Abstract
1. An alkaline pH change occurred when L-rhamnose, L-mannose or L-lyxose was added to L-rhamnose-grown energy-depleted suspensions of strains of Escherichia coli. This is diagnostic of sugar-H+ symport activity. 2. L-Rhamnose, L-mannose and L-lyxose were inducers of the sugar-H+ symport and of L-[14C]rhamnose transport activity. L-Rhamnose also induced the biochemically and genetically distinct L-fucose-H+ symport activity in strains competent for L-rhamnose metabolism. 3. Steady-state kinetic measurements showed that L-mannose and L-lyxose were competitive inhibitors (alternative substrates) for the L-rhamnose transport system, and that L-galactose and D-arabinose were competitive inhibitors (alternative substrates) for the L-fucose transport system. Additional measurements with other sugars of related structure defined the different substrate specificities of the two transport systems. 4. The relative rates of H+ symport and of sugar metabolism, and the relative values of their kinetic parameters, suggested that the physiological role of the transport activity was primarily for utilization of L-rhamnose, not for L-mannose or L-lyxose. 5. L-Rhamnose transport into subcellular vesicles of E. coli was dependent on respiration, was optimal at pH 7, and was inhibited by protonophores and ionophores. It was insensitive to N-ethylmaleimide or cytochalasin B. 6. L-Rhamnose, L-mannose and L-lyxose each elicited an alkaline pH change when added to energy-depleted suspensions of L-rhamnose-grown Salmonella typhimurium LT2, Klebsiella pneumoniae, Klebsiella aerogenes, Erwinia carotovora carotovora and Erwinia carotovora atroseptica. The relative rates of subsequent acidification varied, depending on both the organism and the sugar. L-Fucose promoted an alkaline pH change in all the L-rhamnose-induced organisms except the Erwinia species. No L-rhamnose-H+ symport occurred in any organism grown on L-fucose. 