PDF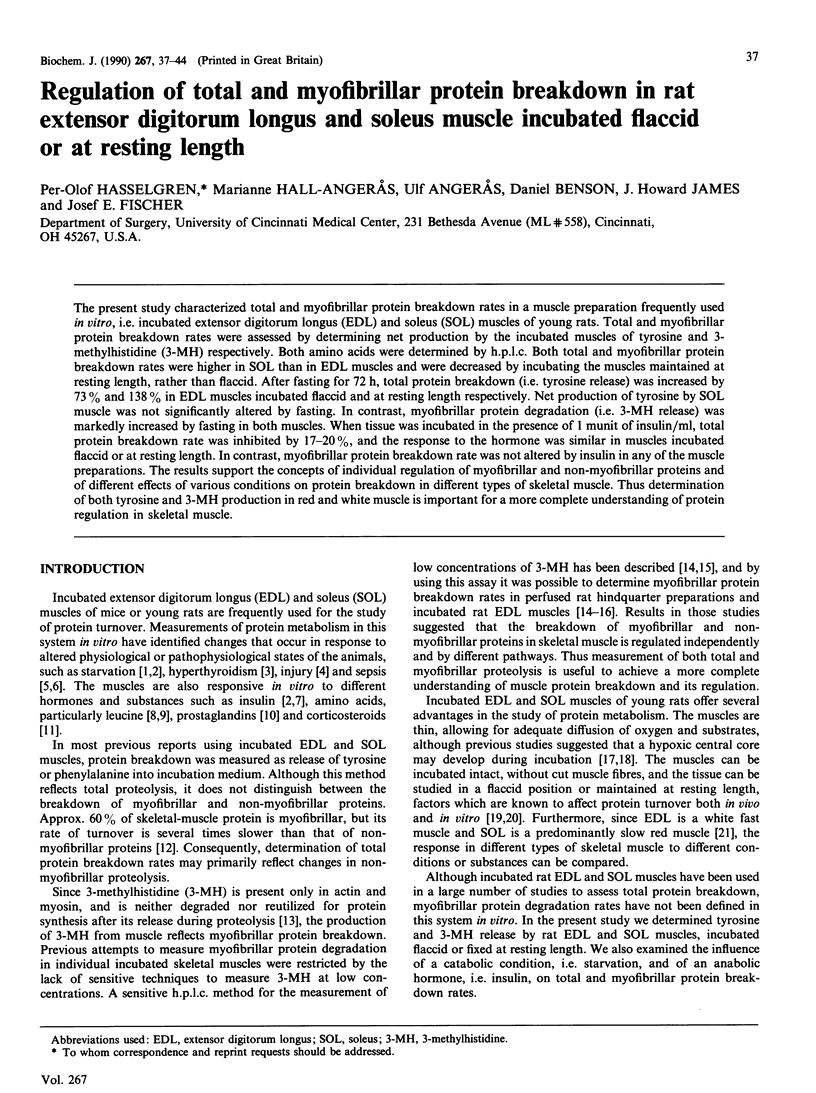
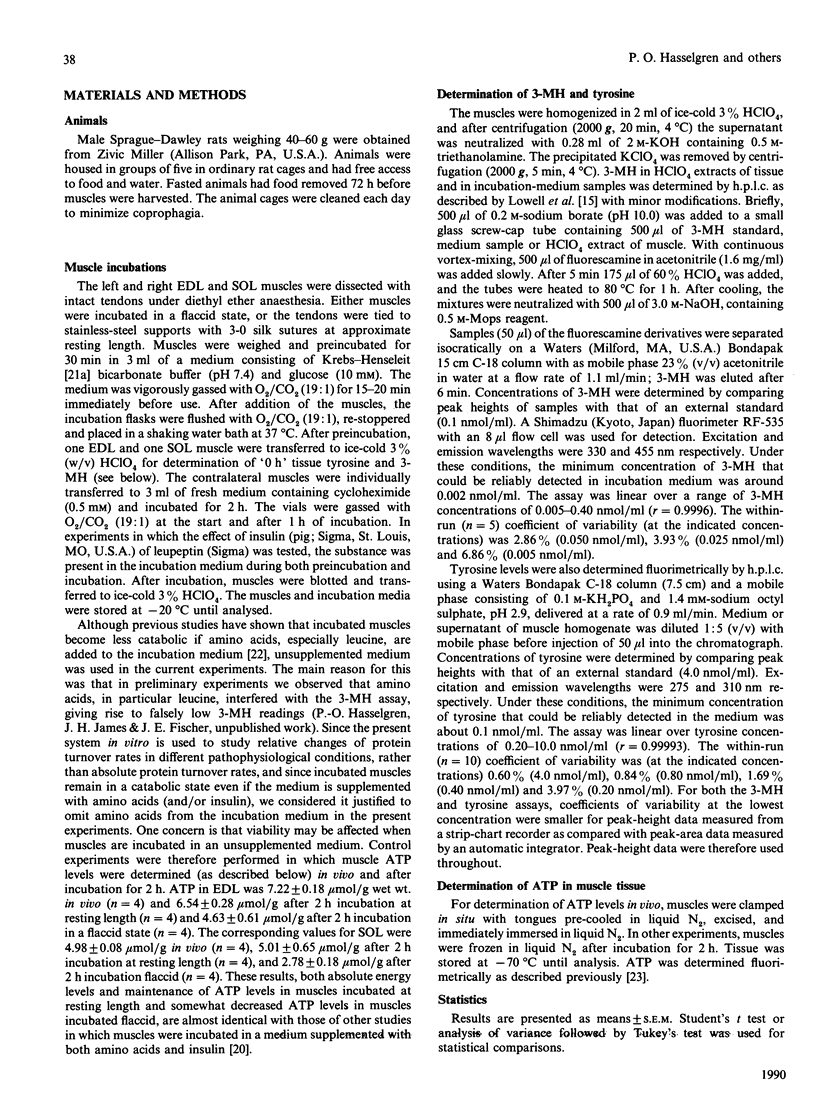
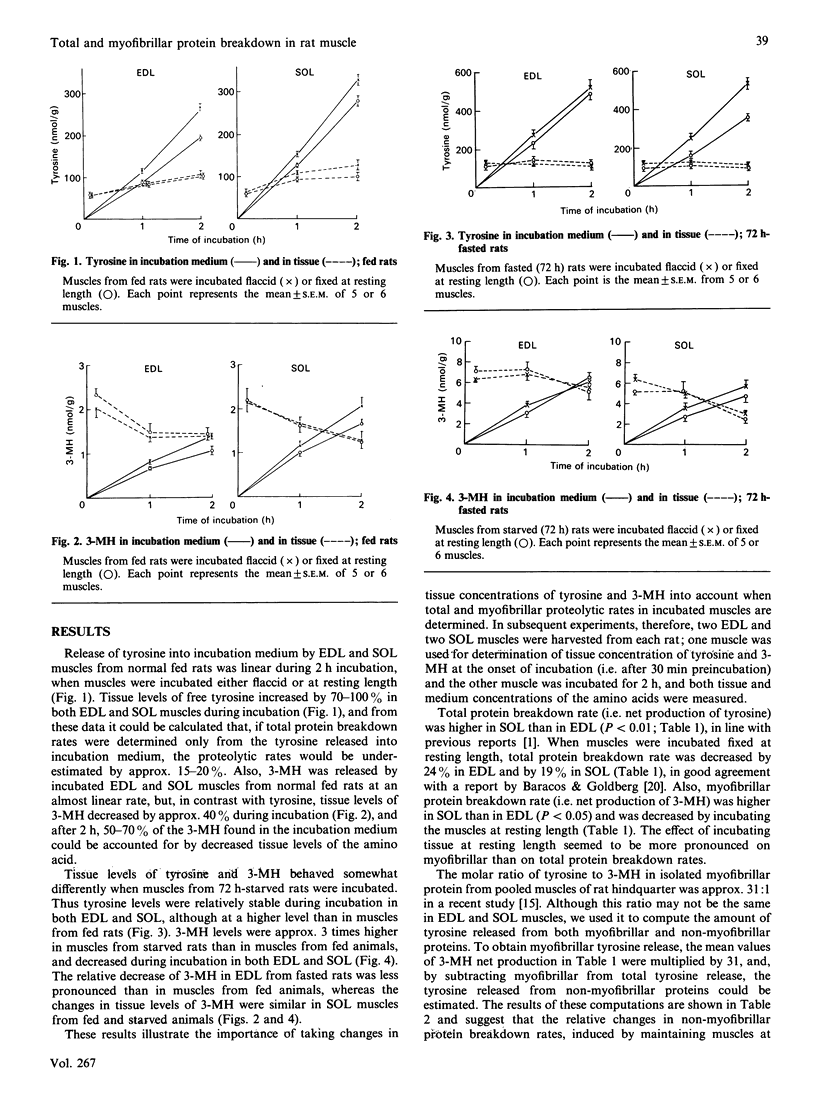
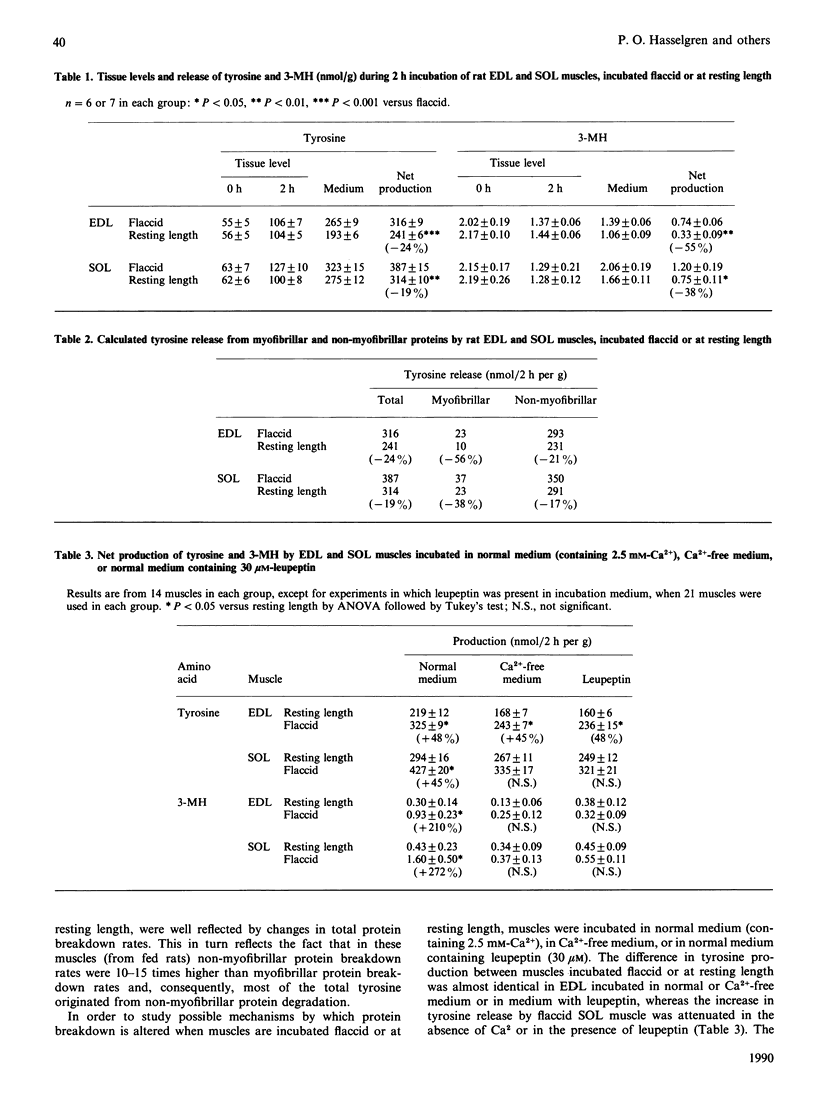
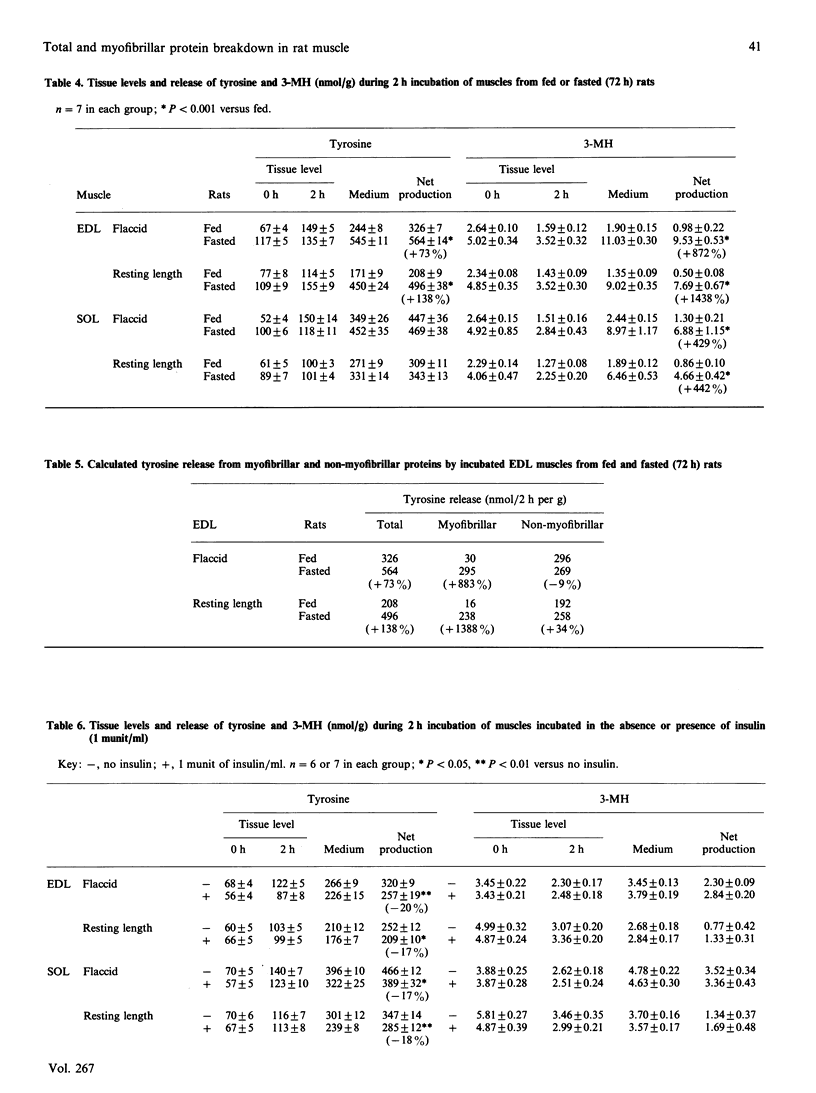
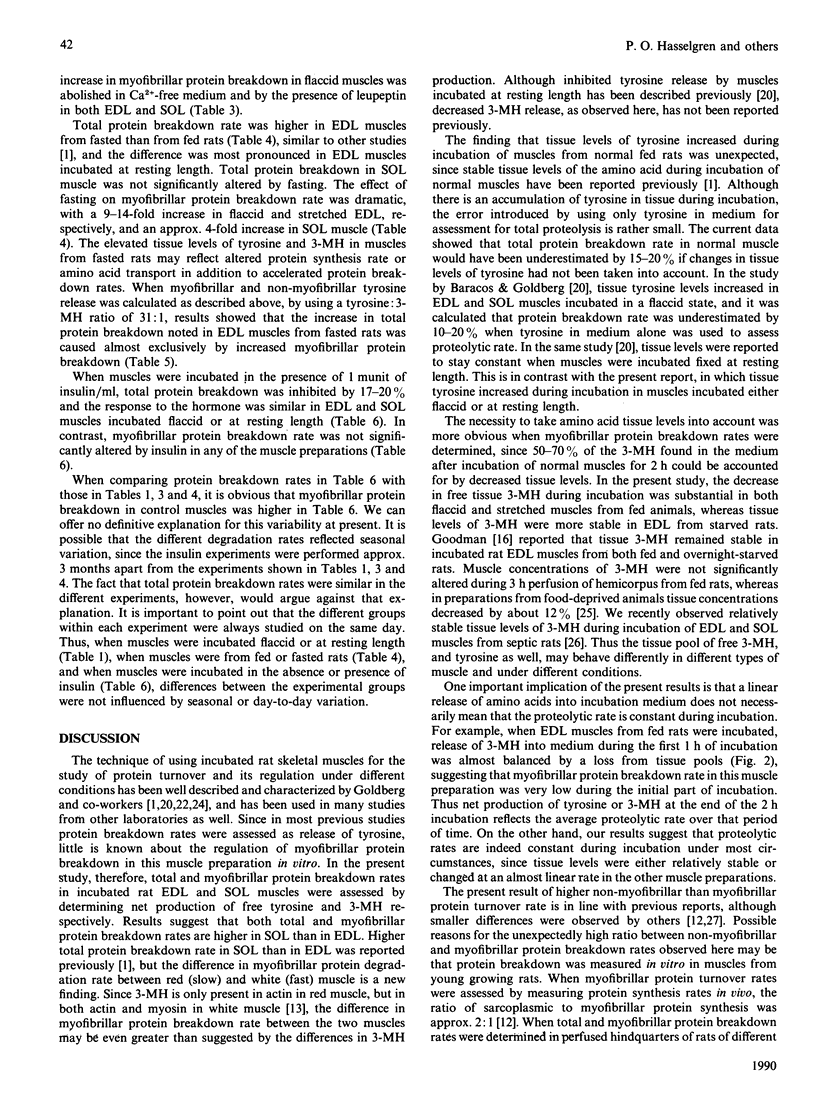
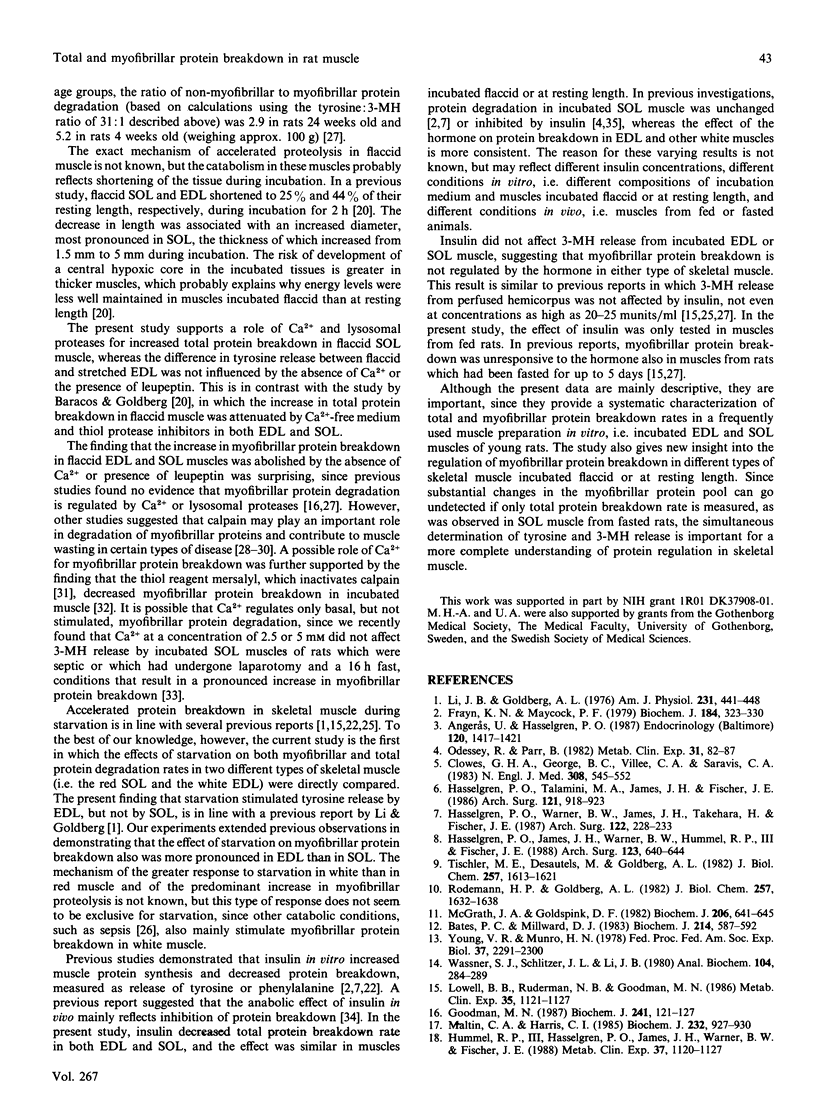
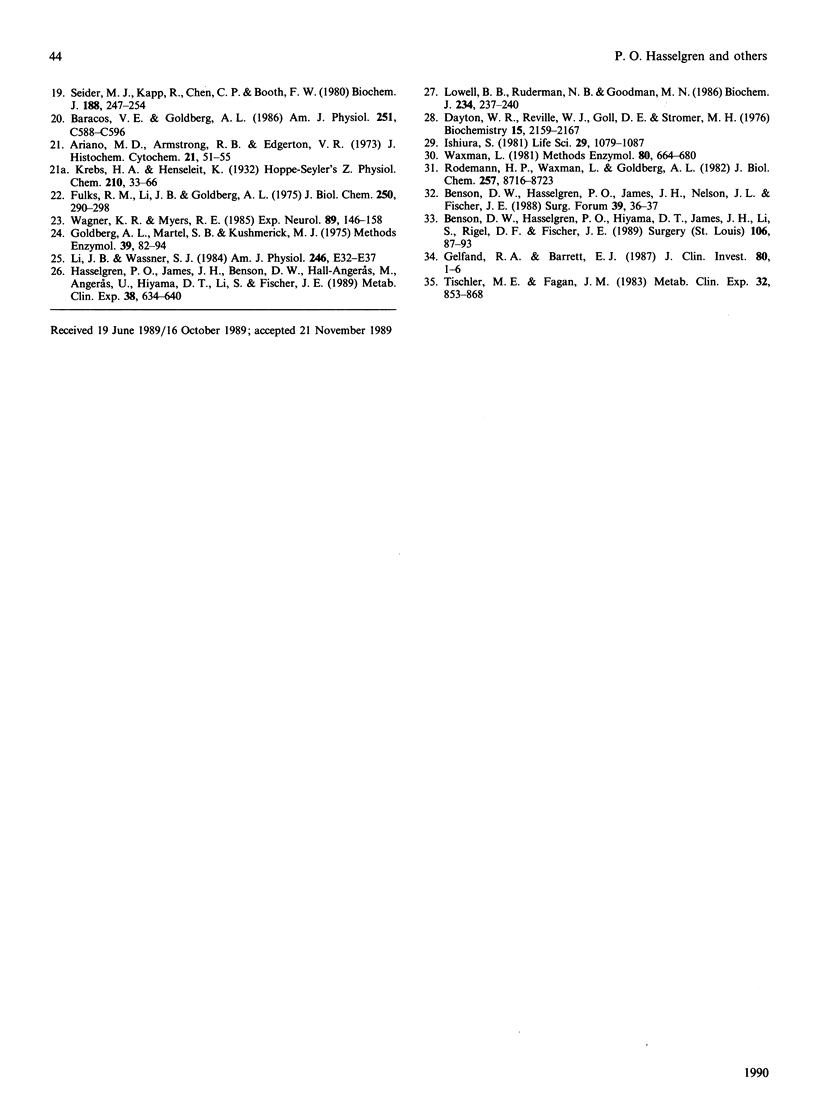
Selected References
These references are in PubMed. This may not be the complete list of references from this article.
- Angerås U., Hasselgren P. O. Protein degradation in skeletal muscle during experimental hyperthyroidism in rats and the effect of beta-blocking agents. Endocrinology. 1987 Apr;120(4):1417–1421. doi: 10.1210/endo-120-4-1417. [DOI] [PubMed] [Google Scholar]
- Ariano M. A., Armstrong R. B., Edgerton V. R. Hindlimb muscle fiber populations of five mammals. J Histochem Cytochem. 1973 Jan;21(1):51–55. doi: 10.1177/21.1.51. [DOI] [PubMed] [Google Scholar]
- Baracos V. E., Goldberg A. L. Maintenance of normal length improves protein balance and energy status in isolated rat skeletal muscles. Am J Physiol. 1986 Oct;251(4 Pt 1):C588–C596. doi: 10.1152/ajpcell.1986.251.4.C588. [DOI] [PubMed] [Google Scholar]
