Abstract
The question of whether, during the evolution of an enzyme, the transition state of the catalysed reaction is largely unchanged, or whether transition state and protein change together, was examined using the egb beta-galactosidases of Escherichia coli. Charge development at the first chemical state was assumed [Konstantinidis and Sinnott (1991) Biochem. J. 279, 587-593] to be proportional to delta delta G++, the ratio of second-order rate constants for the hydrolysis of beta-D-galactopyranosyl fluoride and 1-fluoro-D-galactopyranosyl fluoride, expressed as a free-energy difference. delta delta G++ (kJ.mol-1) falls from 10.4 for wild-type enzyme to 6.8 and 7.2 as a consequence of two different single amino-acid changes (which arise from single evolutionary events), to 6.3 as a consequence of the two amino-acid changes together, and then increases slightly to 7.3 as a consequence of a third single evolutionary change involving three further amino-acid changes.
Full text
PDF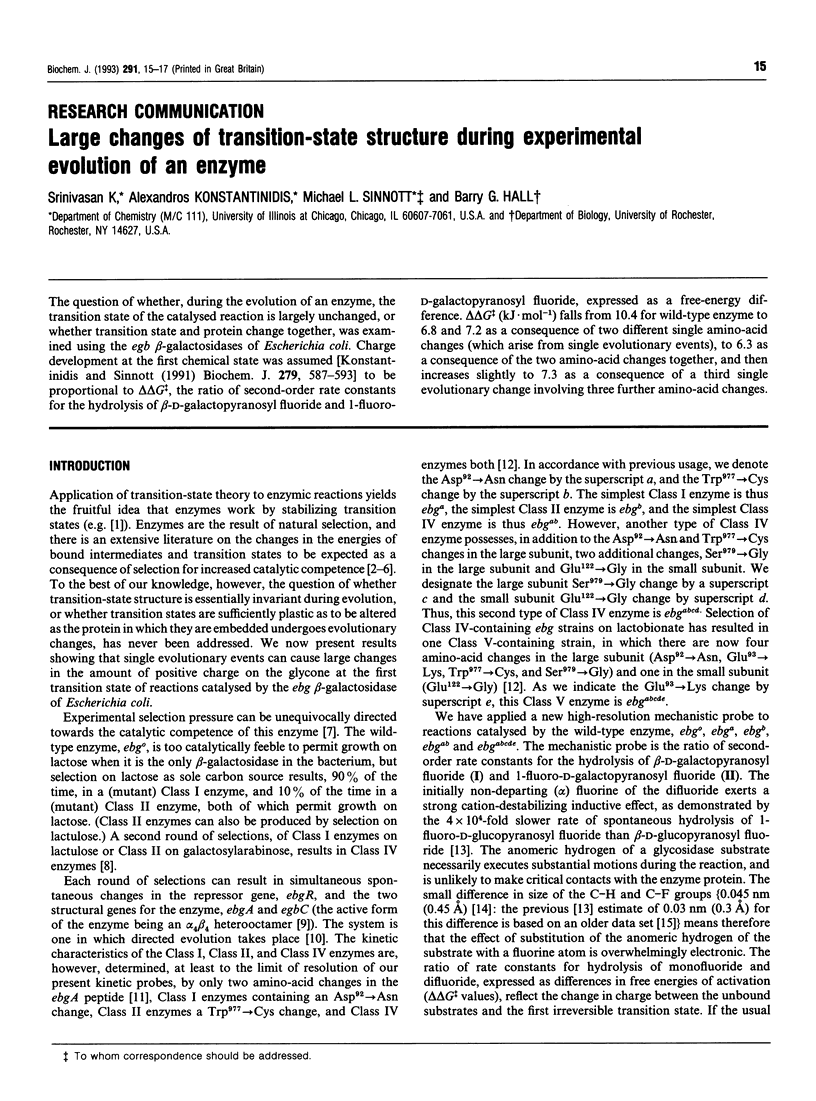
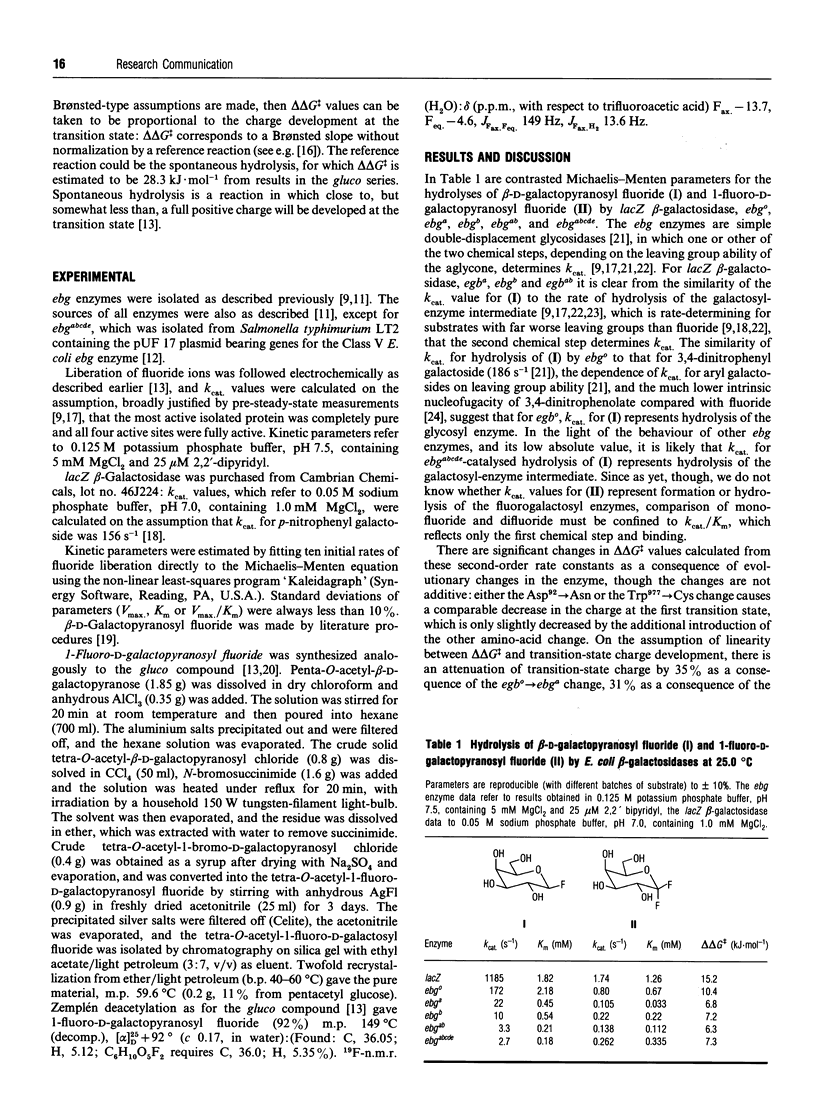
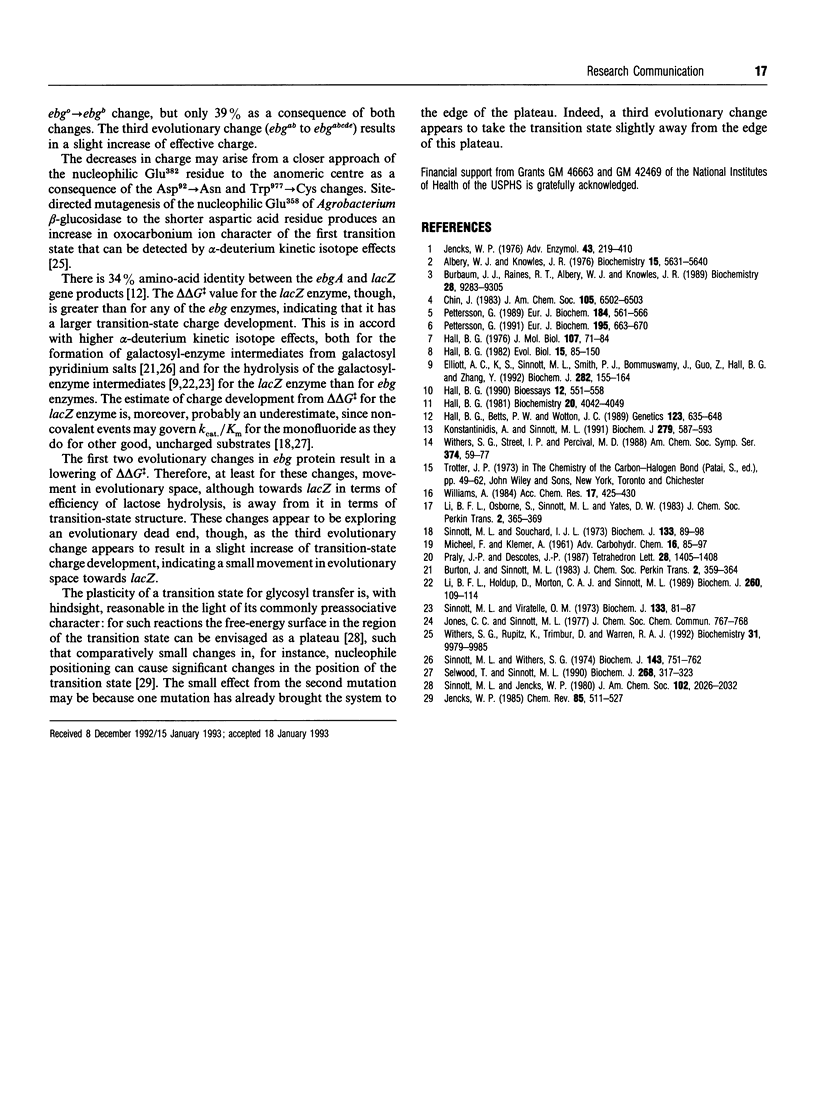
Selected References
These references are in PubMed. This may not be the complete list of references from this article.
- Albery W. J., Knowles J. R. Evolution of enzyme function and the development of catalytic efficiency. Biochemistry. 1976 Dec 14;15(25):5631–5640. doi: 10.1021/bi00670a032. [DOI] [PubMed] [Google Scholar]