7. All these results showed that L-rhamnose transport into the micro-organisms occurred by a system different from that for L-fucose transport. Both systems are energized by the trans-membrane electrochemical gradient of protons. 8. Neither steady-state kinetic measurements nor binding-protein assays revealed the existence of a second L-rhamnose transport system in E. coli.
Full text
PDF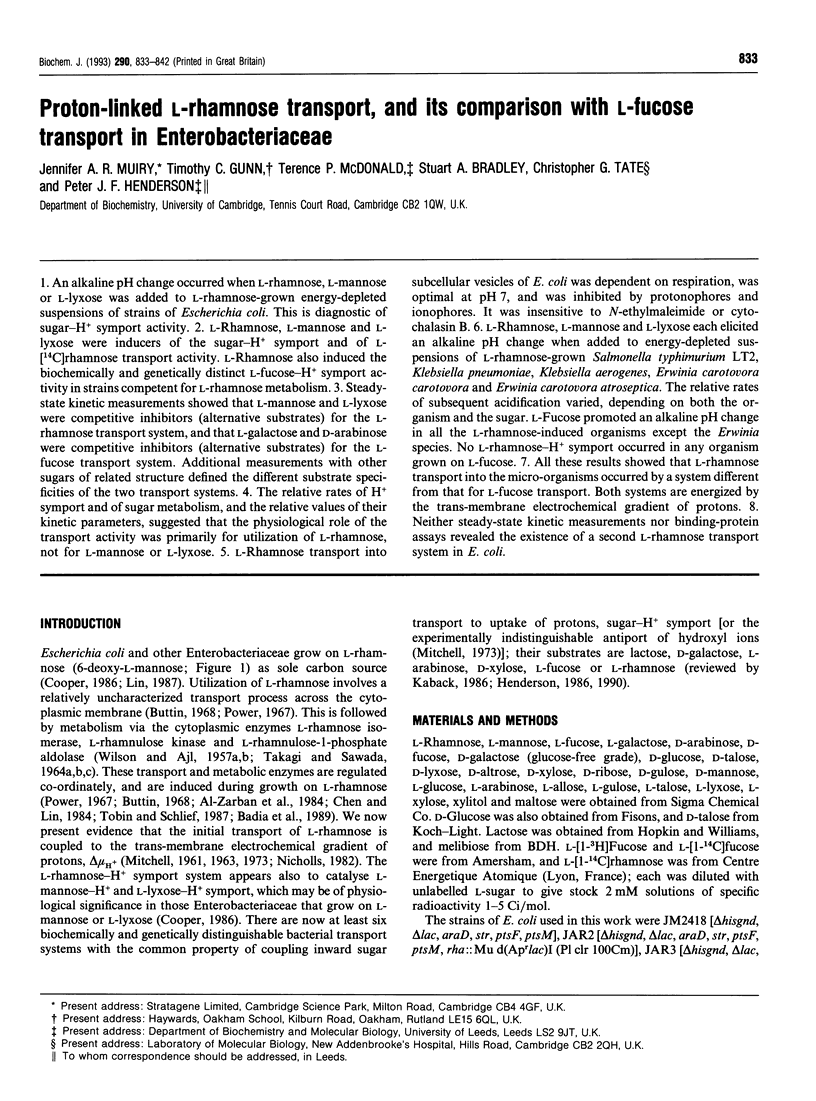
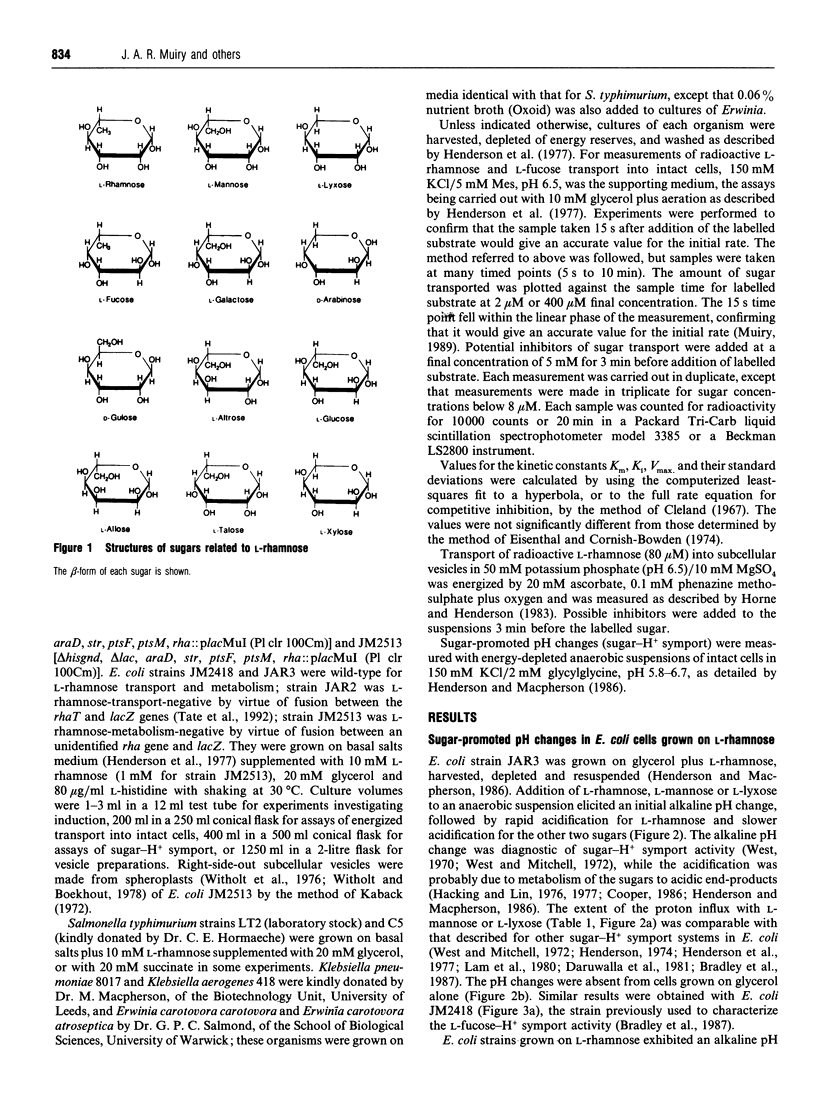
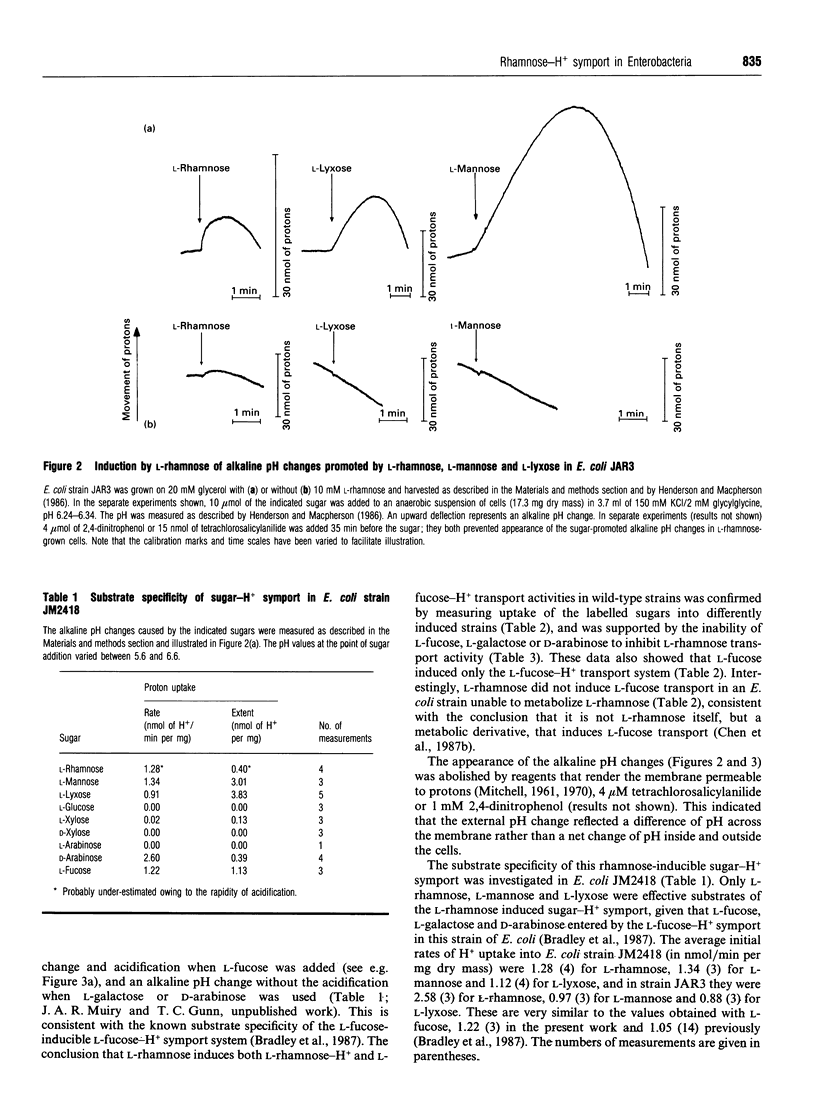
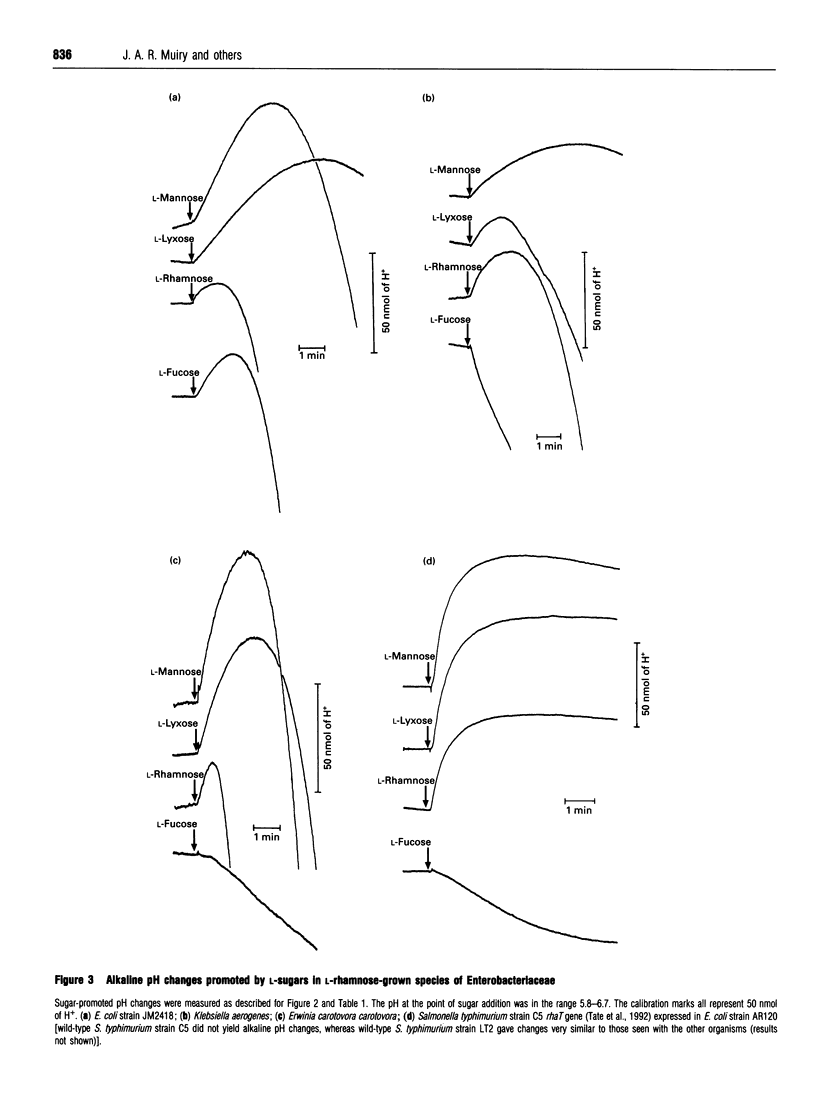
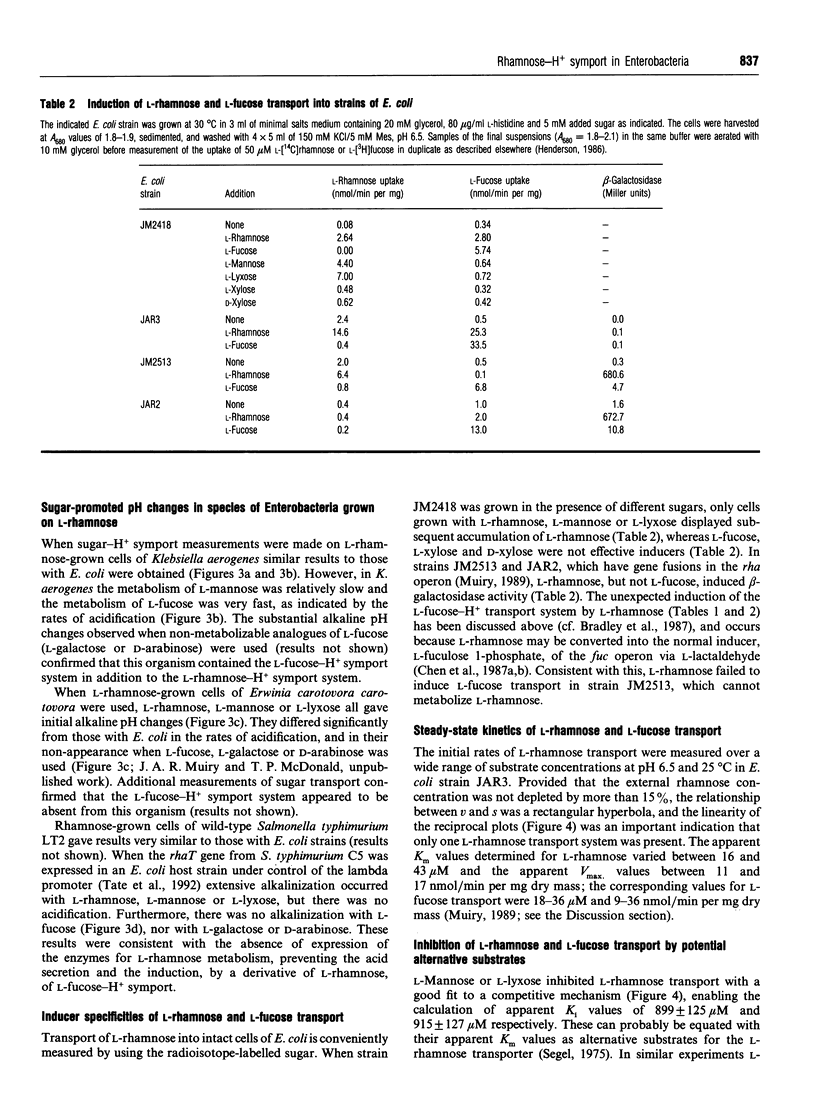
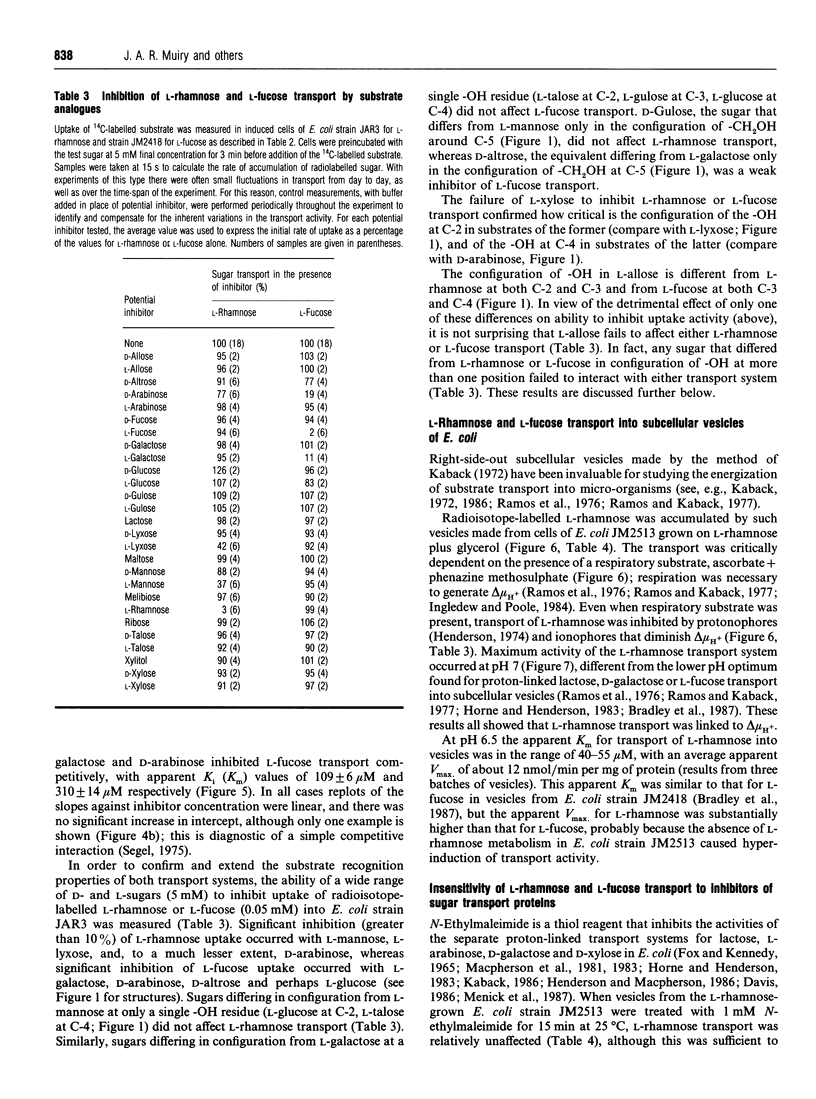
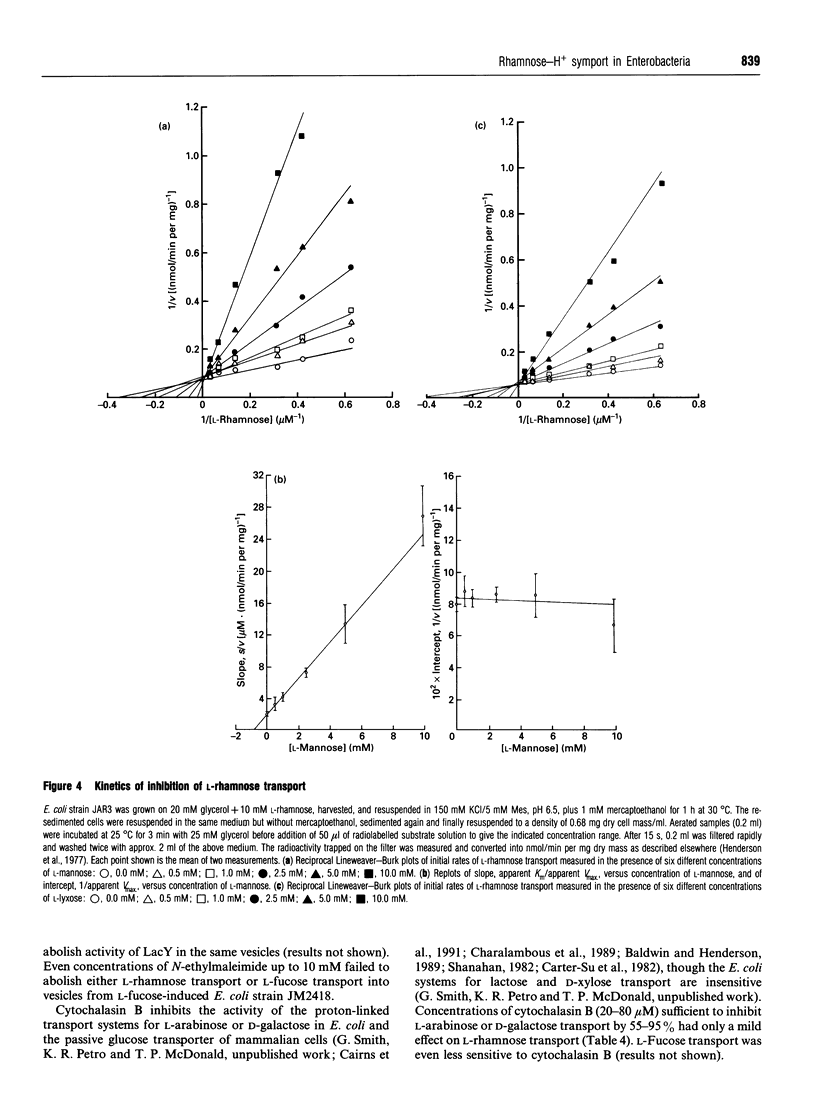
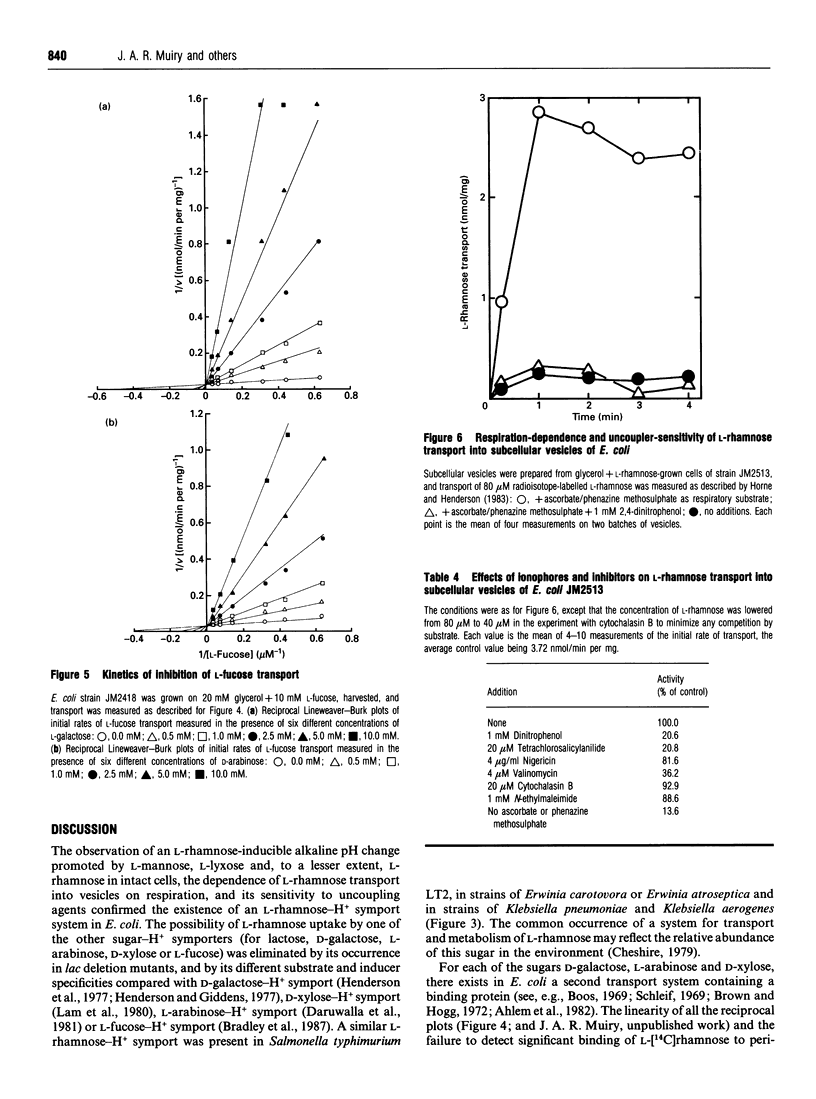
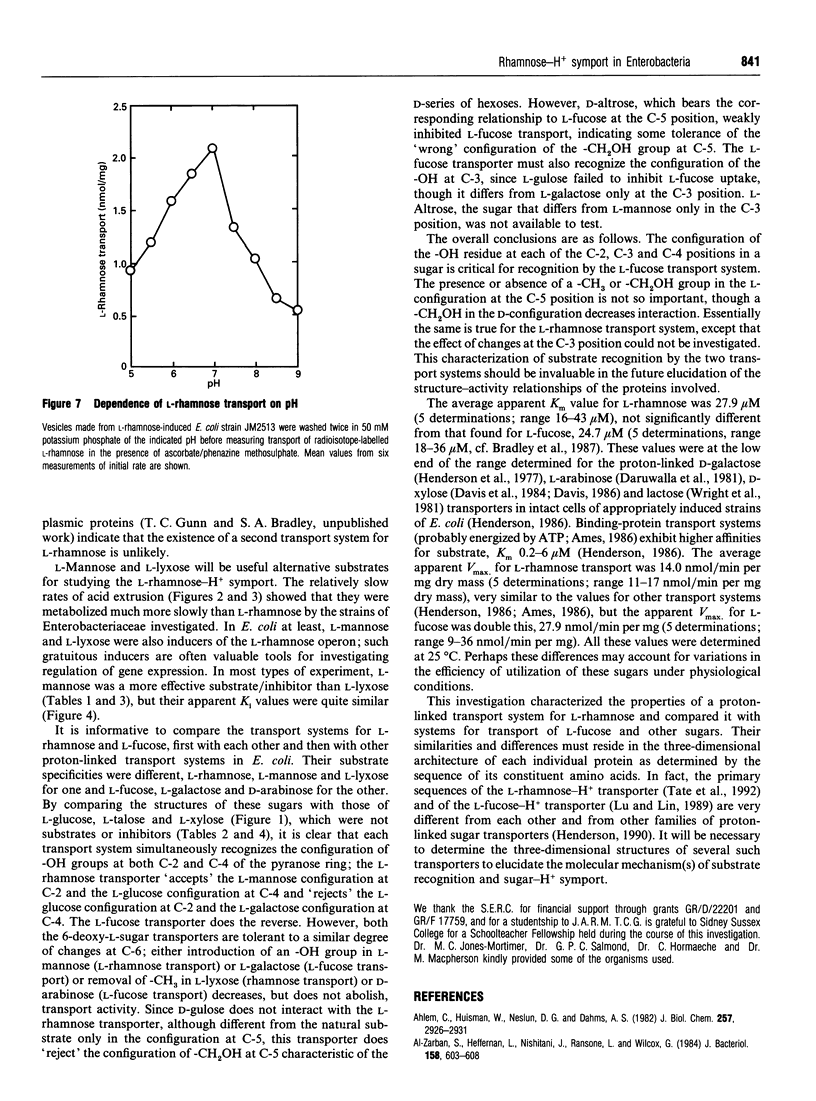
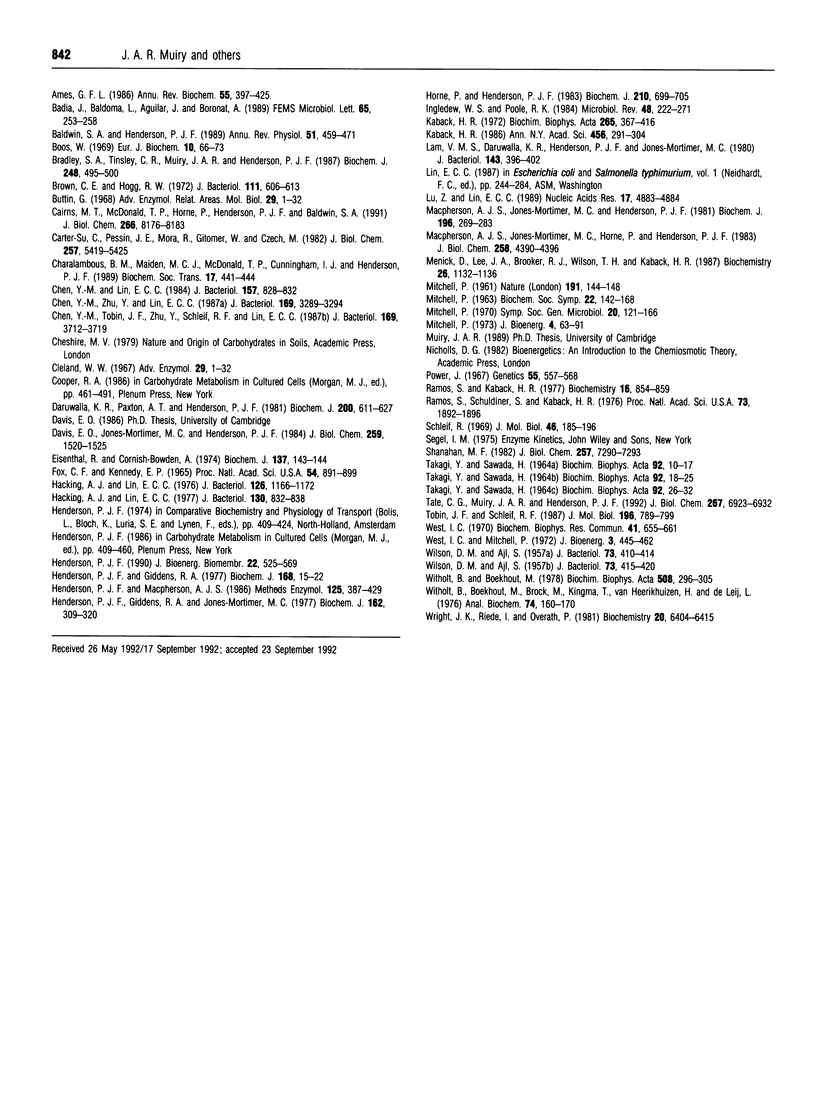
Selected References
These references are in PubMed. This may not be the complete list of references from this article.
- Ahlem C., Huisman W., Neslund G., Dahms A. S. Purification and properties of a periplasmic D-xylose-binding protein from Escherichia coli K-12. J Biol Chem. 1982 Mar 25;257(6):2926–2931. [PubMed] [Google Scholar]
- Al-Zarban S., Heffernan L., Nishitani J., Ransone L., Wilcox G. Positive control of the L-rhamnose genetic system in Salmonella typhimurium LT2. J Bacteriol. 1984 May;158(2):603–608. doi: 10.1128/jb.158.2.603-608.1984. [DOI] [PMC free article] [PubMed] [Google Scholar]
- Ames G. F. Bacterial periplasmic transport systems: structure, mechanism, and evolution. Annu Rev Biochem. 1986;55:397–425. doi: 10.1146/annurev.bi.55.070186.002145. [DOI] [PubMed] [Google Scholar]
- Badía J., Baldomà L., Aguilar J., Boronat A. Identification of the rhaA, rhaB and rhaD gene products from Escherichia coli K-12. FEMS Microbiol Lett. 1989 Dec;53(3):253–257. doi: 10.1016/0378-1097(89)90226-7. [DOI] [PubMed] [Google Scholar]