- Bates P. C., Millward D. J. Myofibrillar protein turnover. Synthesis rates of myofibrillar and sarcoplasmic protein fractions in different muscles and the changes observed during postnatal development and in response to feeding and starvation. Biochem J. 1983 Aug 15;214(2):587–592. doi: 10.1042/bj2140587. [DOI] [PMC free article] [PubMed] [Google Scholar]
- Benson D. W., Hasselgren P. O., Hiyama D. T., James J. H., Li S., Rigel D. F., Fischer J. E. Effect of sepsis on calcium uptake and content in skeletal muscle and regulation in vitro by calcium of total and myofibrillar protein breakdown in control and septic muscle: results from a preliminary study. Surgery. 1989 Jul;106(1):87–93. [PubMed] [Google Scholar]
- Clowes G. H., Jr, George B. C., Villee C. A., Jr, Saravis C. A. Muscle proteolysis induced by a circulating peptide in patients with sepsis or trauma. N Engl J Med. 1983 Mar 10;308(10):545–552. doi: 10.1056/NEJM198303103081001. [DOI] [PubMed] [Google Scholar]
- Dayton W. R., Reville W. J., Goll D. E., Stromer M. H. A Ca2+-activated protease possibly involved in myofibrillar protein turnover. Partial characterization of the purified enzyme. Biochemistry. 1976 May 18;15(10):2159–2167. doi: 10.1021/bi00655a020. [DOI] [PubMed] [Google Scholar]
