Abstract
We have investigated the mechanisms of regulation of the Na+/glucose co-transporter (SGLT1) in a ruminant animal, which is an exceptional model system for studying intestinal glucose transport. Pre-ruminant lambs absorb glucose, produced by hydrolysis of the milk sugar lactose, in the intestine via apical SGLT1 and basolateral facilitative glucose transporters (GLUT2). Weaning coincides with the development of the rumen, and consequently the amount of hexoses reaching the small intestine of the ruminant sheep is undetectable. During development, SGLT1 activity and abundance in intestinal brush-border membranes decreased by over 200-fold, and either maintaining lambs on a milk replacer diet or infusing sheep intestine with D-glucose restored co-transporter activity and expression. We have measured ovine intestinal SGLT1 mRNA levels during development, with changes in diet and after direct infusion of D-glucose or methyl alpha-D-glucopyranoside into the intestinal lumen, in order to determine the level of regulation. During development, mRNA levels decreased only 4-fold. Lambs maintained on a milk replacer diet showed no change in mRNA levels relative to age-matched controls. Finally, upon infusion of the intestine of the ruminant sheep with sugars, D-glucose infusion increased SGLT1 mRNA, but only by 2-fold, compared with a 60-90-fold increase in co-transporter number and activity. Since the change in Na(+)-dependent glucose transport activity is correlated with SGLT1 protein abundance, and since changes in mRNA levels do not account for the dramatic changes in protein abundance, we conclude that the principal level of SGLT1 regulation by luminal sugar is translational or post-translational.
