Abstract
The usefulness in structure/function studies of molybdenum-containing hydroxylases in work with rosy mutant strains of Drosophila melanogaster has been investigated. At least 23 such strains are available, each corresponding to a single known amino acid change in the xanthine dehydrogenase sequence. Sequence comparisons permit identification, with some certainty, of regions associated with the iron-sulphur centres and the pterin molybdenum cofactor of the enzyme. Procedures have been developed and rigorously tested for the assay in gel-filtered extracts of the flies, of different catalytic activities of xanthine dehydrogenase by the use of various oxidizing and reducing substrates. These methods have been applied to 11 different rosy mutant strains that map to different regions of the sequence. All the mutations studied cause characteristic activity changes in the enzyme. In general these are consistent with the accepted assignment of the cofactors to the different domains and with the known reactivities of the molybdenum, flavin and iron-sulphur centres. Most results are interpretable in terms of the mutation affecting electron transfer to or from one redox centre only. The activity data provide evidence that FAD and the NAD+/NADH binding sites are retained in mutants mapping to the flavin domain. Therefore, despite some indications from sequence comparisons, it is concluded that the structure of this domain of xanthine dehydrogenase cannot be directly related to that of other flavoproteins for which structural data are available. The data also indicate that the artificial electron acceptor phenazine methosulphate acts at the iron-sulphur centres and suggest that these centres may not be essential for electron transfer between molybdenum and flavin. The work emphasizes the importance of combined genetic and biochemical study of rosy mutant xanthine dehydrogenase variants in probing the structure and function of enzymes of this class.
Full text
