Abstract
1. A new thiol-specific reactivity probe 4,4'-dipyrimidyl disulphide [compound (VII), m.p. 110 degrees C, pKa of its monohydronated form 0.91] was synthesized and used to resolve the ambiguity of interpretation of the behaviour of papain (EC 3.4.22.2) in alkaline media known to depend to varying extents on two ionizations with pKa values approx. 8.0-8.5 and > or = 9.5 respectively. 2. A new extensive pH-second-order rate constant (k) data set for the reaction of papain with 2-(acetamido)-ethyl 2'-pyridyl disulphide (IV) demonstrated the existence of a striking rate maximum at pH approx. 4, the independence of k around pH 8 and the increase in k with increase in pH across a pKa value of 10.0, behaviour similar to that of other 2-pyridyl disulphides (R-S-S-2-Py) that lack key substrate-like binding sites in R. 3. Although the simplest interpretation of the pKa value of 10.0 assigns it to the formation of (Cys-25)-S-/(His-159)-Im from the ion-pair state of the papain catalytic site, another interpretation may be conceived in which this pKa value is assigned to another group remote from the catalytic site, the state of ionization of which modulates catalytic-site behaviour. This alternative assignment is shown to require compensating effects in the pH region around 8 such that the formation of (Cys-25)-S-/(His-159)-Im across pKa 8.0-8.5 is without net kinetic effect in the reactions of simple 2-pyridyl disulphides such as compound (IV) and 2,2'-dipyridyl disulphide (II). 