Abstract
Based on the conformational dependence of the amide-I i.r. band, this paper explores the use of Fourier-transform i.r. spectroscopy methods to probe structural features of proteins present in native membranes from Torpedo highly enriched in acetylcholine receptor (AcChR). The interference of water absorbance on the amide-I spectral region has been eliminated through isotopic exchange by freeze-drying the membranes in the presence of trehalose to avoid protein denaturation induced by drying, followed by resuspension in deuterated water. AcChR-rich membrane samples prepared in such a way maintained an ability to undergo affinity-state transitions and to promote cation translocation in response to cholinergic agonists, which are functional characteristics of native untreated samples. The temperature-dependence of the i.r. spectrum indicates a massive loss of ordered protein structure, occurring at temperatures similar to those reported for thermal denaturation of the AcChR by differential scanning calorimetry and by thermal inactivation of alpha-bungarotoxin-binding sites on the AcChR [Artigues, Villar, Ferragut & Gonzalez-Ros (1987) Arch. Biochem. Biophys. 258, 33-41], thus suggesting that the observed i.r. spectral changes correspond to alterations in the structure of the AcChR protein. Furthermore, the presence of detergents as well as cholinergic agonists and antagonists produces spectral changes that are also consistent with the alterations in AcChR protein structure expected from previous calorimetric