Full text
PDF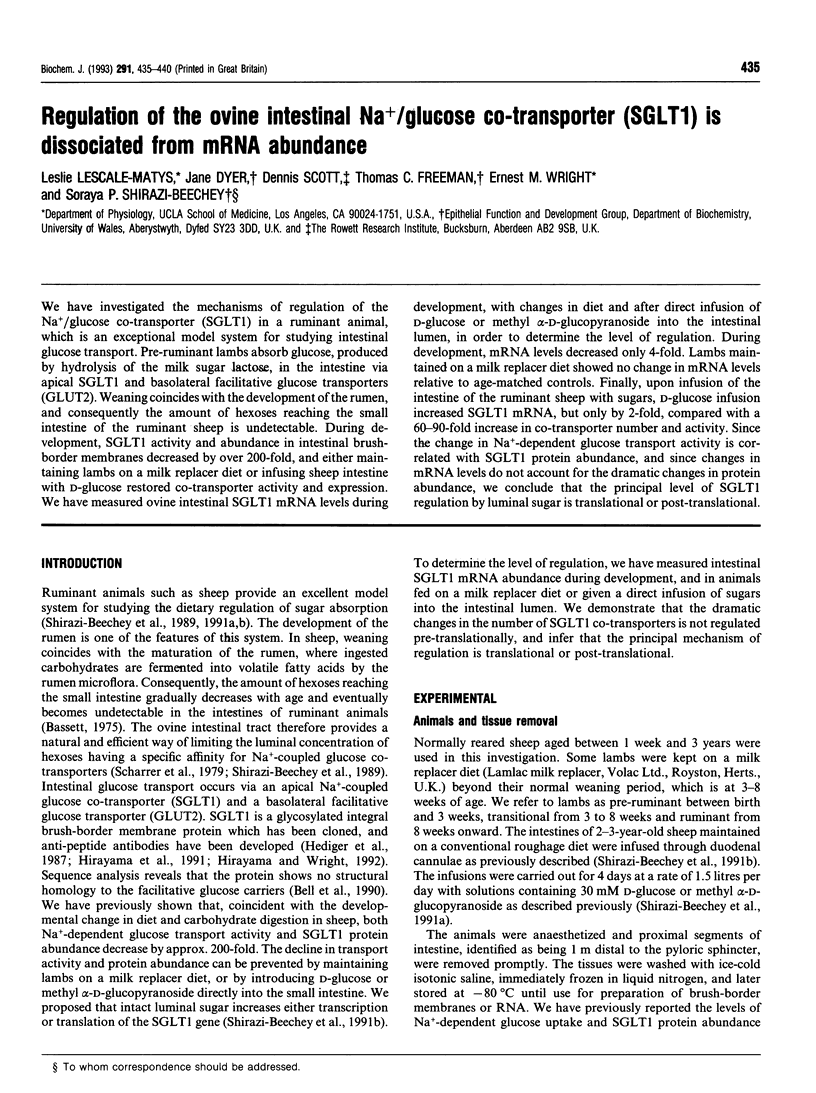
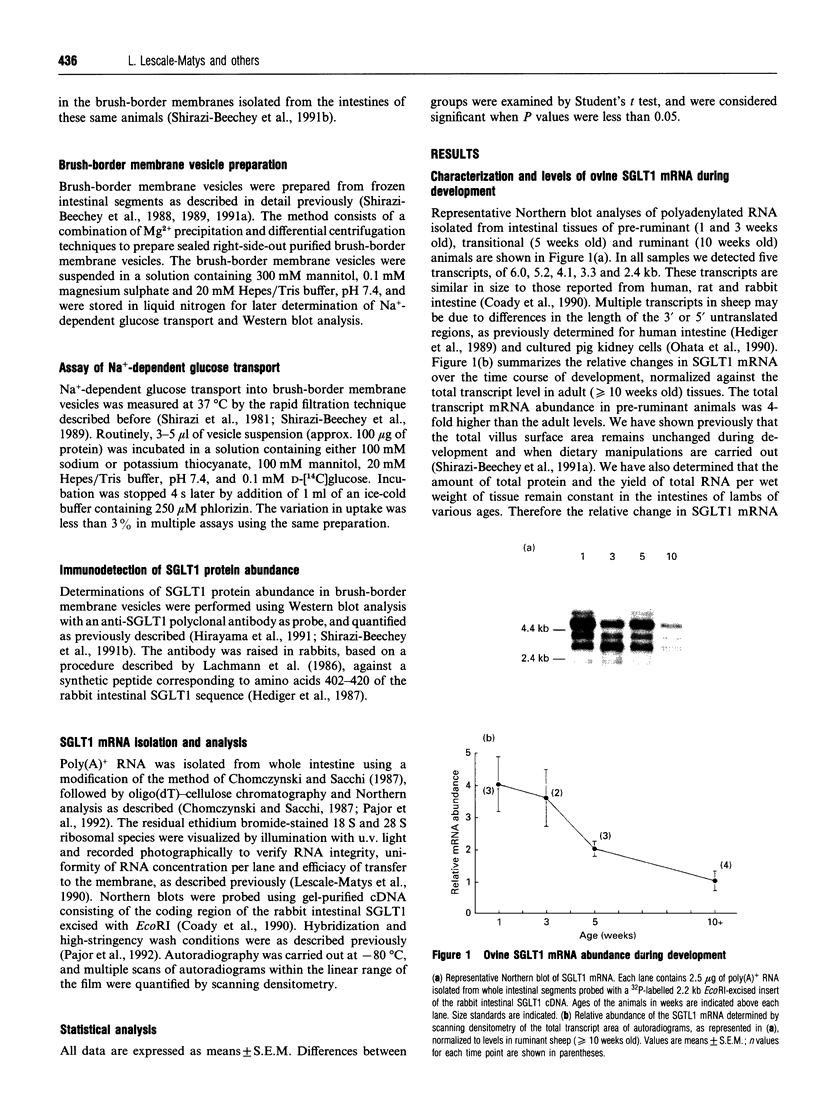
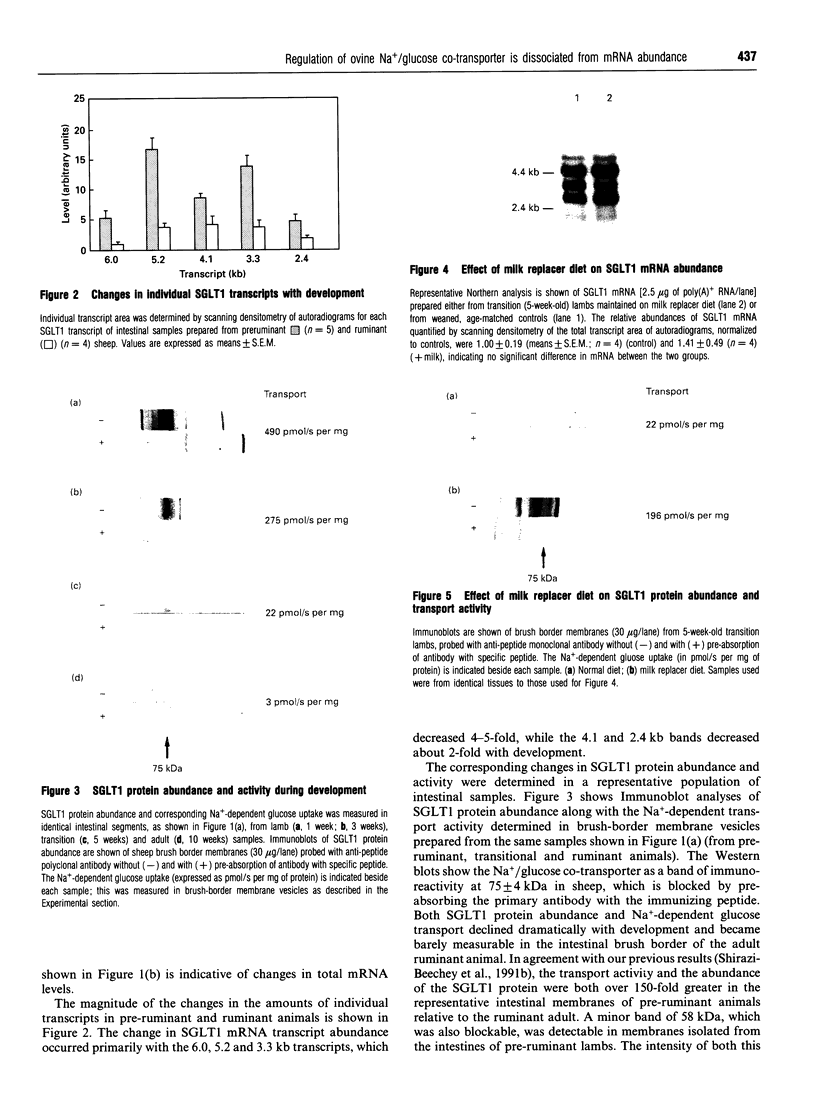
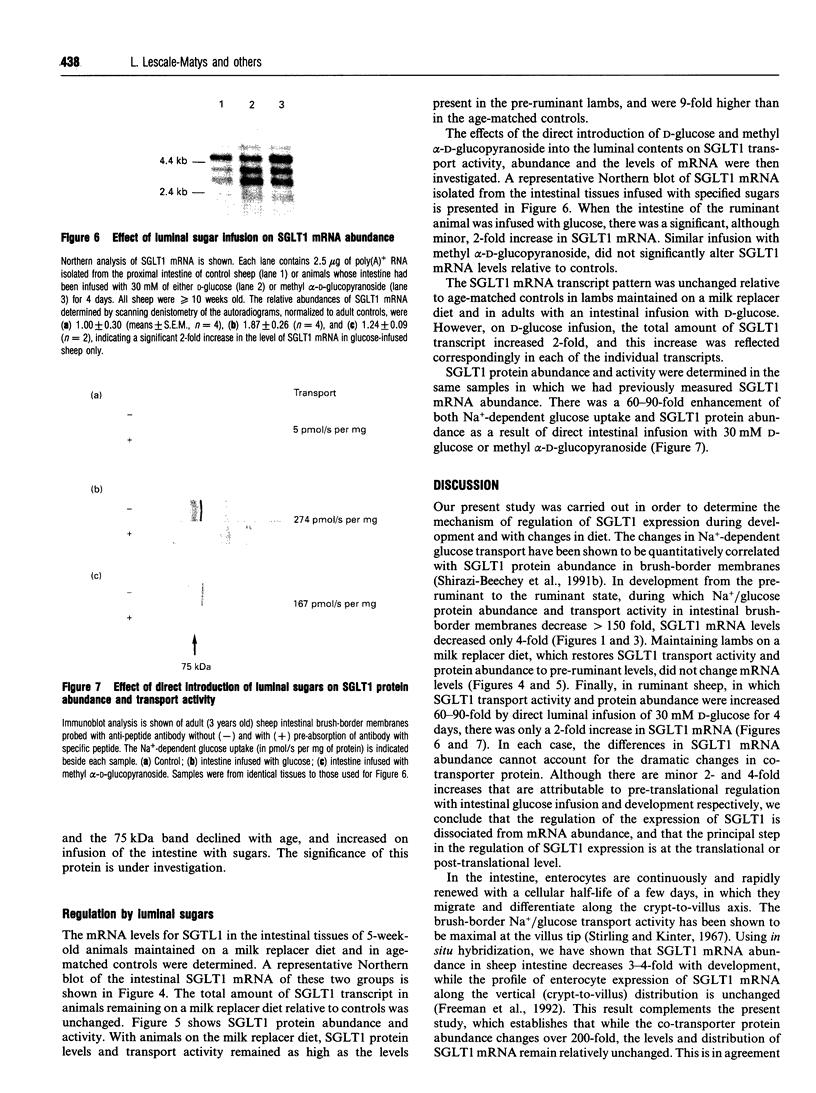
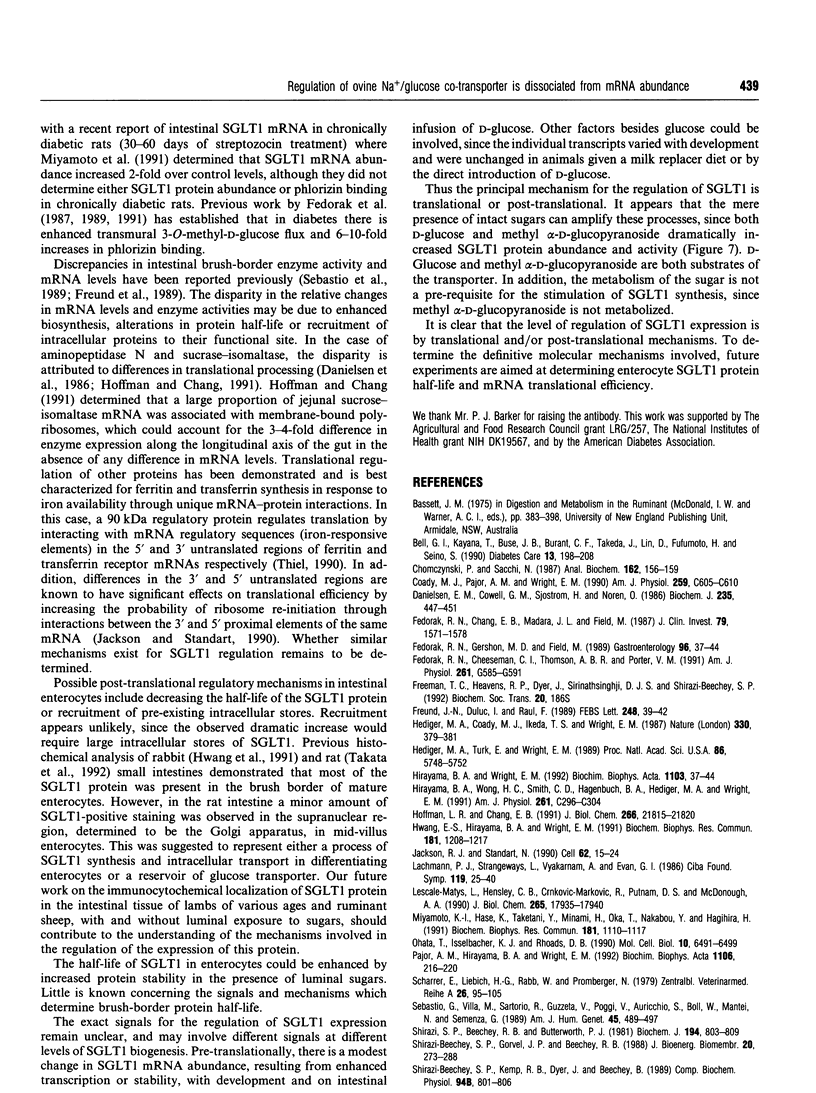
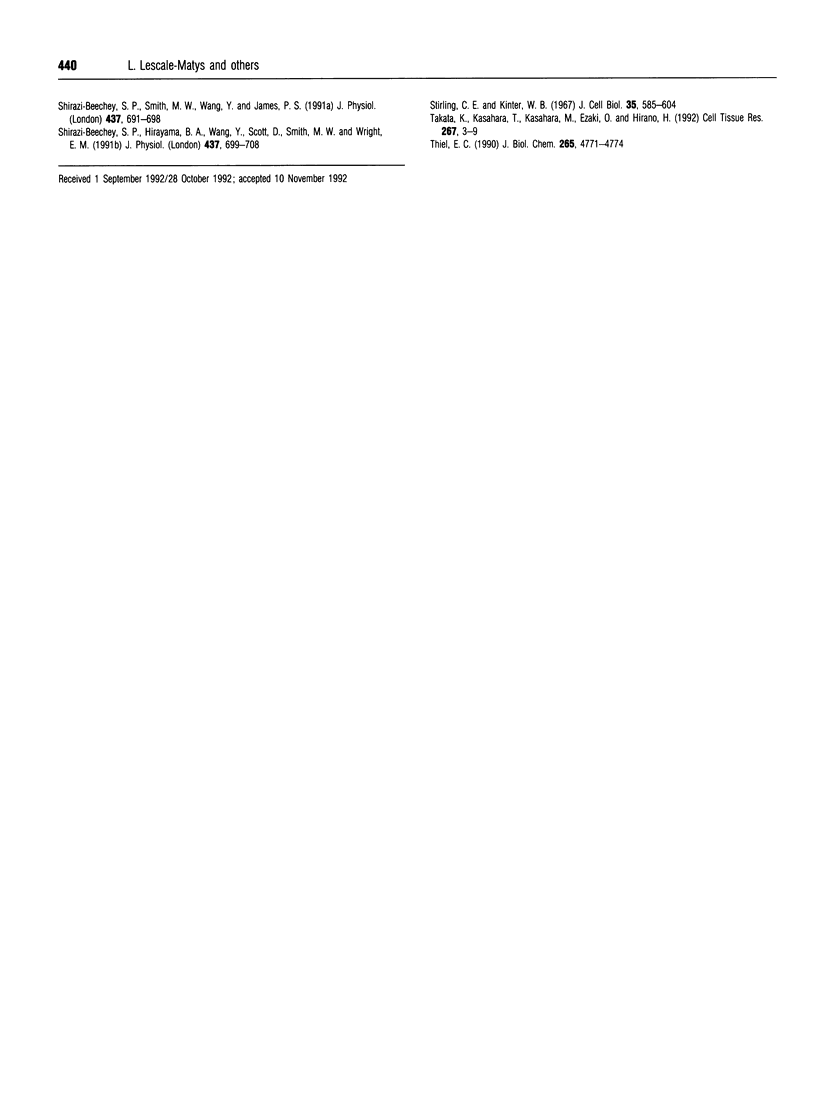
Images in this article
Selected References
These references are in PubMed. This may not be the complete list of references from this article.