- Burbaum J. J., Raines R. T., Albery W. J., Knowles J. R. Evolutionary optimization of the catalytic effectiveness of an enzyme. Biochemistry. 1989 Nov 28;28(24):9293–9305. doi: 10.1021/bi00450a009. [DOI] [PubMed] [Google Scholar]
- Elliott A. C., K S., Sinnott M. L., Smith P. J., Bommuswamy J., Guo Z., Hall B. G., Zhang Y. The catalytic consequences of experimental evolution. Studies on the subunit structure of the second (ebg) beta-galactosidase of Escherichia coli, and on catalysis by ebgab, an experimental evolvant containing two amino acid substitutions. Biochem J. 1992 Feb 15;282(Pt 1):155–164. doi: 10.1042/bj2820155. [DOI] [PMC free article] [PubMed] [Google Scholar]
- Hall B. G., Betts P. W., Wootton J. C. DNA sequence analysis of artificially evolved ebg enzyme and ebg repressor genes. Genetics. 1989 Dec;123(4):635–648. doi: 10.1093/genetics/123.4.635. [DOI] [PMC free article] [PubMed] [Google Scholar]
- Hall B. G. Changes in the substrate specificities of an enzyme during directed evolution of new functions. Biochemistry. 1981 Jul 7;20(14):4042–4049. doi: 10.1021/bi00517a015. [DOI] [PubMed] [Google Scholar]
- Hall B. G. Directed evolution of a bacterial operon. Bioessays. 1990 Nov;12(11):551–558. doi: 10.1002/bies.950121109. [DOI] [PubMed] [Google Scholar]
- Hall B. G. Experimental evolution of a new enzymatic function. Kinetic analysis of the ancestral (ebg) and evolved (ebg) enzymes. J Mol Biol. 1976 Oct 15;107(1):71–84. doi: 10.1016/s0022-2836(76)80018-6. [DOI] [PubMed] [Google Scholar]