studies. In contrast with the information obtained by calorimetry, i.r. spectroscopy allows the contribution of secondary structural changes to be distinguished from the overall change in protein structure. Thus prolonged exposure to cholinergic agonists, which drives the AcChR protein into the desensitized state, produces only negligible alterations in the amide-I band shape, but increases substantially the thermal stability of the protein. This suggests that rearrangements in the tertiary or quaternary structure of the protein are more likely to occur than extensive changes in secondary structure as a consequence of AcChR desensitization.
Full text
PDF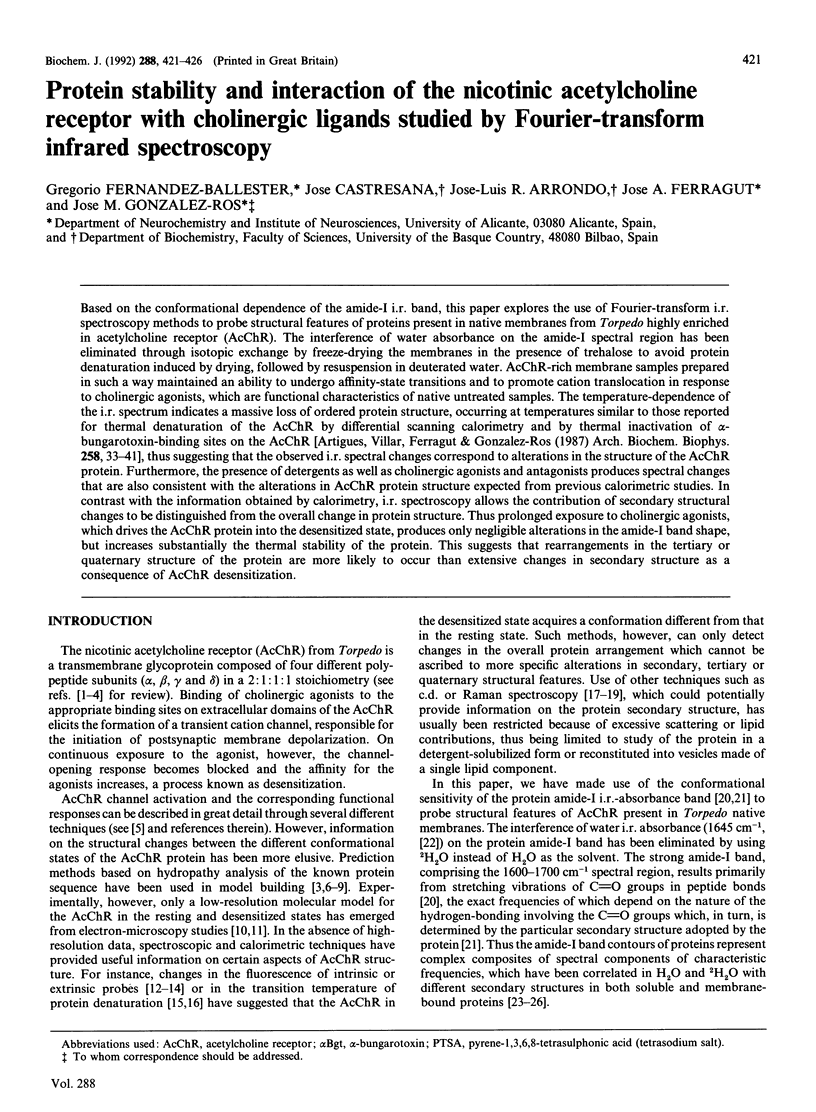
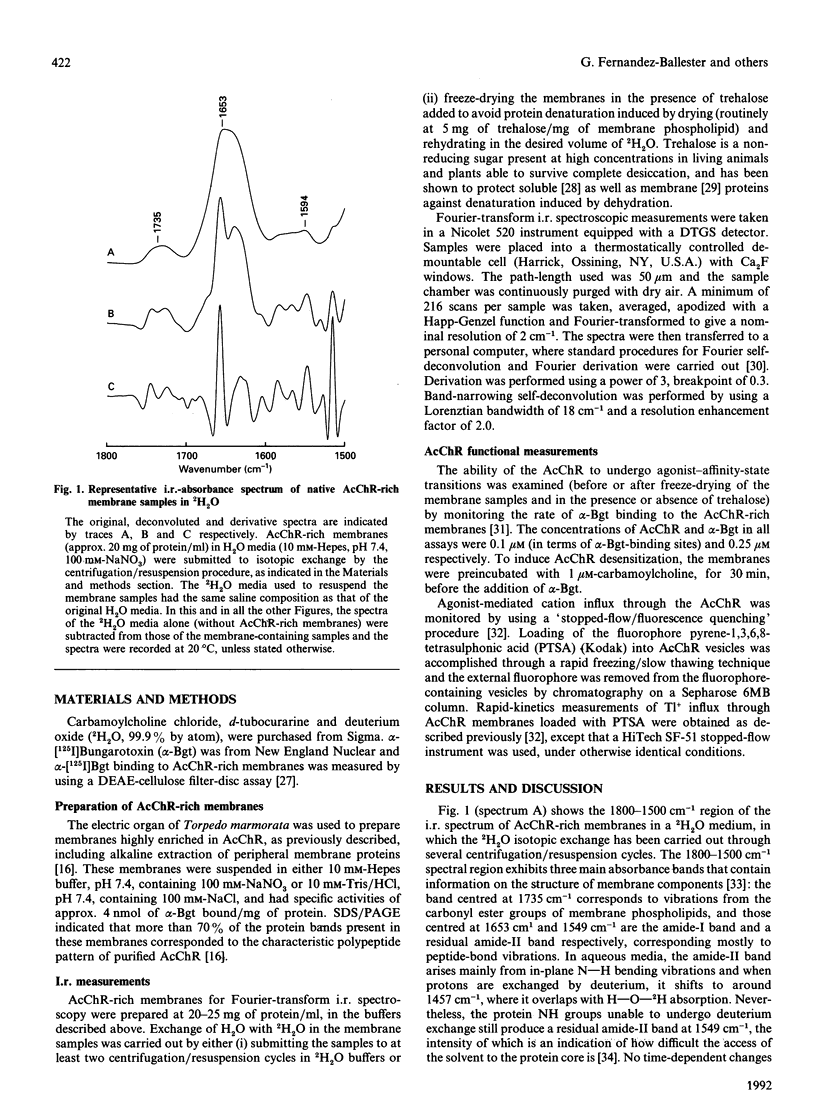
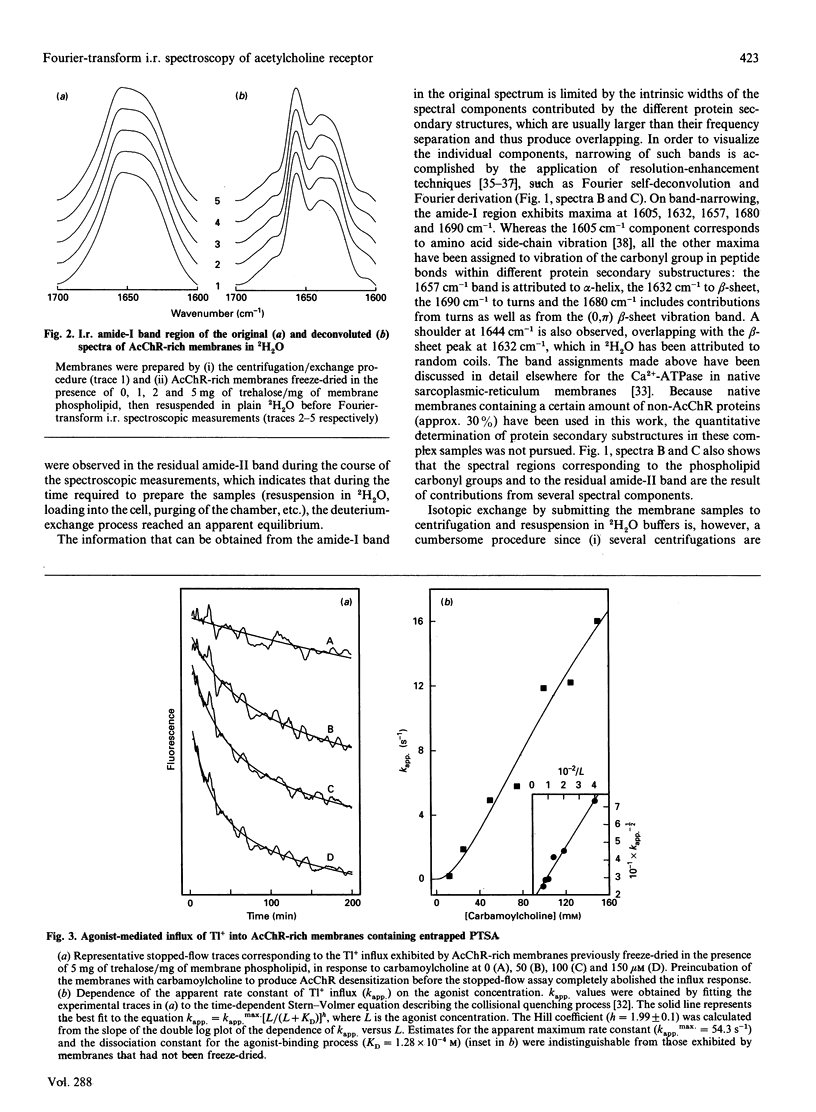
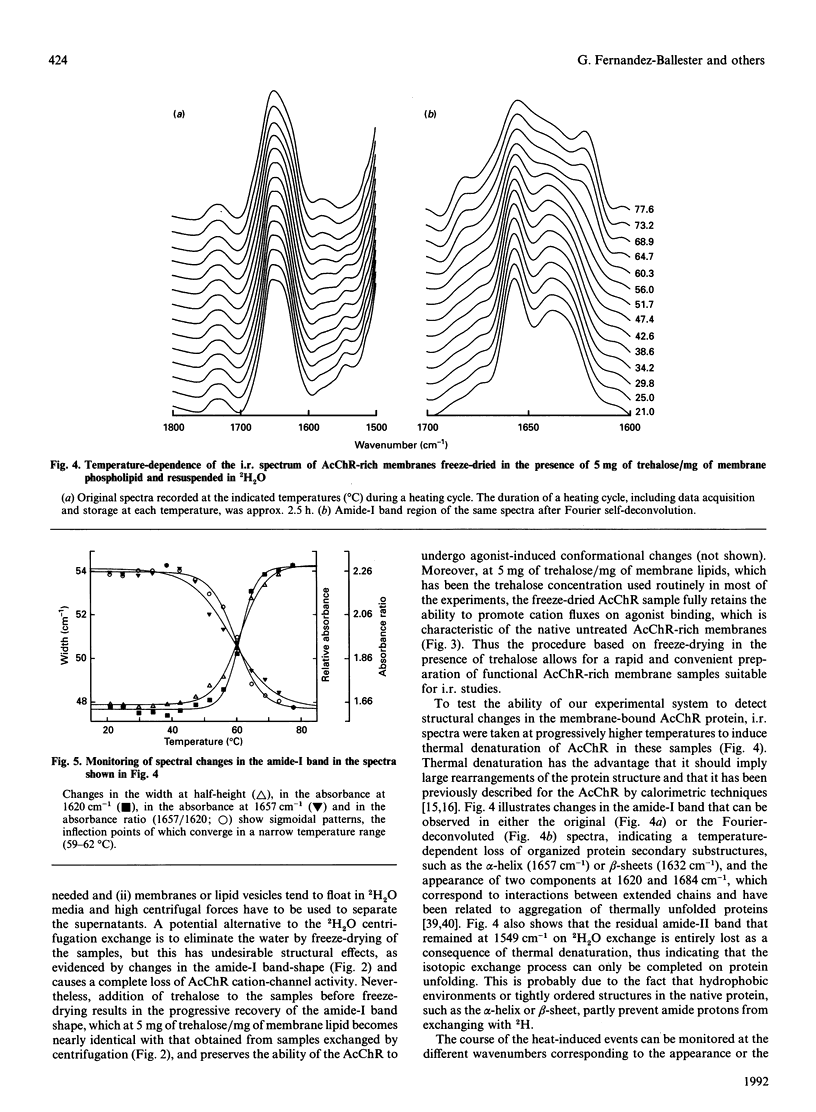
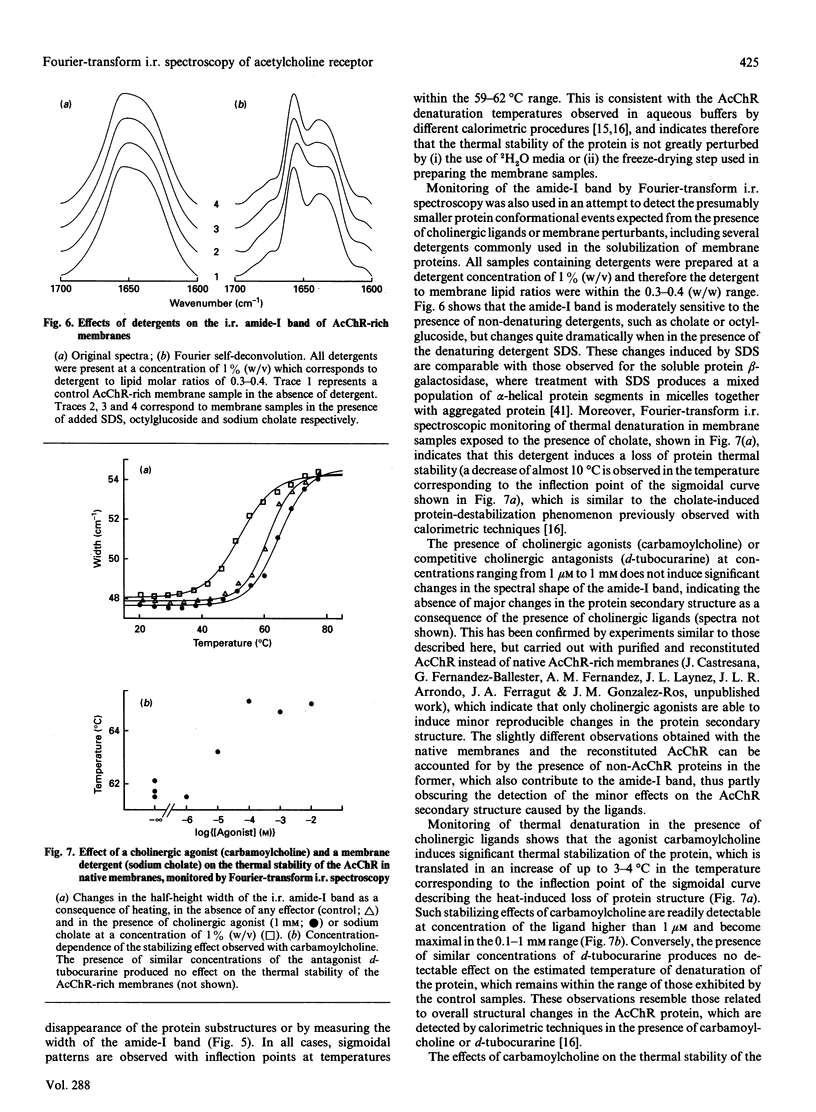
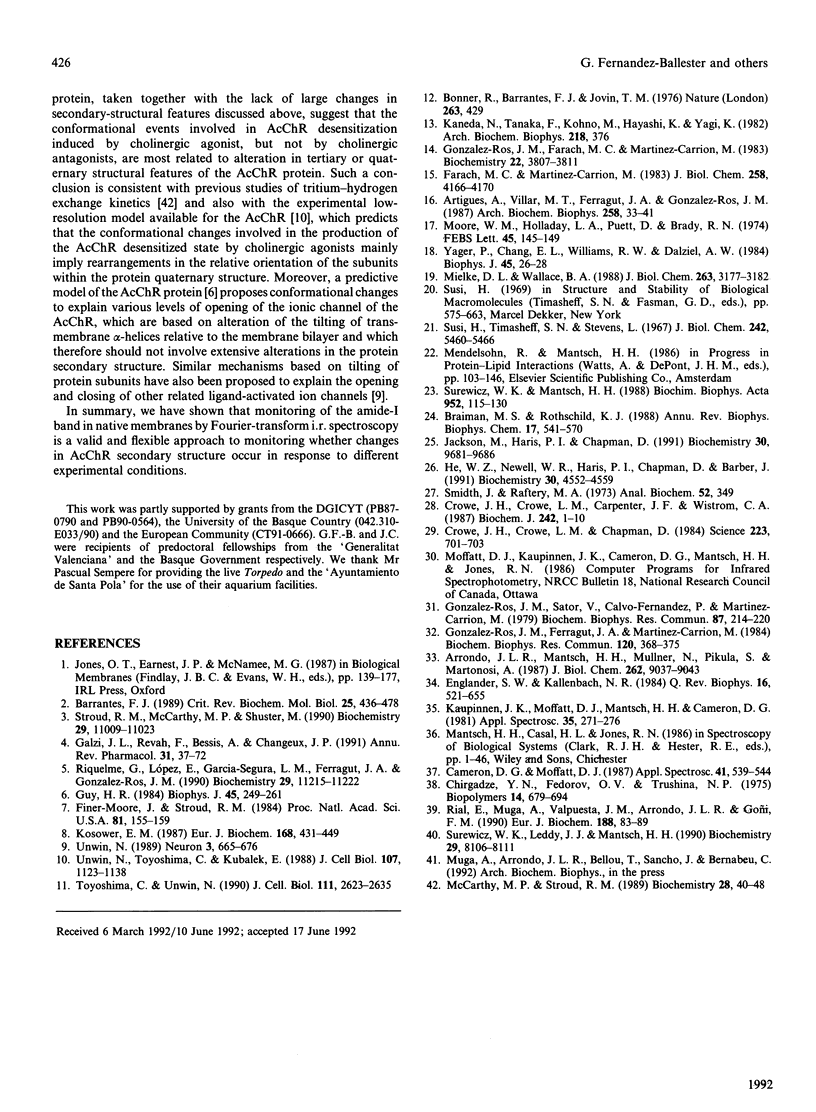
Selected References
These references are in PubMed. This may not be the complete list of references from this article.