- Frayn K. N., Maycock P. F. Regulation of protein metabolism by a physiological concentration of insulin in mouse soleus and extensor digitorum longus muscles. Effects of starvation and scald injury. Biochem J. 1979 Nov 15;184(2):323–330. doi: 10.1042/bj1840323. [DOI] [PMC free article] [PubMed] [Google Scholar]
- Fulks R. M., Li J. B., Goldberg A. L. Effects of insulin, glucose, and amino acids on protein turnover in rat diaphragm. J Biol Chem. 1975 Jan 10;250(1):290–298. [PubMed] [Google Scholar]
- Gelfand R. A., Barrett E. J. Effect of physiologic hyperinsulinemia on skeletal muscle protein synthesis and breakdown in man. J Clin Invest. 1987 Jul;80(1):1–6. doi: 10.1172/JCI113033. [DOI] [PMC free article] [PubMed] [Google Scholar]
- Goldberg A. L., Martel S. B., Kushmerick M. J. In vitro preparations of the diaphragm and other skeletal muscles. Methods Enzymol. 1975;39:82–94. doi: 10.1016/s0076-6879(75)39012-5. [DOI] [PubMed] [Google Scholar]
- Goodman M. N. Differential effects of acute changes in cell Ca2+ concentration on myofibrillar and non-myofibrillar protein breakdown in the rat extensor digitorum longus muscle in vitro. Assessment by production of tyrosine and N tau-methylhistidine. Biochem J. 1987 Jan 1;241(1):121–127. doi: 10.1042/bj2410121. [DOI] [PMC free article] [PubMed] [Google Scholar]
- Hasselgren P. O., James J. H., Benson D. W., Hall-Angerås M., Angerås U., Hiyama D. T., Li S., Fischer J. E. Total and myofibrillar protein breakdown in different types of rat skeletal muscle: effects of sepsis and regulation by insulin. Metabolism. 1989 Jul;38(7):634–640. doi: 10.1016/0026-0495(89)90100-5. [DOI] [PubMed] [Google Scholar]
- Hasselgren P. O., James J. H., Warner B. W., Hummel R. P., 3rd, Fischer J. E. Protein synthesis and degradation in skeletal muscle from septic rats. Response to leucine and alpha-ketoisocaproic acid. Arch Surg. 1988 May;123(5):640–644. doi: 10.1001/archsurg.1988.01400290126022. [DOI] [PubMed] [Google Scholar]
- Hasselgren P. O., Talamini M., James J. H., Fischer J. E. Protein metabolism in different types of skeletal muscle during early and late sepsis in rats. Arch Surg. 1986 Aug;121(8):918–923. doi: 10.1001/archsurg.1986.01400080064011. [DOI] [PubMed] [Google Scholar]