- Baldwin S. A., Henderson P. J. Homologies between sugar transporters from eukaryotes and prokaryotes. Annu Rev Physiol. 1989;51:459–471. doi: 10.1146/annurev.ph.51.030189.002331. [DOI] [PubMed] [Google Scholar]
- Boos W. The galactose binding protein and its relationship to the beta-methylgalactoside permease from Escherichia coli. Eur J Biochem. 1969 Aug;10(1):66–73. doi: 10.1111/j.1432-1033.1969.tb00656.x. [DOI] [PubMed] [Google Scholar]
- Bradley S. A., Tinsley C. R., Muiry J. A., Henderson P. J. Proton-linked L-fucose transport in Escherichia coli. Biochem J. 1987 Dec 1;248(2):495–500. doi: 10.1042/bj2480495. [DOI] [PMC free article] [PubMed] [Google Scholar]
- Brown C. E., Hogg R. W. A second transport system for L-arabinose in Escherichia coli B-r controlled by the araC gene. J Bacteriol. 1972 Aug;111(2):606–613. doi: 10.1128/jb.111.2.606-613.1972. [DOI] [PMC free article] [PubMed] [Google Scholar]
- Cairns M. T., McDonald T. P., Horne P., Henderson P. J., Baldwin S. A. Cytochalasin B as a probe of protein structure and substrate recognition by the galactose/H+ transporter of Escherichia coli. J Biol Chem. 1991 May 5;266(13):8176–8183. [PubMed] [Google Scholar]
- Carter-Su C., Pessin J. E., Mora R., Gitomer W., Czech M. P. Photoaffinity labeling of the human erythrocyte D-glucose transporter. J Biol Chem. 1982 May 25;257(10):5419–5425. [PubMed] [Google Scholar]
- Charalambous B. M., Maiden M. C., McDonald T. P., Cunningham I. J., Henderson P. J. Detection of proton-linked sugar transport proteins in Enterobacteriaceae. Biochem Soc Trans. 1989 Jun;17(3):441–444. doi: 10.1042/bst0170441a. [DOI] [PubMed] [Google Scholar]
- Chen Y. M., Lin E. C. Dual control of a common L-1,2-propanediol oxidoreductase by L-fucose and L-rhamnose in Escherichia coli. J Bacteriol. 1984 Mar;157(3):828–832. doi: 10.1128/jb.157.3.828-832.1984. [DOI] [PMC free article] [PubMed] [Google Scholar]