4. The lower basicity of compound (VII) relative to that of compound (II) (pKa 2.45) was predicted to diminish or abolish the compensation postulated as a possibility in reactions of 2-pyridyl disulphides because of the decreased effectiveness of reaction via a (His-159)-Im+H-assisted transition state. The characteristics of the pH-dependence of the reaction of papain with compound (VII) which are quite different from those for its reaction with compound (II) support both this prediction and the alternative assignment with a value of 8.3 for the pKa of the formation of (Cys-25)-S-/(His-159)-Im. 5. Evidence that the behaviour of papain towards both substrates and some substrate-derived time-dependent inhibitors is determined not only by the loss of the (Cys-25)-S-/(His-159)-Im+H ion-pair state by dehydronation with pKa 8.3 but also by another ionization of pKa approx. 10.0 is briefly discussed.
Full text
PDF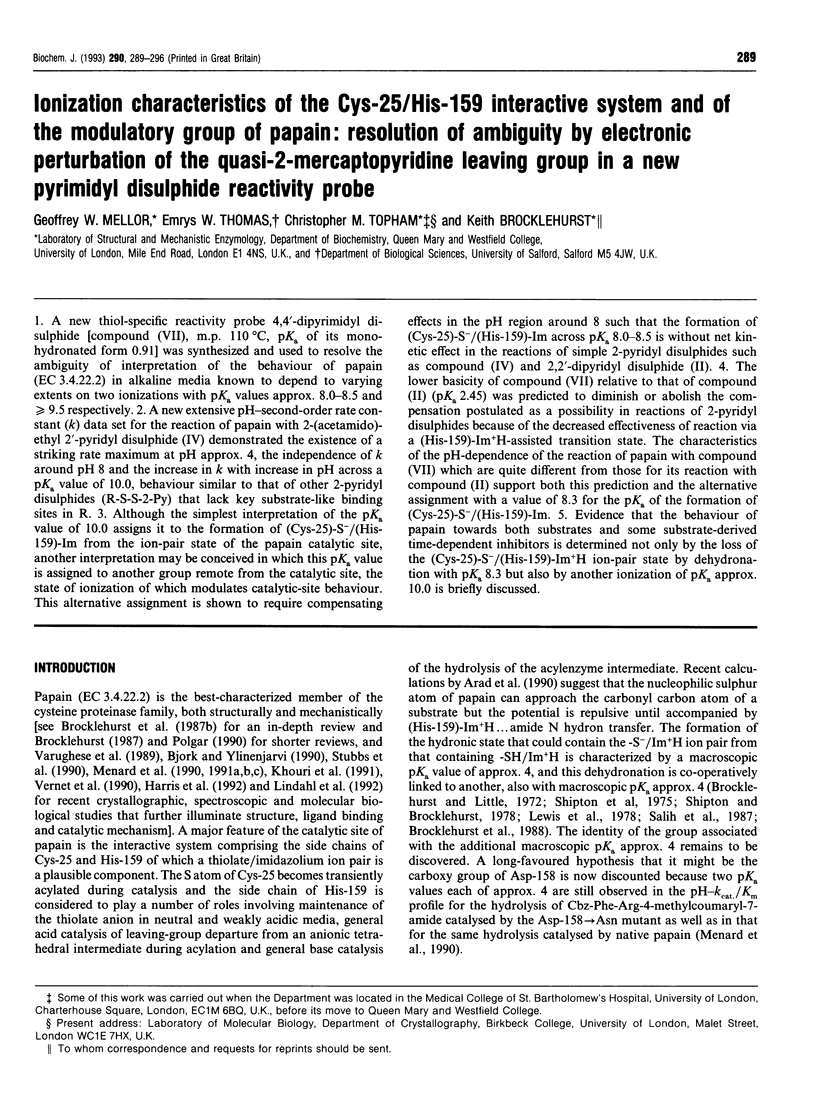
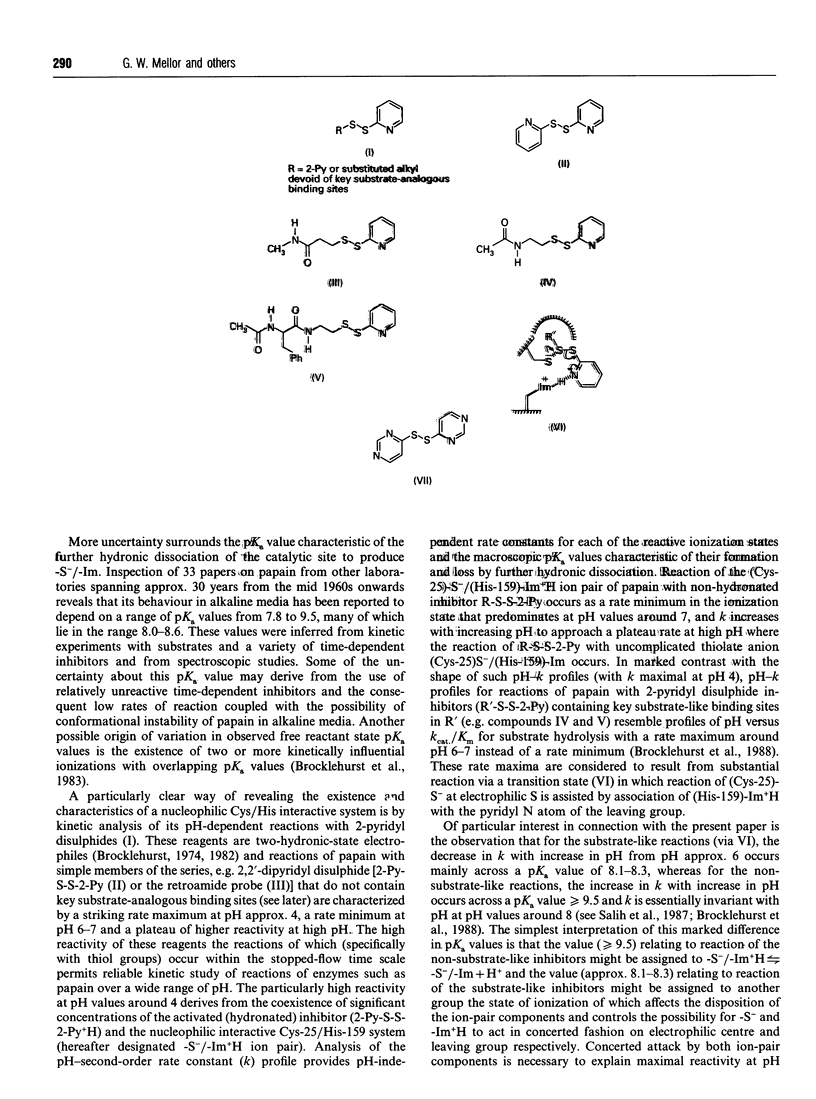
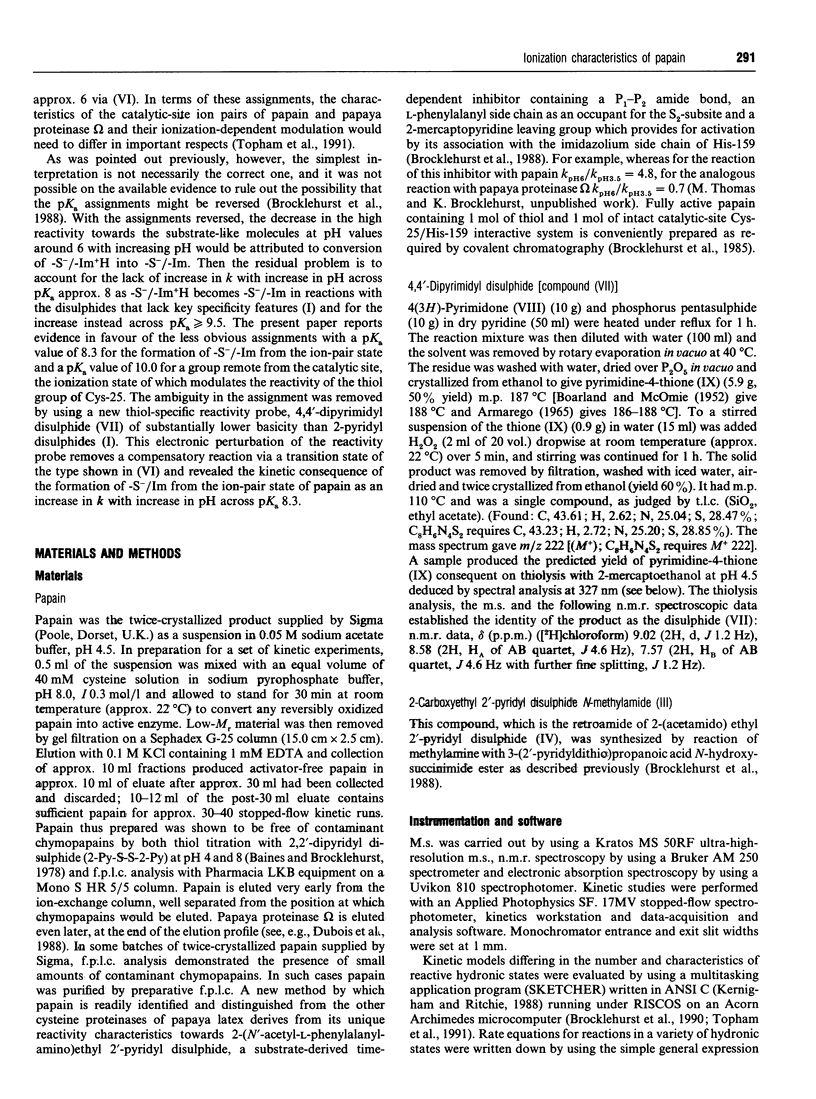
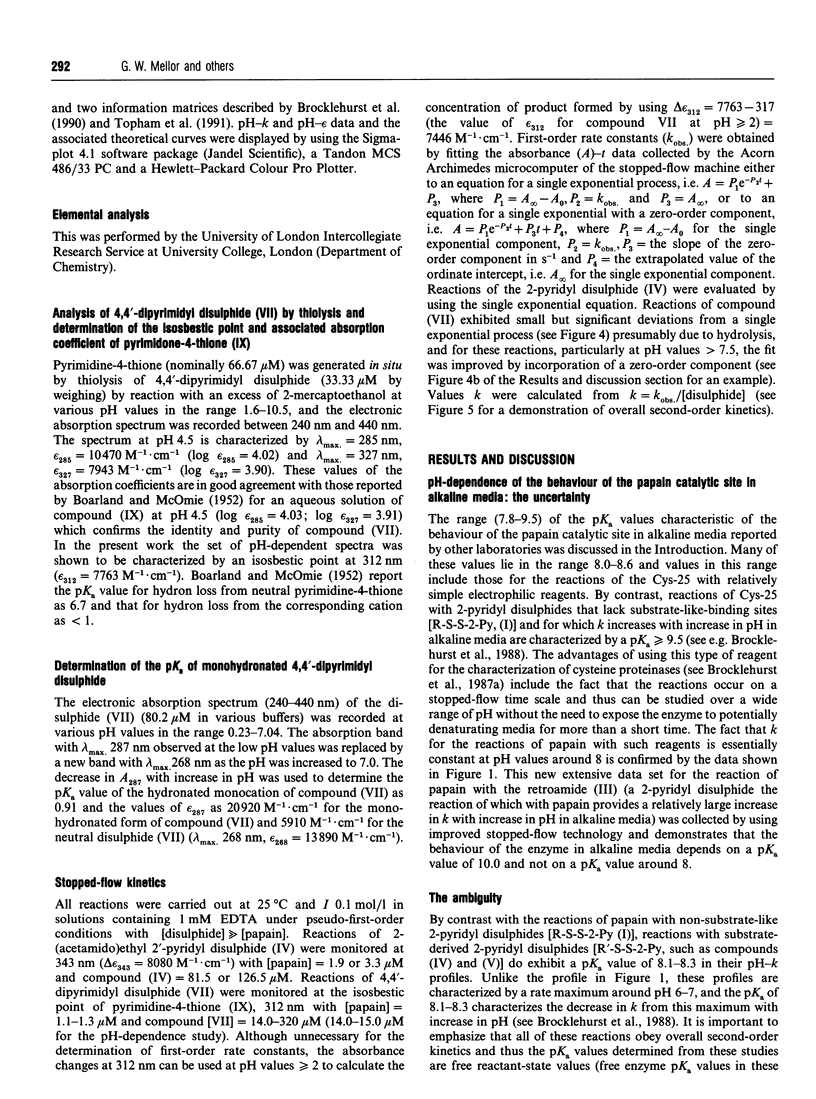
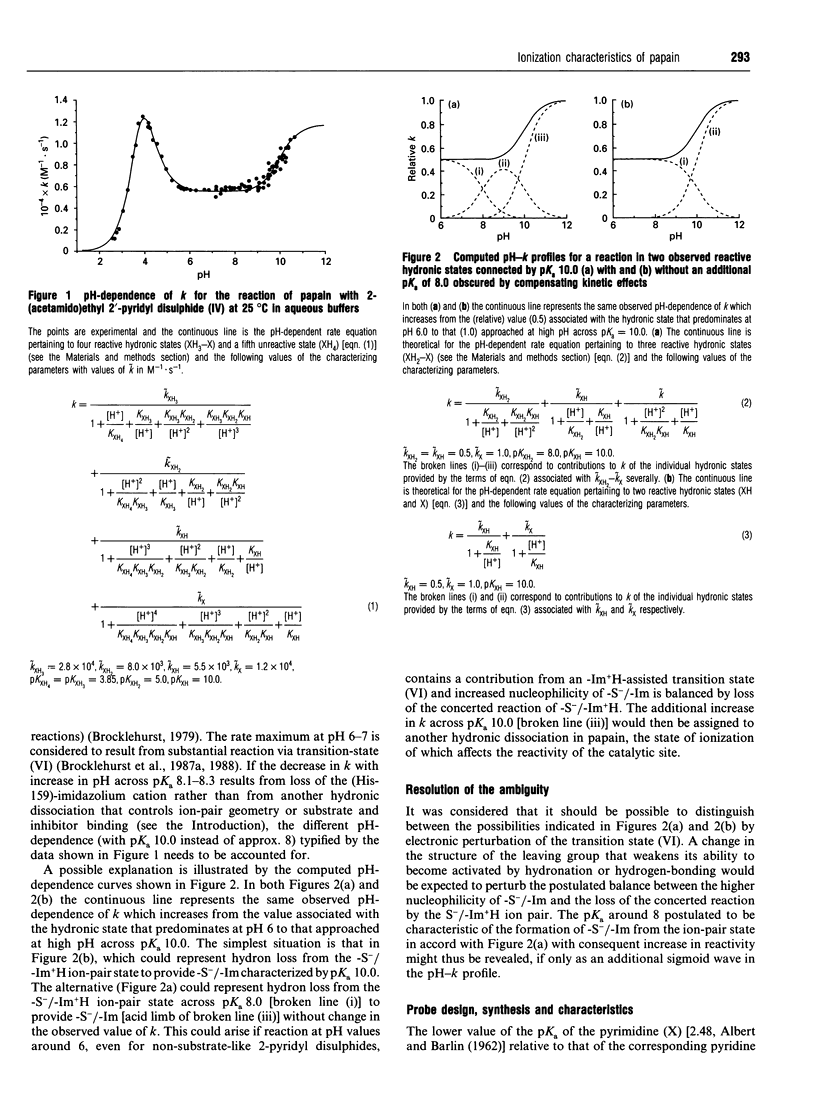
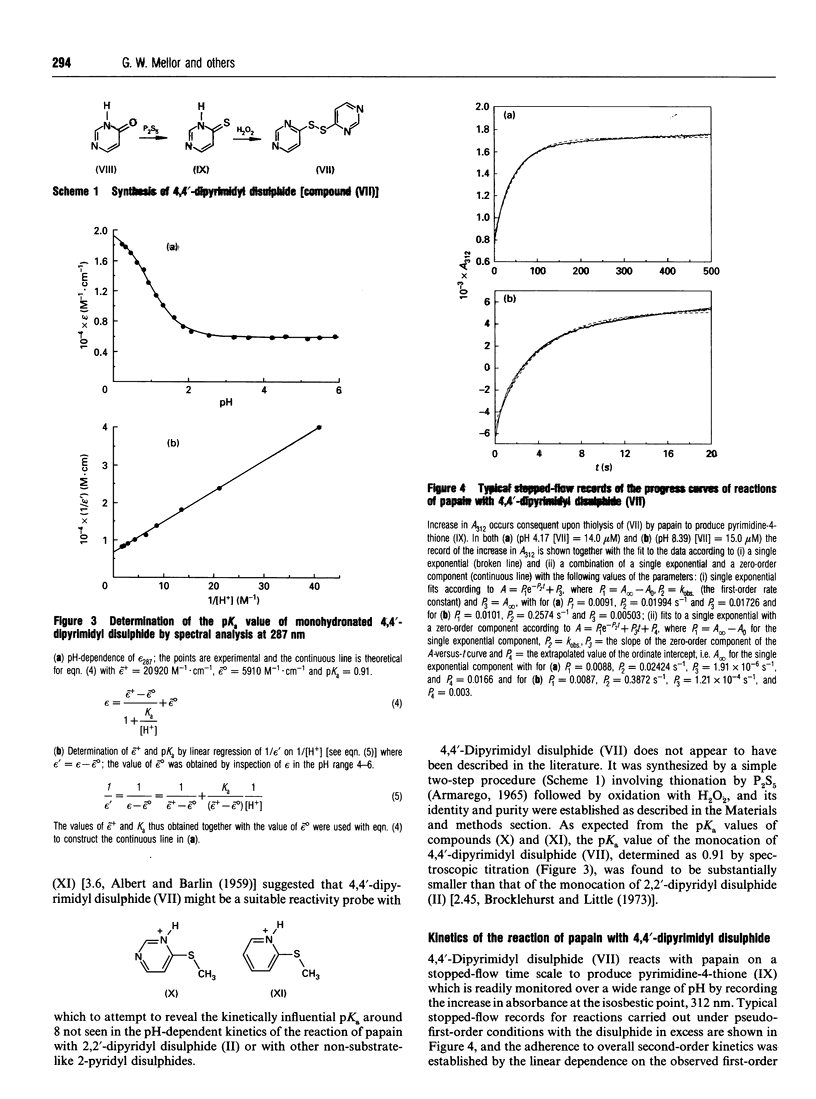
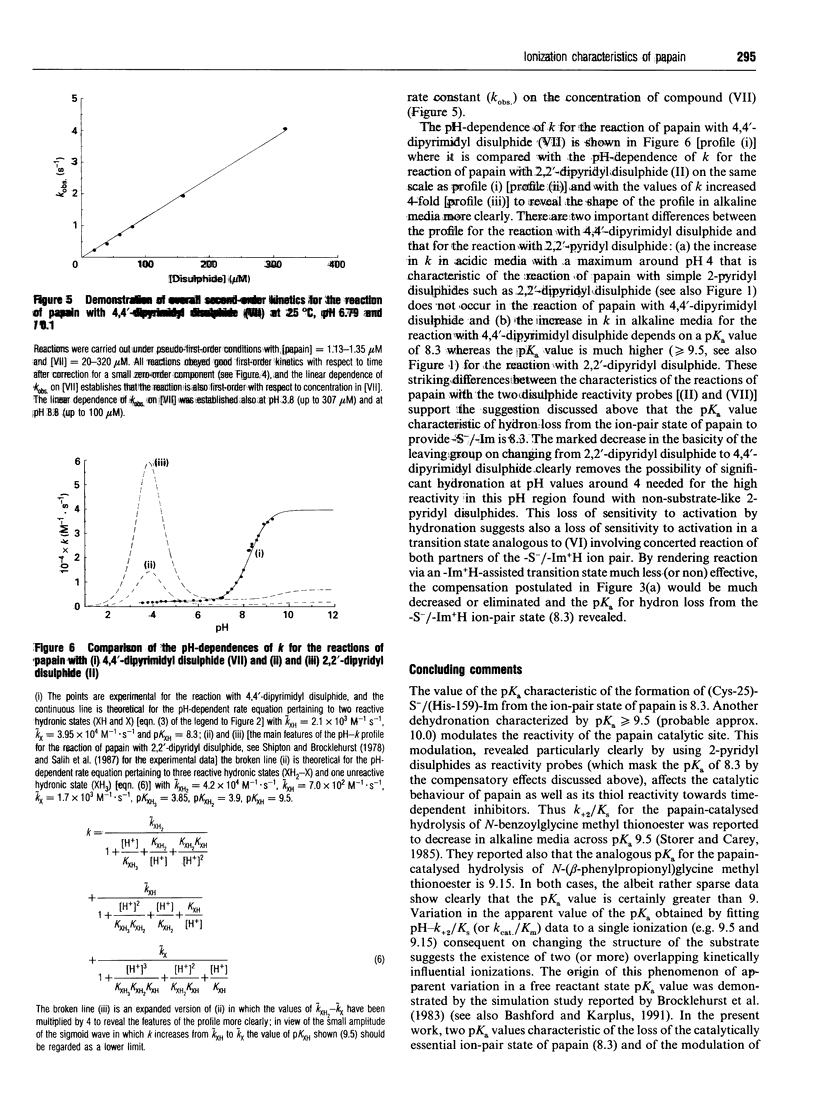
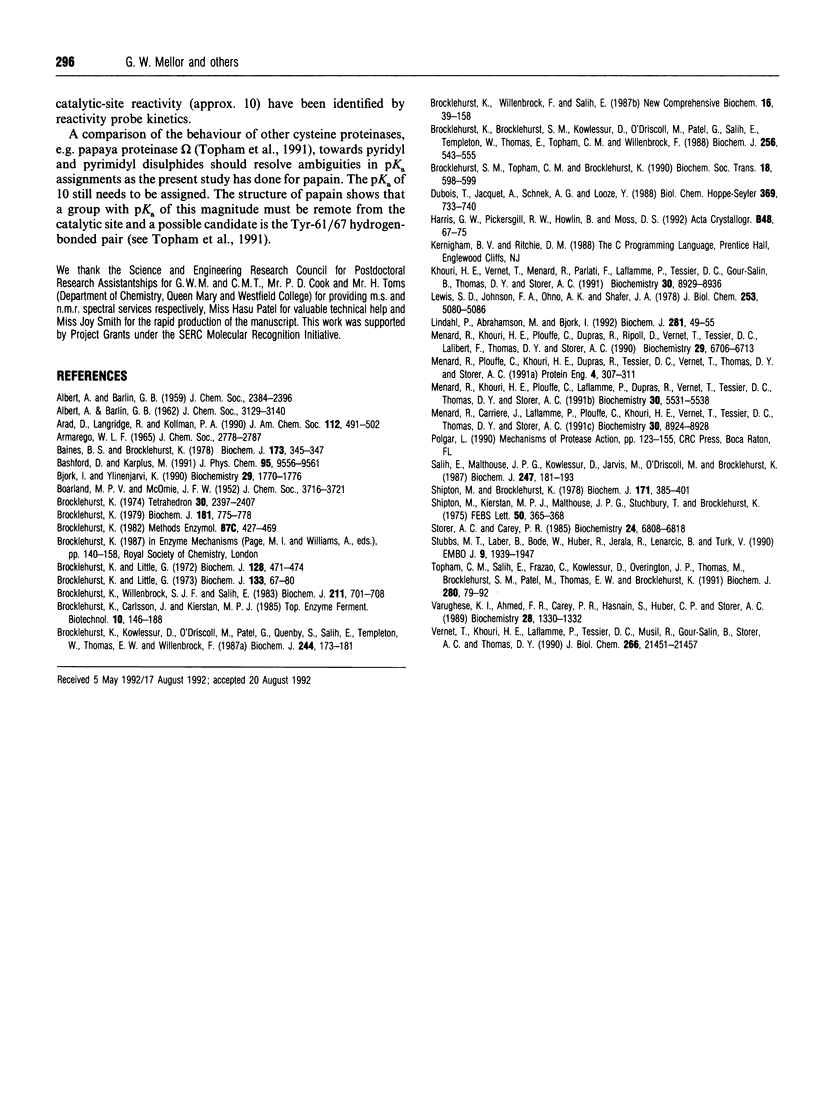
Selected References
These references are in PubMed. This may not be the complete list of references from this article.