- Hasselgren P. O., Warner B. W., James J. H., Takehara H., Fischer J. E. Effect of insulin on amino acid uptake and protein turnover in skeletal muscle from septic rats. Evidence for insulin resistance of protein breakdown. Arch Surg. 1987 Feb;122(2):228–233. doi: 10.1001/archsurg.1987.01400140110015. [DOI] [PubMed] [Google Scholar]
- Hummel R. P., 3rd, Hasselgren P. O., James J. H., Warner B. W., Fischer J. E. The effect of sepsis in rats on skeletal muscle protein synthesis in vivo and in periphery and central core of incubated muscle preparations in vitro. Metabolism. 1988 Dec;37(12):1120–1127. doi: 10.1016/0026-0495(88)90187-4. [DOI] [PubMed] [Google Scholar]
- Ishiura S. Calcium-dependent proteolysis in living cells. Life Sci. 1981 Sep 14;29(11):1079–1087. doi: 10.1016/0024-3205(81)90194-6. [DOI] [PubMed] [Google Scholar]
- Li J. B., Goldberg A. L. Effects of food deprivation on protein synthesis and degradation in rat skeletal muscles. Am J Physiol. 1976 Aug;231(2):441–448. doi: 10.1152/ajplegacy.1976.231.2.441. [DOI] [PubMed] [Google Scholar]