- Chen Y. M., Zhu Y., Lin E. C. NAD-linked aldehyde dehydrogenase for aerobic utilization of L-fucose and L-rhamnose by Escherichia coli. J Bacteriol. 1987 Jul;169(7):3289–3294. doi: 10.1128/jb.169.7.3289-3294.1987. [DOI] [PMC free article] [PubMed] [Google Scholar]
- Cleland W. W. The statistical analysis of enzyme kinetic data. Adv Enzymol Relat Areas Mol Biol. 1967;29:1–32. doi: 10.1002/9780470122747.ch1. [DOI] [PubMed] [Google Scholar]
- Cornish-Bowden A. A simple graphical method for determining the inhibition constants of mixed, uncompetitive and non-competitive inhibitors. Biochem J. 1974 Jan;137(1):143–144. doi: 10.1042/bj1370143. [DOI] [PMC free article] [PubMed] [Google Scholar]
- Daruwalla K. R., Paxton A. T., Henderson P. J. Energization of the transport systems for arabinose and comparison with galactose transport in Escherichia coli. Biochem J. 1981 Dec 15;200(3):611–627. doi: 10.1042/bj2000611. [DOI] [PMC free article] [PubMed] [Google Scholar]
- Davis E. O., Jones-Mortimer M. C., Henderson P. J. Location of a structural gene for xylose-H+ symport at 91 min on the linkage map of Escherichia coli K12. J Biol Chem. 1984 Feb 10;259(3):1520–1525. [PubMed] [Google Scholar]
- Fox C. F., Kennedy E. P. Specific labeling and partial purification of the M protein, a component of the beta-galactoside transport system of Escherichia coli. Proc Natl Acad Sci U S A. 1965 Sep;54(3):891–899. doi: 10.1073/pnas.54.3.891. [DOI] [PMC free article] [PubMed] [Google Scholar]
- Hacking A. J., Lin E. C. Disruption of the fucose pathway as a consequence of genetic adaptation to propanediol as a carbon source in Escherichia coli. J Bacteriol. 1976 Jun;126(3):1166–1172. doi: 10.1128/jb.126.3.1166-1172.1976. [DOI] [PMC free article] [PubMed] [Google Scholar]