- Arrondo J. L., Mantsch H. H., Mullner N., Pikula S., Martonosi A. Infrared spectroscopic characterization of the structural changes connected with the E1----E2 transition in the Ca2+-ATPase of sarcoplasmic reticulum. J Biol Chem. 1987 Jul 5;262(19):9037–9043. [PubMed] [Google Scholar]
- Artigues A., Villar M. T., Ferragut J. A., Gonzalez-Ros J. M. Thermal perturbation studies of membrane-bound acetylcholine receptor from Torpedo: effects of cholinergic ligands and membrane perturbants. Arch Biochem Biophys. 1987 Oct;258(1):33–41. doi: 10.1016/0003-9861(87)90319-5. [DOI] [PubMed] [Google Scholar]
- Barrantes F. J. The lipid environment of the nicotinic acetylcholine receptor in native and reconstituted membranes. Crit Rev Biochem Mol Biol. 1989;24(5):437–478. doi: 10.3109/10409238909086961. [DOI] [PubMed] [Google Scholar]
- Bonner R., Barrantes F. J., Jovin T. M. Kinetics of agonist-induced intrinsic fluorescence changes in membrane-bound acetylcholine receptor. Nature. 1976 Sep 30;263(5576):429–431. doi: 10.1038/263429a0. [DOI] [PubMed] [Google Scholar]
- Braiman M. S., Rothschild K. J. Fourier transform infrared techniques for probing membrane protein structure. Annu Rev Biophys Biophys Chem. 1988;17:541–570. doi: 10.1146/annurev.bb.17.060188.002545. [DOI] [PubMed] [Google Scholar]
- Chirgadze Y. N., Fedorov O. V., Trushina N. P. Estimation of amino acid residue side-chain absorption in the infrared spectra of protein solutions in heavy water. Biopolymers. 1975 Apr;14(4):679–694. doi: 10.1002/bip.1975.360140402. [DOI] [PubMed] [Google Scholar]
- Crowe J. H., Crowe L. M., Carpenter J. F., Aurell Wistrom C. Stabilization of dry phospholipid bilayers and proteins by sugars. Biochem J. 1987 Feb 15;242(1):1–10. doi: 10.1042/bj2420001. [DOI] [PMC free article] [PubMed] [Google Scholar]
- Crowe J. H., Crowe L. M., Chapman D. Preservation of membranes in anhydrobiotic organisms: the role of trehalose. Science. 1984 Feb 17;223(4637):701–703. doi: 10.1126/science.223.4637.701. [DOI] [PubMed] [Google Scholar]
- Englander S. W., Kallenbach N. R. Hydrogen exchange and structural dynamics of proteins and nucleic acids. Q Rev Biophys. 1983 Nov;16(4):521–655. doi: 10.1017/s0033583500005217. [DOI] [PubMed] [Google Scholar]
- Farach M. C., Martinez-Carrion M. A differential scanning calorimetry study of acetylcholine receptor-rich membranes from Torpedo californica. J Biol Chem. 1983 Apr 10;258(7):4166–4170. [PubMed] [Google Scholar]
- Finer-Moore J., Stroud R. M. Amphipathic analysis and possible formation of the ion channel in an acetylcholine receptor. Proc Natl Acad Sci U S A. 1984 Jan;81(1):155–159. doi: 10.1073/pnas.81.1.155. [DOI] [PMC free article] [PubMed] [Google Scholar]
- Galzi J. L., Revah F., Bessis A., Changeux J. P. Functional architecture of the nicotinic acetylcholine receptor: from electric organ to brain. Annu Rev Pharmacol Toxicol. 1991;31:37–72. doi: 10.1146/annurev.pa.31.040191.000345. [DOI] [PubMed] [Google Scholar]