- Jencks W. P. Binding energy, specificity, and enzymic catalysis: the circe effect. Adv Enzymol Relat Areas Mol Biol. 1975;43:219–410. doi: 10.1002/9780470122884.ch4. [DOI] [PubMed] [Google Scholar]
- Konstantinidis A., Sinnott M. L. The interaction of 1-fluoro-D-glucopyranosyl fluoride with glucosidases. Biochem J. 1991 Oct 15;279(Pt 2):587–593. doi: 10.1042/bj2790587. [DOI] [PMC free article] [PubMed] [Google Scholar]
- Li B. F., Holdup D., Morton C. A., Sinnott M. L. The catalytic consequences of experimental evolution. Transition-state structure during catalysis by the evolved beta-galactosidases of Escherichia coli (ebg enzymes) changed by a single mutational event. Biochem J. 1989 May 15;260(1):109–114. doi: 10.1042/bj2600109. [DOI] [PMC free article] [PubMed] [Google Scholar]
- MICHEEL F., KLEMER A. Glycosyl fluorides and azides. Adv Carbohydr Chem. 1961;16:85–103. doi: 10.1016/s0096-5332(08)60260-x. [DOI] [PubMed] [Google Scholar]
- Pettersson G. Effect of evolution on the kinetic properties of enzymes. Eur J Biochem. 1989 Oct 1;184(3):561–566. doi: 10.1111/j.1432-1033.1989.tb15050.x. [DOI] [PubMed] [Google Scholar]
- Pettersson G. Why do many Michaelian enzymes exhibit an equilibrium constant close to unity for the interconversion of enzyme-bound substrate and product? Eur J Biochem. 1991 Feb 14;195(3):663–670. doi: 10.1111/j.1432-1033.1991.tb15751.x. [DOI] [PubMed] [Google Scholar]