- Hacking A. J., Lin E. C. Regulatory changes in the fucose system associated with the evolution of a catabolic pathway for propanediol in Escherichia coli. J Bacteriol. 1977 May;130(2):832–838. doi: 10.1128/jb.130.2.832-838.1977. [DOI] [PMC free article] [PubMed] [Google Scholar]
- Henderson P. J., Giddens R. A. 2-Deoxy-D-galactose, a substrate for the galactose-transport system of Escherichia coli. Biochem J. 1977 Oct 15;168(1):15–22. doi: 10.1042/bj1680015. [DOI] [PMC free article] [PubMed] [Google Scholar]
- Henderson P. J., Giddens R. A., Jones-Mortimer M. C. Transport of galactose, glucose and their molecular analogues by Escherichia coli K12. Biochem J. 1977 Feb 15;162(2):309–320. doi: 10.1042/bj1620309. [DOI] [PMC free article] [PubMed] [Google Scholar]
- Henderson P. J., Macpherson A. J. Assay, genetics, proteins, and reconstitution of proton-linked galactose, arabinose, and xylose transport systems of Escherichia coli. Methods Enzymol. 1986;125:387–429. doi: 10.1016/s0076-6879(86)25033-8. [DOI] [PubMed] [Google Scholar]
- Henderson P. J. Proton-linked sugar transport systems in bacteria. J Bioenerg Biomembr. 1990 Aug;22(4):525–569. doi: 10.1007/BF00762961. [DOI] [PubMed] [Google Scholar]
- Horne P., Henderson P. J. The association of proton movement with galactose transport into subcellular membrane vesicles of Escherichia coli. Biochem J. 1983 Mar 15;210(3):699–705. doi: 10.1042/bj2100699. [DOI] [PMC free article] [PubMed] [Google Scholar]
- Imae Y., Oosawa K., Mizuno T., Kihara M., Macnab R. M. Phenol: a complex chemoeffector in bacterial chemotaxis. J Bacteriol. 1987 Jan;169(1):371–379. doi: 10.1128/jb.169.1.371-379.1987. [DOI] [PMC free article] [PubMed] [Google Scholar]
- Ingledew W. J., Poole R. K. The respiratory chains of Escherichia coli. Microbiol Rev. 1984 Sep;48(3):222–271. doi: 10.1128/mr.48.3.222-271.1984. [DOI] [PMC free article] [PubMed] [Google Scholar]