- Bell G. I., Kayano T., Buse J. B., Burant C. F., Takeda J., Lin D., Fukumoto H., Seino S. Molecular biology of mammalian glucose transporters. Diabetes Care. 1990 Mar;13(3):198–208. doi: 10.2337/diacare.13.3.198. [DOI] [PubMed] [Google Scholar]
- Chomczynski P., Sacchi N. Single-step method of RNA isolation by acid guanidinium thiocyanate-phenol-chloroform extraction. Anal Biochem. 1987 Apr;162(1):156–159. doi: 10.1006/abio.1987.9999. [DOI] [PubMed] [Google Scholar]
- Coady M. J., Pajor A. M., Wright E. M. Sequence homologies among intestinal and renal Na+/glucose cotransporters. Am J Physiol. 1990 Oct;259(4 Pt 1):C605–C610. doi: 10.1152/ajpcell.1990.259.4.C605. [DOI] [PubMed] [Google Scholar]
- Danielsen E. M., Cowell G. M., Sjöström H., Norén O. Translational control of an intestinal microvillar enzyme. Biochem J. 1986 Apr 15;235(2):447–451. doi: 10.1042/bj2350447. [DOI] [PMC free article] [PubMed] [Google Scholar]
- Fedorak R. N., Chang E. B., Madara J. L., Field M. Intestinal adaptation to diabetes. Altered Na-dependent nutrient absorption in streptozocin-treated chronically diabetic rats. J Clin Invest. 1987 Jun;79(6):1571–1578. doi: 10.1172/JCI112991. [DOI] [PMC free article] [PubMed] [Google Scholar]
- Fedorak R. N., Cheeseman C. I., Thomson A. B., Porter V. M. Altered glucose carrier expression: mechanism of intestinal adaptation during streptozocin-induced diabetes in rats. Am J Physiol. 1991 Oct;261(4 Pt 1):G585–G591. doi: 10.1152/ajpgi.1991.261.4.G585. [DOI] [PubMed] [Google Scholar]
- Fedorak R. N., Gershon M. D., Field M. Induction of intestinal glucose carriers in streptozocin-treated chronically diabetic rats. Gastroenterology. 1989 Jan;96(1):37–44. doi: 10.1016/0016-5085(89)90761-0. [DOI] [PubMed] [Google Scholar]
- Freeman T. C., Heavens R. P., Dyer J., Sirinathsinghji D. J., Shirazi-Beechey S. P. The expression of the Na+/glucose cotransporter in the lamb small intestine. Biochem Soc Trans. 1992 May;20(2):186S–186S. doi: 10.1042/bst020186s. [DOI] [PubMed] [Google Scholar]
- Freund J. N., Duluc I., Raul F. Discrepancy between the intestinal lactase enzymatic activity and mRNA accumulation in sucklings and adults. Effect of starvation and thyroxine treatment. FEBS Lett. 1989 May 8;248(1-2):39–42. doi: 10.1016/0014-5793(89)80427-2. [DOI] [PubMed] [Google Scholar]
- Hediger M. A., Coady M. J., Ikeda T. S., Wright E. M. Expression cloning and cDNA sequencing of the Na+/glucose co-transporter. 1987 Nov 26-Dec 2Nature. 330(6146):379–381. doi: 10.1038/330379a0. [DOI] [PubMed] [Google Scholar]
- Hediger M. A., Turk E., Wright E. M. Homology of the human intestinal Na+/glucose and Escherichia coli Na+/proline cotransporters. Proc Natl Acad Sci U S A. 1989 Aug;86(15):5748–5752. doi: 10.1073/pnas.86.15.5748. [DOI] [PMC free article] [PubMed] [Google Scholar]
- Hirayama B. A., Wong H. C., Smith C. D., Hagenbuch B. A., Hediger M. A., Wright E. M. Intestinal and renal Na+/glucose cotransporters share common structures. Am J Physiol. 1991 Aug;261(2 Pt 1):C296–C304. doi: 10.1152/ajpcell.1991.261.2.C296. [DOI] [PubMed] [Google Scholar]
- Hirayama B. A., Wright E. M. Glycosylation of the rabbit intestinal brush border Na+/glucose cotransporter. Biochim Biophys Acta. 1992 Jan 10;1103(1):37–44. doi: 10.1016/0005-2736(92)90054-p. [DOI] [PubMed] [Google Scholar]
- Hoffman L. R., Chang E. B. Determinants of regional sucrase-isomaltase expression in adult rat small intestine. J Biol Chem. 1991 Nov 15;266(32):21815–21820. [PubMed] [Google Scholar]
- Hwang E. S., Hirayama B. A., Wright E. M. Distribution of the SGLT1 Na+/glucose cotransporter and mRNA along the crypt-villus axis of rabbit small intestine. Biochem Biophys Res Commun. 1991 Dec 31;181(3):1208–1217. doi: 10.1016/0006-291x(91)92067-t. [DOI] [PubMed] [Google Scholar]