- Baines B. S., Brocklehurst K. A spectrophotometric method for the detection of contaminant chymopapains in preparations of papain. Selective modification of one type of thiol group in the chymopapains by a two-protonic-state reagent. Biochem J. 1978 Jul 1;173(1):345–347. doi: 10.1042/bj1730345. [DOI] [PMC free article] [PubMed] [Google Scholar]
- Björk I., Ylinenjärvi K. Interaction between chicken cystatin and the cysteine proteinases actinidin, chymopapain A, and ficin. Biochemistry. 1990 Feb 20;29(7):1770–1776. doi: 10.1021/bi00459a016. [DOI] [PubMed] [Google Scholar]
- Brocklehurst K., Brocklehurst S. M., Kowlessur D., O'Driscoll M., Patel G., Salih E., Templeton W., Thomas E., Topham C. M., Willenbrock F. Supracrystallographic resolution of interactions contributing to enzyme catalysis by use of natural structural variants and reactivity-probe kinetics. Biochem J. 1988 Dec 1;256(2):543–558. doi: 10.1042/bj2560543. [DOI] [PMC free article] [PubMed] [Google Scholar]
- Brocklehurst K., Kowlessur D., O'Driscoll M., Patel G., Quenby S., Salih E., Templeton W., Thomas E. W., Willenbrock F. Substrate-derived two-protonic-state electrophiles as sensitive kinetic specificity probes for cysteine proteinases. Activation of 2-pyridyl disulphides by hydrogen-bonding. Biochem J. 1987 May 15;244(1):173–181. doi: 10.1042/bj2440173. [DOI] [PMC free article] [PubMed] [Google Scholar]
- Brocklehurst K., Little G. Reactions of papain and of low-molecular-weight thiols with some aromatic disulphides. 2,2'-Dipyridyl disulphide as a convenient active-site titrant for papain even in the presence of other thiols. Biochem J. 1973 May;133(1):67–80. doi: 10.1042/bj1330067. [DOI] [PMC free article] [PubMed] [Google Scholar]
- Brocklehurst K., Little G. Reactivities of the various protonic states in the reactions of papain and of L-cysteine with 2,2'- and with 4,4'- dipyridyl disulphide: evidence for nucleophilic reactivity in the un-ionized thiol group of the cysteine-25 residue of papain occasioned by its interaction with the histidine-159-asparagine-175 hydrogen-bonded system. Biochem J. 1972 Jun;128(2):471–474. doi: 10.1042/bj1280471. [DOI] [PMC free article] [PubMed] [Google Scholar]
- Brocklehurst K. The equilibrium assumption is valid for the kinetic treatment of most time-dependent protein-modification reactions. Biochem J. 1979 Sep 1;181(3):775–778. doi: 10.1042/bj1810775. [DOI] [PMC free article] [PubMed] [Google Scholar]
- Brocklehurst K. Two-protonic-state electrophiles as probes of enzyme mechanisms. Methods Enzymol. 1982;87:427–469. doi: 10.1016/s0076-6879(82)87026-2. [DOI] [PubMed] [Google Scholar]
- Brocklehurst K., Willenbrock S. J., Salih E. Effects of conformational selectivity and of overlapping kinetically influential ionizations on the characteristics of pH-dependent enzyme kinetics. Implications of free-enzyme pKa variability in reactions of papain for its catalytic mechanism. Biochem J. 1983 Jun 1;211(3):701–708. doi: 10.1042/bj2110701. [DOI] [PMC free article] [PubMed] [Google Scholar]
- Brocklehurst S. M., Topham C. M., Brocklehurst K. A general kinetic equation for multihydronic state reactions and rapid procedures for parameter evaluation. Biochem Soc Trans. 1990 Aug;18(4):598–600. doi: 10.1042/bst0180598. [DOI] [PubMed] [Google Scholar]
- Dubois T., Jacquet A., Schnek A. G., Looze Y. The thiol proteinases from the latex of Carica papaya L. I. Fractionation, purification and preliminary characterization. Biol Chem Hoppe Seyler. 1988 Aug;369(8):733–740. doi: 10.1515/bchm3.1988.369.2.733. [DOI] [PubMed] [Google Scholar]
- Harris G. W., Pickersgill R. W., Howlin B., Moss D. S. The segmented anisotropic refinement of monoclinic papain by the application of the rigid-body TLS model and comparison to bovine ribonuclease A. Acta Crystallogr B. 1992 Feb 1;48(Pt 1):67–75. doi: 10.1107/s0108768191006663. [DOI] [PubMed] [Google Scholar]
- Khouri H. E., Vernet T., Ménard R., Parlati F., Laflamme P., Tessier D. C., Gour-Salin B., Thomas D. Y., Storer A. C. Engineering of papain: selective alteration of substrate specificity by site-directed mutagenesis. Biochemistry. 1991 Sep 17;30(37):8929–8936. doi: 10.1021/bi00101a003. [DOI] [PubMed] [Google Scholar]
- Lewis S. D., Johnson F. A., Ohno A. K., Shafer J. A. Dependence of the catalytic activity of papain on the ionization of two acidic groups. J Biol Chem. 1978 Jul 25;253(14):5080–5086. [PubMed] [Google Scholar]