- Selwood T., Sinnott M. L. A solvent-isotope-effect study of proton transfer during catalysis by Escherichia coli (lacZ) beta-galactosidase. Biochem J. 1990 Jun 1;268(2):317–323. doi: 10.1042/bj2680317. [DOI] [PMC free article] [PubMed] [Google Scholar]
- Sinnott M. L., Souchard I. J. The mechanism of action of beta-galactosidase. Effect of aglycone nature and -deuterium substitution on the hydrolysis of aryl galactosides. Biochem J. 1973 May;133(1):89–98. doi: 10.1042/bj1330089. [DOI] [PMC free article] [PubMed] [Google Scholar]
- Sinnott M. L., Viratelle O. M. The effect of methanol and dioxan on the rates of the beta-galactosidase-catalysed hydrolyses of some beta-D-galactrophyranosides: rate-limiting degalactosylation. The ph-dependence of galactosylation and degalactosylation. Biochem J. 1973 May;133(1):81–87. doi: 10.1042/bj1330081. [DOI] [PMC free article] [PubMed] [Google Scholar]
- Sinnott M. L., Withers S. G. The beta-galactosidase-catalysed hydrolyses of beta-d-galactopyranosyl pyridium salts. Rate-limiting generation of an enzyme-bound galactopyranosyl cation in a process dependent only on aglycone acidity. Biochem J. 1974 Dec;143(3):751–762. doi: 10.1042/bj1430751. [DOI] [PMC free article] [PubMed] [Google Scholar]
- Withers S. G., Rupitz K., Trimbur D., Warren R. A. Mechanistic consequences of mutation of the active site nucleophile Glu 358 in Agrobacterium beta-glucosidase. Biochemistry. 1992 Oct 20;31(41):9979–9985. doi: 10.1021/bi00156a017. [DOI] [PubMed] [Google Scholar]