- Li J. B., Wassner S. J. Effects of food deprivation and refeeding on total protein and actomyosin degradation. Am J Physiol. 1984 Jan;246(1 Pt 1):E32–E37. doi: 10.1152/ajpendo.1984.246.1.E32. [DOI] [PubMed] [Google Scholar]
- Lowell B. B., Ruderman N. B., Goodman M. N. Evidence that lysosomes are not involved in the degradation of myofibrillar proteins in rat skeletal muscle. Biochem J. 1986 Feb 15;234(1):237–240. doi: 10.1042/bj2340237. [DOI] [PMC free article] [PubMed] [Google Scholar]
- Lowell B. B., Ruderman N. B., Goodman M. N. Regulation of myofibrillar protein degradation in rat skeletal muscle during brief and prolonged starvation. Metabolism. 1986 Dec;35(12):1121–1127. doi: 10.1016/0026-0495(86)90025-9. [DOI] [PubMed] [Google Scholar]
- Maltin C. A., Harris C. I. Morphological observations and rates of protein synthesis in rat muscles incubated in vitro. Biochem J. 1985 Dec 15;232(3):927–930. doi: 10.1042/bj2320927. [DOI] [PMC free article] [PubMed] [Google Scholar]
- McGrath J. A., Goldspink D. F. Glucocorticoid action on protein synthesis and protein breakdown in isolated skeletal muscles. Biochem J. 1982 Sep 15;206(3):641–645. doi: 10.1042/bj2060641. [DOI] [PMC free article] [PubMed] [Google Scholar]