- Jackson R. J., Standart N. Do the poly(A) tail and 3' untranslated region control mRNA translation? Cell. 1990 Jul 13;62(1):15–24. doi: 10.1016/0092-8674(90)90235-7. [DOI] [PubMed] [Google Scholar]
- Lachmann P. J., Strangeways L., Vyakarnam A., Evan G. Raising antibodies by coupling peptides to PPD and immunizing BCG-sensitized animals. Ciba Found Symp. 1986;119:25–57. doi: 10.1002/9780470513286.ch3. [DOI] [PubMed] [Google Scholar]
- Lescale-Matys L., Hensley C. B., Crnkovic-Markovic R., Putnam D. S., McDonough A. A. Low K+ increases Na,K-ATPase abundance in LLC-PK1/Cl4 cells by differentially increasing beta, and not alpha, subunit mRNA. J Biol Chem. 1990 Oct 15;265(29):17935–17940. [PubMed] [Google Scholar]
- Miyamoto K., Hase K., Taketani Y., Minami H., Oka T., Nakabou Y., Hagihira H. Diabetes and glucose transporter gene expression in rat small intestine. Biochem Biophys Res Commun. 1991 Dec 31;181(3):1110–1117. doi: 10.1016/0006-291x(91)92053-m. [DOI] [PubMed] [Google Scholar]
- Ohta T., Isselbacher K. J., Rhoads D. B. Regulation of glucose transporters in LLC-PK1 cells: effects of D-glucose and monosaccharides. Mol Cell Biol. 1990 Dec;10(12):6491–6499. doi: 10.1128/mcb.10.12.6491. [DOI] [PMC free article] [PubMed] [Google Scholar]
- Scharrer E., Liebich H. G., Raab W., Promberger N. Influence of age and rumen development on intestinal absorption of galactose and glucose in lambs. A functional and morphological study. Zentralbl Veterinarmed A. 1979 Feb;26(2):95–105. doi: 10.1111/j.1439-0442.1979.tb00655.x. [DOI] [PubMed] [Google Scholar]
- Sebastio G., Villa M., Sartorio R., Guzzetta V., Poggi V., Auricchio S., Boll W., Mantei N., Semenza G. Control of lactase in human adult-type hypolactasia and in weaning rabbits and rats. Am J Hum Genet. 1989 Oct;45(4):489–497. [PMC free article] [PubMed] [Google Scholar]
- Shirazi-Beechey S. P., Gorvel J. P., Beechey R. B. Phosphate transport in intestinal brush-border membrane. J Bioenerg Biomembr. 1988 Apr;20(2):273–288. doi: 10.1007/BF00768399. [DOI] [PubMed] [Google Scholar]
- Shirazi-Beechey S. P., Hirayama B. A., Wang Y., Scott D., Smith M. W., Wright E. M. Ontogenic development of lamb intestinal sodium-glucose co-transporter is regulated by diet. J Physiol. 1991 Jun;437:699–708. doi: 10.1113/jphysiol.1991.sp018620. [DOI] [PMC free article] [PubMed] [Google Scholar]
- Shirazi-Beechey S. P., Smith M. W., Wang Y., James P. S. Postnatal development of lamb intestinal digestive enzymes is not regulated by diet. J Physiol. 1991 Jun;437:691–698. doi: 10.1113/jphysiol.1991.sp018619. [DOI] [PMC free article] [PubMed] [Google Scholar]
- Shirazi S. P., Beechey R. B., Butterworth P. J. The use of potent inhibitors of alkaline phosphatase to investigate the role of the enzyme in intestinal transport of inorganic phosphate. Biochem J. 1981 Mar 15;194(3):803–809. doi: 10.1042/bj1940803. [DOI] [PMC free article] [PubMed] [Google Scholar]
- Stirling C. E., Kinter W. B. High-resolution radioautography of galactose-3H accumulation in rings of hamster intestine. J Cell Biol. 1967 Dec;35(3):585–604. doi: 10.1083/jcb.35.3.585. [DOI] [PMC free article] [PubMed] [Google Scholar]
- Takata K., Kasahara T., Kasahara M., Ezaki O., Hirano H. Immunohistochemical localization of Na(+)-dependent glucose transporter in rat jejunum. Cell Tissue Res. 1992 Jan;267(1):3–9. doi: 10.1007/BF00318685. [DOI] [PubMed] [Google Scholar]
- Theil E. C. Regulation of ferritin and transferrin receptor mRNAs. J Biol Chem. 1990 Mar 25;265(9):4771–4774. [PubMed] [Google Scholar]