- Kaback H. R. Proton electrochemical gradients and active transport: the saga of lac permease. Ann N Y Acad Sci. 1985;456:291–304. doi: 10.1111/j.1749-6632.1985.tb14879.x. [DOI] [PubMed] [Google Scholar]
- Kaback H. R. Transport across isolated bacterial cytoplasmic membranes. Biochim Biophys Acta. 1972 Aug 4;265(3):367–416. doi: 10.1016/0304-4157(72)90014-7. [DOI] [PubMed] [Google Scholar]
- Lam V. M., Daruwalla K. R., Henderson P. J., Jones-Mortimer M. C. Proton-linked D-xylose transport in Escherichia coli. J Bacteriol. 1980 Jul;143(1):396–402. doi: 10.1128/jb.143.1.396-402.1980. [DOI] [PMC free article] [PubMed] [Google Scholar]
- Lu Z., Lin E. C. The nucleotide sequence of Escherichia coli genes for L-fucose dissimilation. Nucleic Acids Res. 1989 Jun 26;17(12):4883–4884. doi: 10.1093/nar/17.12.4883. [DOI] [PMC free article] [PubMed] [Google Scholar]
- MITCHELL P. Coupling of phosphorylation to electron and hydrogen transfer by a chemi-osmotic type of mechanism. Nature. 1961 Jul 8;191:144–148. doi: 10.1038/191144a0. [DOI] [PubMed] [Google Scholar]
- MacPherson A. J., Jones-Mortimer M. C., Henderson P. J. Identification of the AraE transport protein of Escherichia coli. Biochem J. 1981 Apr 15;196(1):269–283. doi: 10.1042/bj1960269. [DOI] [PMC free article] [PubMed] [Google Scholar]
- Macpherson A. J., Jones-Mortimer M. C., Horne P., Henderson P. J. Identification of the GalP galactose transport protein of Escherichia coli. J Biol Chem. 1983 Apr 10;258(7):4390–4396. [PubMed] [Google Scholar]
- Menick D. R., Lee J. A., Brooker R. J., Wilson T. H., Kaback H. R. Role of cysteine residues in the lac permease of Escherichia coli. Biochemistry. 1987 Feb 24;26(4):1132–1136. doi: 10.1021/bi00378a022. [DOI] [PubMed] [Google Scholar]