- Odessey R., Parr B. Effect of insulin and leucine on protein turnover in rat soleus muscle after burn injury. Metabolism. 1982 Jan;31(1):82–87. [PubMed] [Google Scholar]
- Rodemann H. P., Goldberg A. L. Arachidonic acid, prostaglandin E2 and F2 alpha influence rates of protein turnover in skeletal and cardiac muscle. J Biol Chem. 1982 Feb 25;257(4):1632–1638. [PubMed] [Google Scholar]
- Rodemann H. P., Waxman L., Goldberg A. L. The stimulation of protein degradation in muscle by Ca2+ is mediated by prostaglandin E2 and does not require the calcium-activated protease. J Biol Chem. 1982 Aug 10;257(15):8716–8723. [PubMed] [Google Scholar]
- Seider M. J., Kapp R., Chen C. P., Booth F. W. The effects of cutting or of stretching skeletal muscle in vitro on the rates of protein synthesis and degradation. Biochem J. 1980 Apr 15;188(1):247–254. doi: 10.1042/bj1880247. [DOI] [PMC free article] [PubMed] [Google Scholar]
- Tischler M. E., Desautels M., Goldberg A. L. Does leucine, leucyl-tRNA, or some metabolite of leucine regulate protein synthesis and degradation in skeletal and cardiac muscle? J Biol Chem. 1982 Feb 25;257(4):1613–1621. [PubMed] [Google Scholar]