- Lindahl P., Abrahamson M., Björk I. Interaction of recombinant human cystatin C with the cysteine proteinases papain and actinidin. Biochem J. 1992 Jan 1;281(Pt 1):49–55. doi: 10.1042/bj2810049. [DOI] [PMC free article] [PubMed] [Google Scholar]
- Ménard R., Carrière J., Laflamme P., Plouffe C., Khouri H. E., Vernet T., Tessier D. C., Thomas D. Y., Storer A. C. Contribution of the glutamine 19 side chain to transition-state stabilization in the oxyanion hole of papain. Biochemistry. 1991 Sep 17;30(37):8924–8928. doi: 10.1021/bi00101a002. [DOI] [PubMed] [Google Scholar]
- Ménard R., Khouri H. E., Plouffe C., Dupras R., Ripoll D., Vernet T., Tessier D. C., Lalberté F., Thomas D. Y., Storer A. C. A protein engineering study of the role of aspartate 158 in the catalytic mechanism of papain. Biochemistry. 1990 Jul 17;29(28):6706–6713. doi: 10.1021/bi00480a021. [DOI] [PubMed] [Google Scholar]
- Ménard R., Khouri H. E., Plouffe C., Laflamme P., Dupras R., Vernet T., Tessier D. C., Thomas D. Y., Storer A. C. Importance of hydrogen-bonding interactions involving the side chain of Asp158 in the catalytic mechanism of papain. Biochemistry. 1991 Jun 4;30(22):5531–5538. doi: 10.1021/bi00236a028. [DOI] [PubMed] [Google Scholar]
- Ménard R., Plouffe C., Khouri H. E., Dupras R., Tessier D. C., Vernet T., Thomas D. Y., Storer A. C. Removal of an inter-domain hydrogen bond through site-directed mutagenesis: role of serine 176 in the mechanism of papain. Protein Eng. 1991 Feb;4(3):307–311. doi: 10.1093/protein/4.3.307. [DOI] [PubMed] [Google Scholar]
- Salih E., Malthouse J. P., Kowlessur D., Jarvis M., O'Driscoll M., Brocklehurst K. Differences in the chemical and catalytic characteristics of two crystallographically 'identical' enzyme catalytic sites. Characterization of actinidin and papain by a combination of pH-dependent substrate catalysis kinetics and reactivity probe studies targeted on the catalytic-site thiol group and its immediate microenvironment. Biochem J. 1987 Oct 1;247(1):181–193. doi: 10.1042/bj2470181. [DOI] [PMC free article] [PubMed] [Google Scholar]
- Shipton M., Brochlehurst K. Characterization of the papain active centre by using two-protonic-state electrophiles as reactivity probes. Evidence for nucleophilic reactivity in the un-interrupted cysteine-25-histidine-159 interactive system. Biochem J. 1978 May 1;171(2):385–401. doi: 10.1042/bj1710385. [DOI] [PMC free article] [PubMed] [Google Scholar]
- Shipton M., Kierstan M. P., Malthouse J. P., Stuchbury T., Brocklehurst K. The case for assigning a value of approximately 4 to pKa-i of the essential histidine-cysteine interactive systems of papain, bromelain and ficin. FEBS Lett. 1975 Feb 15;50(3):365–368. doi: 10.1016/0014-5793(75)80529-1. [DOI] [PubMed] [Google Scholar]
- Storer A. C., Carey P. R. Comparison of the kinetics and mechanism of the papain-catalyzed hydrolysis of esters and thiono esters. Biochemistry. 1985 Nov 19;24(24):6808–6818. doi: 10.1021/bi00345a012. [DOI] [PubMed] [Google Scholar]
- Stubbs M. T., Laber B., Bode W., Huber R., Jerala R., Lenarcic B., Turk V. The refined 2.4 A X-ray crystal structure of recombinant human stefin B in complex with the cysteine proteinase papain: a novel type of proteinase inhibitor interaction. EMBO J. 1990 Jun;9(6):1939–1947. doi: 10.1002/j.1460-2075.1990.tb08321.x. [DOI] [PMC free article] [PubMed] [Google Scholar]
- Topham C. M., Salih E., Frazao C., Kowlessur D., Overington J. P., Thomas M., Brocklehurst S. M., Patel M., Thomas E. W., Brocklehurst K. Structure-function relationships in the cysteine proteinases actinidin, papain and papaya proteinase omega. Three-dimensional structure of papaya proteinase omega deduced by knowledge-based modelling and active-centre characteristics determined by two-hydronic-state reactivity probe kinetics and kinetics of catalysis. Biochem J. 1991 Nov 15;280(Pt 1):79–92. doi: 10.1042/bj2800079. [DOI] [PMC free article] [PubMed] [Google Scholar]
- Varughese K. I., Ahmed F. R., Carey P. R., Hasnain S., Huber C. P., Storer A. C. Crystal structure of a papain-E-64 complex. Biochemistry. 1989 Feb 7;28(3):1330–1332. doi: 10.1021/bi00429a058. [DOI] [PubMed] [Google Scholar]
- Vernet T., Khouri H. E., Laflamme P., Tessier D. C., Musil R., Gour-Salin B. J., Storer A. C., Thomas D. Y. Processing of the papain precursor. Purification of the zymogen and characterization of its mechanism of processing. J Biol Chem. 1991 Nov 15;266(32):21451–21457. [PubMed] [Google Scholar]