- Mitchell P. Performance and conservation of osmotic work by proton-coupled solute porter systems. J Bioenerg. 1973 Jan;4(1):63–91. doi: 10.1007/BF01516051. [DOI] [PubMed] [Google Scholar]
- Power J. The L-rhamnose genetic system in Escherichia coli K-12. Genetics. 1967 Mar;55(3):557–568. doi: 10.1093/genetics/55.3.557. [DOI] [PMC free article] [PubMed] [Google Scholar]
- Ramos S., Kaback H. R. The relationship between the electrochemical proton gradient and active transport in Escherichia coli membrane vesicles. Biochemistry. 1977 Mar 8;16(5):854–859. doi: 10.1021/bi00624a007. [DOI] [PubMed] [Google Scholar]
- Ramos S., Schuldiner S., Kaback H. R. The electrochemical gradient of protons and its relationship to active transport in Escherichia coli membrane vesicles. Proc Natl Acad Sci U S A. 1976 Jun;73(6):1892–1896. doi: 10.1073/pnas.73.6.1892. [DOI] [PMC free article] [PubMed] [Google Scholar]
- SAWADA H., TAKAGI Y. THE METABOLISM OF L-RHAMNOSE IN ESCHERICHIA COLI. 3. L-RHAMULOSE-PHOSPHATE ALDOLASE. Biochim Biophys Acta. 1964 Oct 23;92:26–32. doi: 10.1016/0926-6569(64)90265-2. [DOI] [PubMed] [Google Scholar]
- Schleif R. An L-arabinose binding protein and arabinose permeation in Escherichia coli. J Mol Biol. 1969 Nov 28;46(1):185–196. doi: 10.1016/0022-2836(69)90065-5. [DOI] [PubMed] [Google Scholar]
- Shanahan M. F. Cytochalasin B. A natural photoaffinity ligand for labeling the human erythrocyte glucose transporter. J Biol Chem. 1982 Jul 10;257(13):7290–7293. [PubMed] [Google Scholar]
- TAKAGI Y., SAWADA H. THE METABOLISM OF L-RHAMNOSE IN ESCHERICHIA COLI. I. L-RHAMNOSE ISOMERASE. Biochim Biophys Acta. 1964 Oct 23;92:10–17. doi: 10.1016/0926-6569(64)90263-9. [DOI] [PubMed] [Google Scholar]
- TAKAGI Y., SAWADA H. THE METABOLISM OF L-RHAMNOSE IN ESCHERICHIA COLI. II. L-RHAMNULOSE KINASE. Biochim Biophys Acta. 1964 Oct 23;92:18–25. doi: 10.1016/0926-6569(64)90264-0. [DOI] [PubMed] [Google Scholar]