- Tischler M. E., Fagan J. M. Response to trauma of protein, amino acid, and carbohydrate metabolism in injured and uninjured rat skeletal muscles. Metabolism. 1983 Sep;32(9):853–868. doi: 10.1016/0026-0495(83)90198-1. [DOI] [PubMed] [Google Scholar]
- Wagner K. R., Myers R. E. Topography of brain metabolites: rhesus monkey, goat, and cat. Exp Neurol. 1985 Jul;89(1):146–158. doi: 10.1016/0014-4886(85)90272-9. [DOI] [PubMed] [Google Scholar]
- Wassner S. J., Schlitzer J. L., Li J. B. A rapid, sensitive method for the determination of 3-methylhistidine levels in urine and plasma using high-pressure liquid chromatography. Anal Biochem. 1980 May 15;104(2):284–289. doi: 10.1016/0003-2697(80)90076-7. [DOI] [PubMed] [Google Scholar]
- Waxman L. Calcium-activated proteases in mammalian tissues. Methods Enzymol. 1981;80(Pt 100):664–680. doi: 10.1016/s0076-6879(81)80051-1. [DOI] [PubMed] [Google Scholar]
- Young V. R., Munro H. N. Ntau-methylhistidine (3-methylhistidine) and muscle protein turnover: an overview. Fed Proc. 1978 Jul;37(9):2291–2300. [PubMed] [Google Scholar]