- Gonzalez-Ros J. M., Farach M. C., Martinez-Carrion M. Ligand-induced effects at regions of acetylcholine receptor accessible to membrane lipids. Biochemistry. 1983 Aug 2;22(16):3807–3811. doi: 10.1021/bi00285a015. [DOI] [PubMed] [Google Scholar]
- Gonzalez-Ros J. M., Ferragut J. A., Martinez-Carrion M. Binding of anti-acetylcholine receptor antibodies inhibits the acetylcholine receptor mediated cation flux. Biochem Biophys Res Commun. 1984 Apr 30;120(2):368–375. doi: 10.1016/0006-291x(84)91263-4. [DOI] [PubMed] [Google Scholar]
- Gonzalez-Ros J. M., Sator V., Calvo-Fernandez P., Martinez-Carrion M. Pyrenesulfonyl azide: a covalent probe permitting in vitro desensitization of labeled acetylcholine-rich membrane fragments from Torpedo californica. Biochem Biophys Res Commun. 1979 Mar 15;87(1):214–220. doi: 10.1016/0006-291x(79)91668-1. [DOI] [PubMed] [Google Scholar]
- Guy H. R. A structural model of the acetylcholine receptor channel based on partition energy and helix packing calculations. Biophys J. 1984 Jan;45(1):249–261. doi: 10.1016/S0006-3495(84)84152-1. [DOI] [PMC free article] [PubMed] [Google Scholar]
- He W. Z., Newell W. R., Haris P. I., Chapman D., Barber J. Protein secondary structure of the isolated photosystem II reaction center and conformational changes studied by Fourier transform infrared spectroscopy. Biochemistry. 1991 May 7;30(18):4552–4559. doi: 10.1021/bi00232a027. [DOI] [PubMed] [Google Scholar]
- Jackson M., Haris P. I., Chapman D. Fourier transform infrared spectroscopic studies of Ca(2+)-binding proteins. Biochemistry. 1991 Oct 8;30(40):9681–9686. doi: 10.1021/bi00104a016. [DOI] [PubMed] [Google Scholar]
- Kaneda N., Tanaka F., Kohno M., Hayashi K., Yagi K. Change in the intrinsic fluorescence of acetylcholine receptor purified from Narke japonica upon binding with cholinergic ligands. Arch Biochem Biophys. 1982 Oct 15;218(2):376–383. doi: 10.1016/0003-9861(82)90359-9. [DOI] [PubMed] [Google Scholar]
- Kosower E. M. A structural and dynamic model for the nicotinic acetylcholine receptor. Eur J Biochem. 1987 Oct 15;168(2):431–449. doi: 10.1111/j.1432-1033.1987.tb13437.x. [DOI] [PubMed] [Google Scholar]
- McCarthy M. P., Stroud R. M. Conformational states of the nicotinic acetylcholine receptor from Torpedo californica induced by the binding of agonists, antagonists, and local anesthetics. Equilibrium measurements using tritium-hydrogen exchange. Biochemistry. 1989 Jan 10;28(1):40–48. doi: 10.1021/bi00427a007. [DOI] [PubMed] [Google Scholar]
- Mielke D. L., Wallace B. A. Secondary structural analyses of the nicotinic acetylcholine receptor as a test of molecular models. J Biol Chem. 1988 Mar 5;263(7):3177–3182. [PubMed] [Google Scholar]
- Moore W. M., Holladay L. A., Puett D., Brady R. N. On the conformation of the acetylcholine receptor protein from Torpedo nobiliana. FEBS Lett. 1974 Sep 1;45(1):145–149. doi: 10.1016/0014-5793(74)80832-x. [DOI] [PubMed] [Google Scholar]