- Tate C. G., Muiry J. A., Henderson P. J. Mapping, cloning, expression, and sequencing of the rhaT gene, which encodes a novel L-rhamnose-H+ transport protein in Salmonella typhimurium and Escherichia coli. J Biol Chem. 1992 Apr 5;267(10):6923–6932. [PubMed] [Google Scholar]
- Tobin J. F., Schleif R. F. Positive regulation of the Escherichia coli L-rhamnose operon is mediated by the products of tandemly repeated regulatory genes. J Mol Biol. 1987 Aug 20;196(4):789–799. doi: 10.1016/0022-2836(87)90405-0. [DOI] [PubMed] [Google Scholar]
- WILSON D. M., AJL S. Metabolism of L-rhamnose by Escherichia coli. I. L-rhamnose isomerase. J Bacteriol. 1957 Mar;73(3):410–414. doi: 10.1128/jb.73.3.410-414.1957. [DOI] [PMC free article] [PubMed] [Google Scholar]
- WILSON D. M., AJL S. Metabolism of L-rhamnose by Escherichia coli. II. The phosphorylation of L-rhamnulose. J Bacteriol. 1957 Mar;73(3):415–420. doi: 10.1128/jb.73.3.415-420.1957. [DOI] [PMC free article] [PubMed] [Google Scholar]
- West I. C. Lactose transport coupled to proton movements in Escherichia coli. Biochem Biophys Res Commun. 1970 Nov 9;41(3):655–661. doi: 10.1016/0006-291x(70)90063-x. [DOI] [PubMed] [Google Scholar]
- West I., Mitchell P. Proton-coupled beta-galactoside translocation in non-metabolizing Escherichia coli. J Bioenerg. 1972 Aug;3(5):445–462. doi: 10.1007/BF01516082. [DOI] [PubMed] [Google Scholar]
- Witholt B., Boekhout M. The effect of osmotic shock on the accessibility of the murein layer of exponentially growing Escherichia coli to lysozyme. Biochim Biophys Acta. 1978 Apr 4;508(2):296–305. doi: 10.1016/0005-2736(78)90332-2. [DOI] [PubMed] [Google Scholar]
- Wright J. K., Riede I., Overath P. Lactose carrier protein of Escherichia coli: interaction with galactosides and protons. Biochemistry. 1981 Oct 27;20(22):6404–6415. doi: 10.1021/bi00525a019. [DOI] [PubMed] [Google Scholar]