- Rial E., Muga A., Valpuesta J. M., Arrondo J. L., Goñi F. M. Infrared spectroscopic studies of detergent-solubilized uncoupling protein from brown-adipose-tissue mitochondria. Eur J Biochem. 1990 Feb 22;188(1):83–89. doi: 10.1111/j.1432-1033.1990.tb15374.x. [DOI] [PubMed] [Google Scholar]
- Riquelme G., Lopez E., Garcia-Segura L. M., Ferragut J. A., Gonzalez-Ros J. M. Giant liposomes: a model system in which to obtain patch-clamp recordings of ionic channels. Biochemistry. 1990 Dec 25;29(51):11215–11222. doi: 10.1021/bi00503a009. [DOI] [PubMed] [Google Scholar]
- Schmidt J., Raftery M. A. A simple assay for the study of solubilized acetylcholine receptors. Anal Biochem. 1973 Apr;52(2):349–354. doi: 10.1016/0003-2697(73)90036-5. [DOI] [PubMed] [Google Scholar]
- Stroud R. M., McCarthy M. P., Shuster M. Nicotinic acetylcholine receptor superfamily of ligand-gated ion channels. Biochemistry. 1990 Dec 18;29(50):11009–11023. doi: 10.1021/bi00502a001. [DOI] [PubMed] [Google Scholar]
- Surewicz W. K., Leddy J. J., Mantsch H. H. Structure, stability, and receptor interaction of cholera toxin as studied by Fourier-transform infrared spectroscopy. Biochemistry. 1990 Sep 4;29(35):8106–8111. doi: 10.1021/bi00487a017. [DOI] [PubMed] [Google Scholar]
- Surewicz W. K., Mantsch H. H. New insight into protein secondary structure from resolution-enhanced infrared spectra. Biochim Biophys Acta. 1988 Jan 29;952(2):115–130. doi: 10.1016/0167-4838(88)90107-0. [DOI] [PubMed] [Google Scholar]
- Susi H., Timasheff S. N., Stevens L. Infrared spectra and protein conformations in aqueous solutions. I. The amide I band in H2O and D2O solutions. J Biol Chem. 1967 Dec 10;242(23):5460–5466. [PubMed] [Google Scholar]
- Toyoshima C., Unwin N. Three-dimensional structure of the acetylcholine receptor by cryoelectron microscopy and helical image reconstruction. J Cell Biol. 1990 Dec;111(6 Pt 1):2623–2635. doi: 10.1083/jcb.111.6.2623. [DOI] [PMC free article] [PubMed] [Google Scholar]
- Unwin N. The structure of ion channels in membranes of excitable cells. Neuron. 1989 Dec;3(6):665–676. doi: 10.1016/0896-6273(89)90235-3. [DOI] [PubMed] [Google Scholar]
- Unwin N., Toyoshima C., Kubalek E. Arrangement of the acetylcholine receptor subunits in the resting and desensitized states, determined by cryoelectron microscopy of crystallized Torpedo postsynaptic membranes. J Cell Biol. 1988 Sep;107(3):1123–1138. doi: 10.1083/jcb.107.3.1123. [DOI] [PMC free article] [PubMed] [Google Scholar]
- Yager P., Chang E. L., Williams R. W., Dalziel A. W. The secondary structure of acetylcholine receptor reconstituted in a single lipid component as determined by Raman spectroscopy. Biophys J. 1984 Jan;45(1):26–28. doi: 10.1016/S0006-3495(84)84095-3. [DOI] [PMC free article] [PubMed] [Google Scholar]