PDF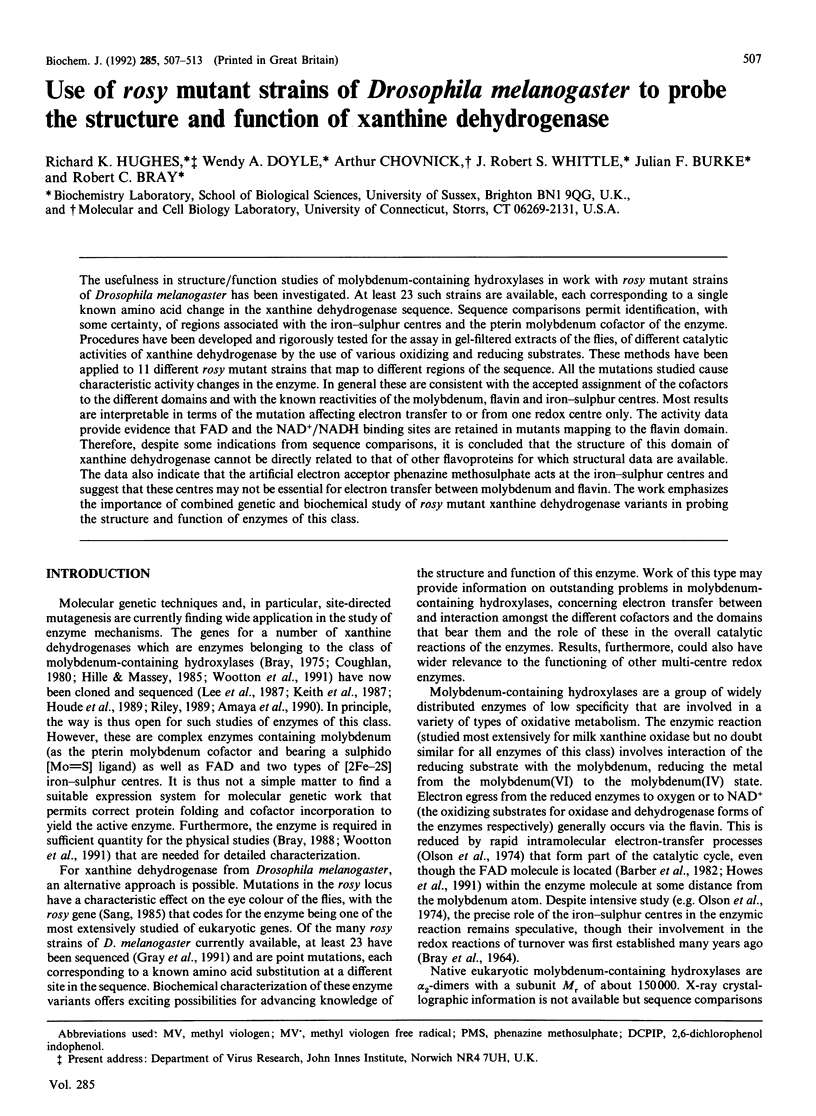
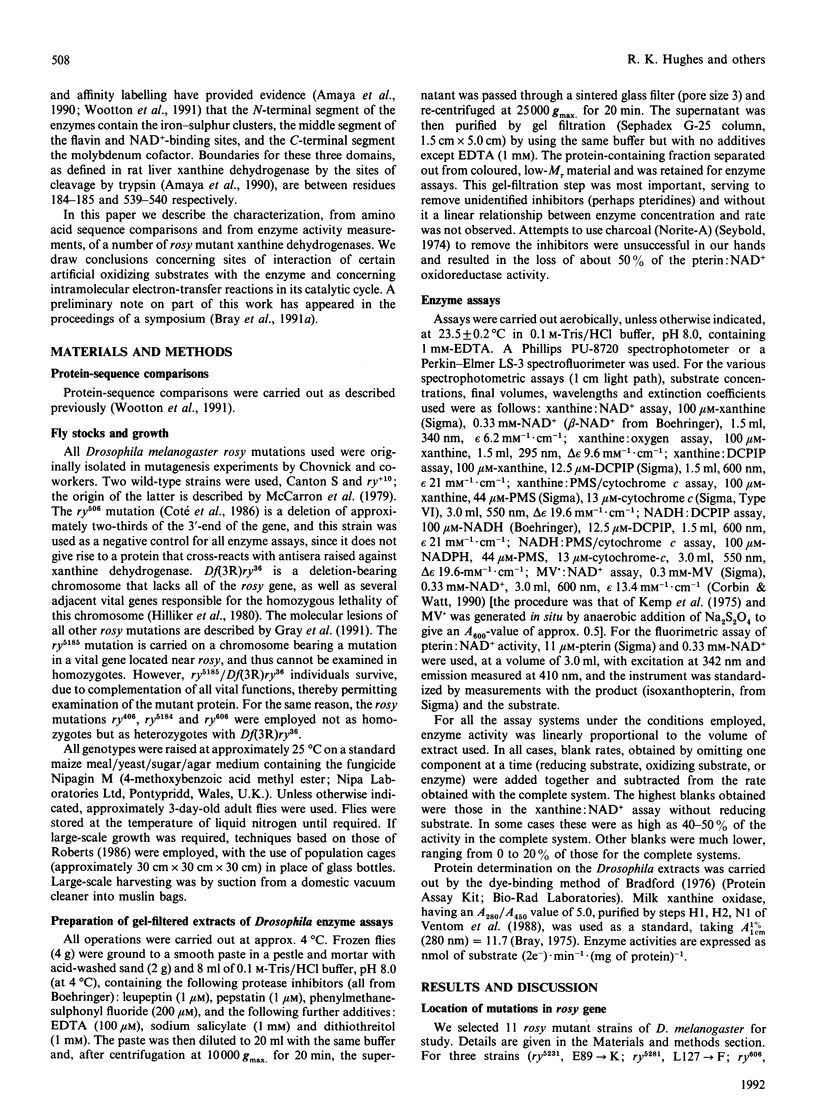
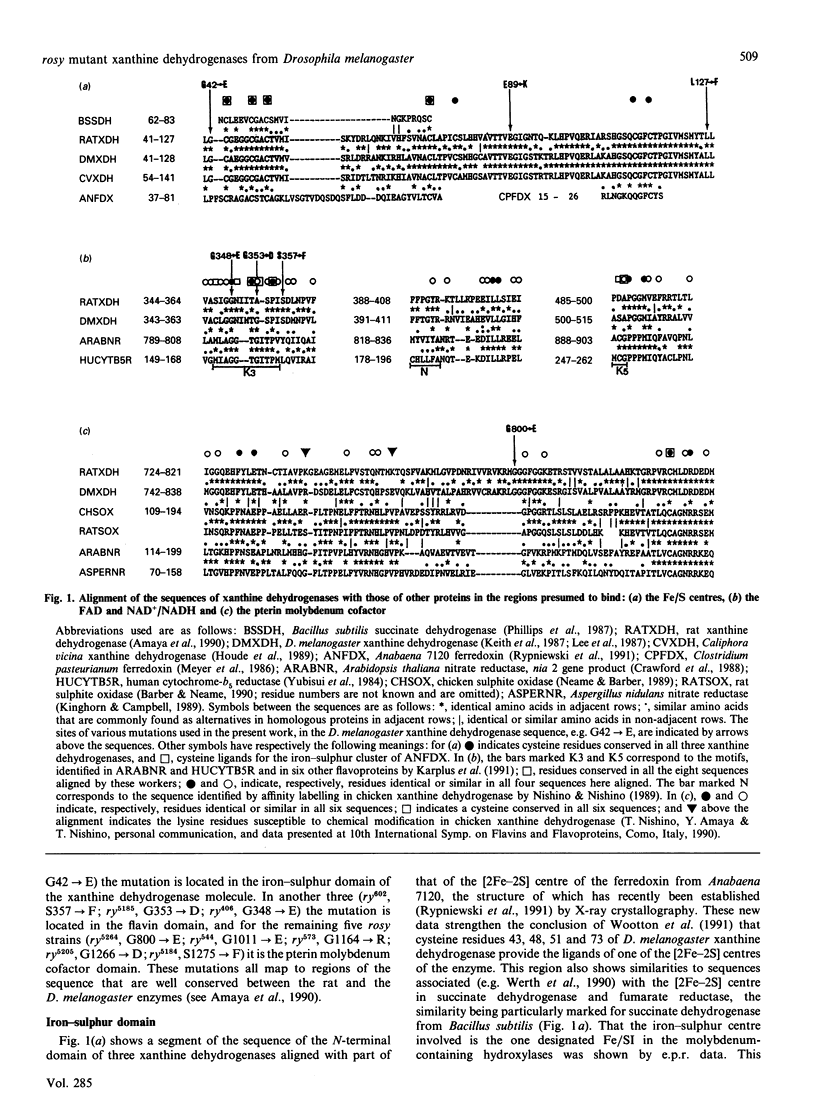
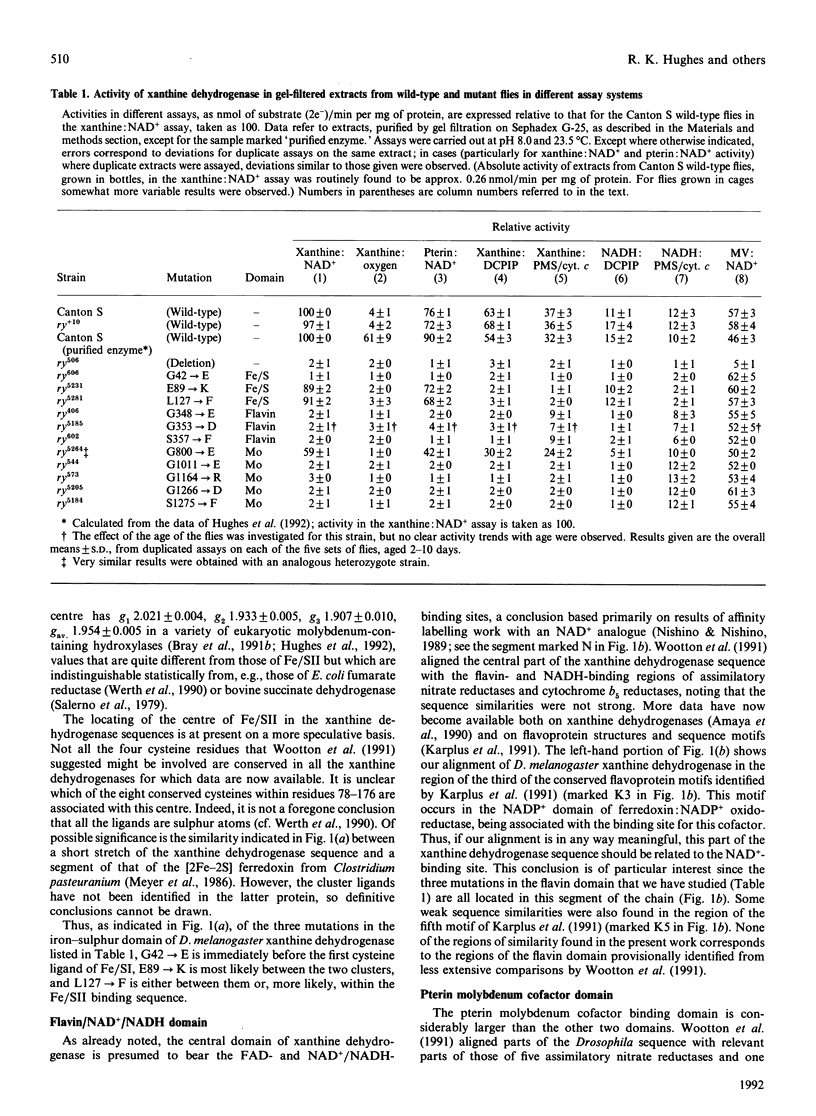
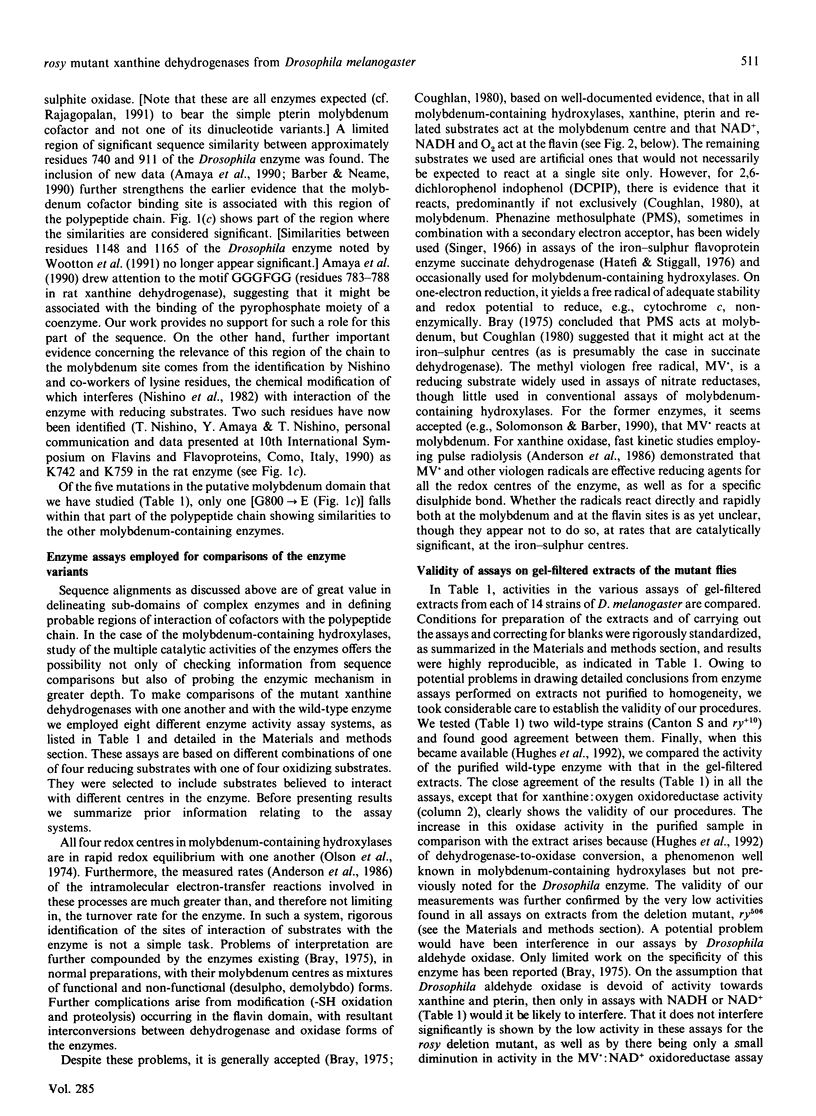
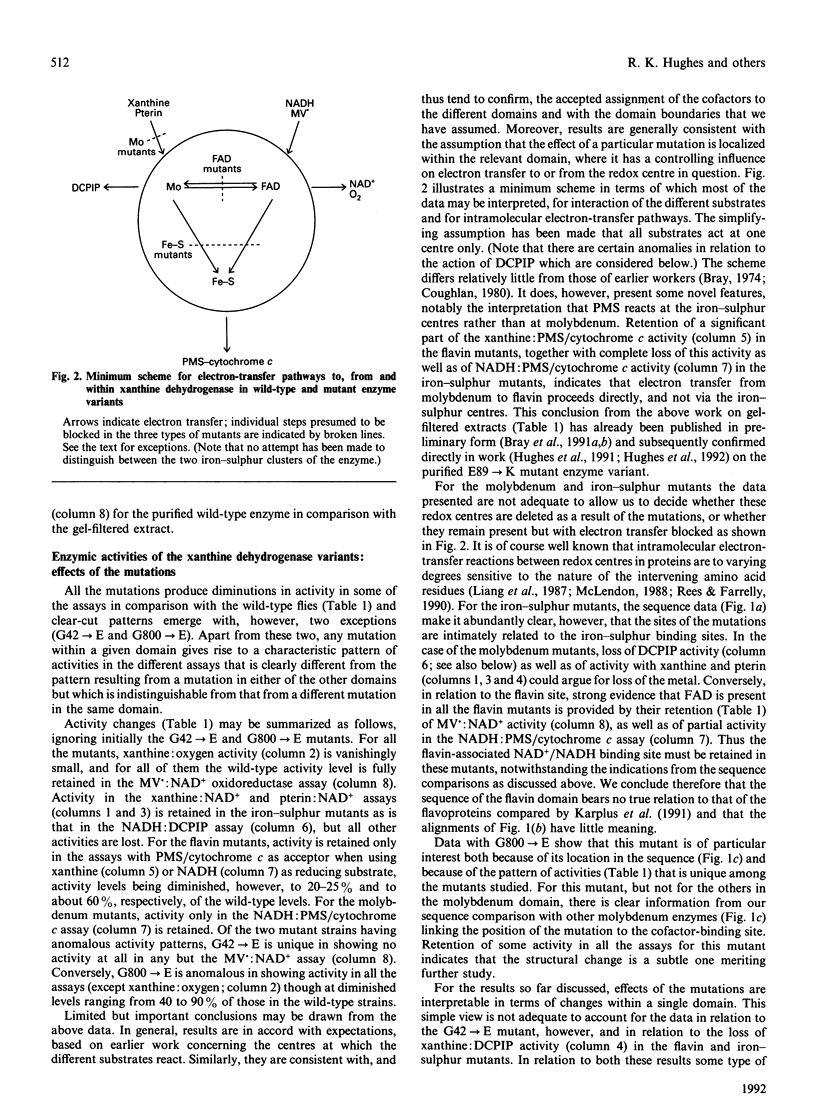
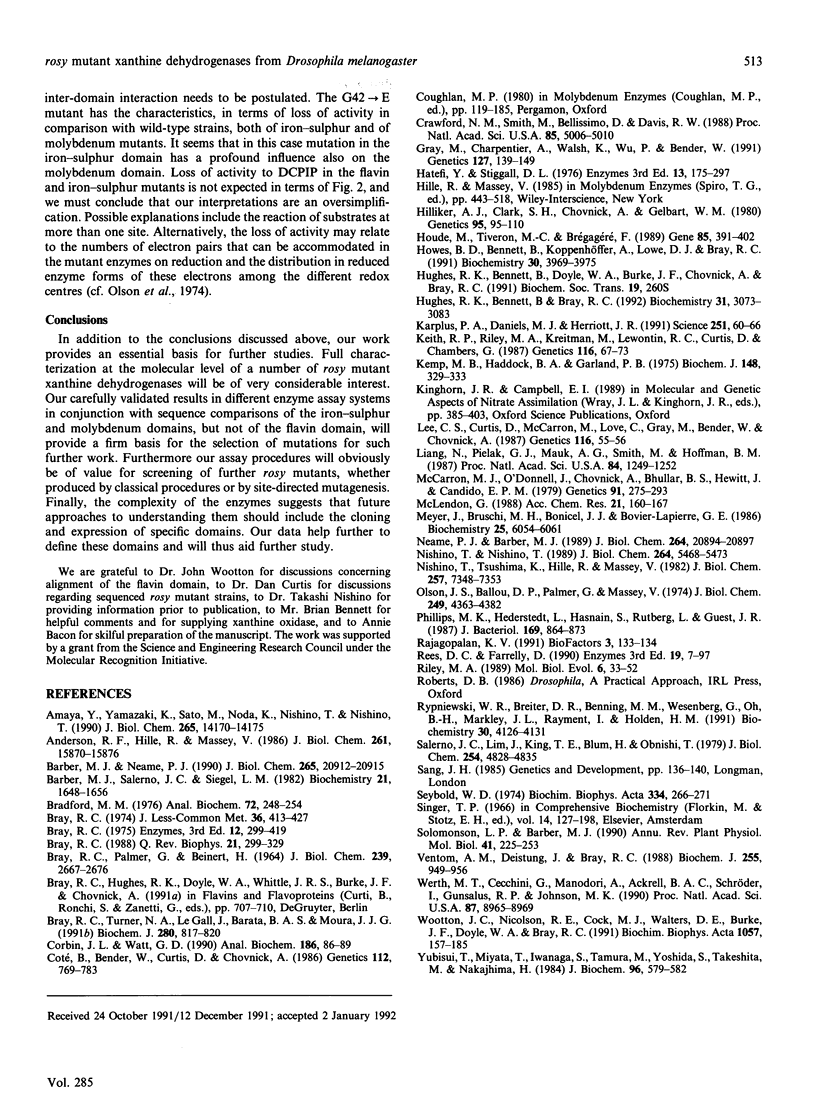
Selected References
These references are in PubMed. This may not be the complete list of references from this article.
- Amaya Y., Yamazaki K., Sato M., Noda K., Nishino T., Nishino T. Proteolytic conversion of xanthine dehydrogenase from the NAD-dependent type to the O2-dependent type. Amino acid sequence of rat liver xanthine dehydrogenase and identification of the cleavage sites of the enzyme protein during irreversible conversion by trypsin. J Biol Chem. 1990 Aug 25;265(24):14170–14175. [PubMed] [Google Scholar]
- Anderson R. F., Hille R., Massey V. The radical chemistry of milk xanthine oxidase as studied by radiation chemistry techniques. J Biol Chem. 1986 Dec 5;261(34):15870–15876. [PubMed] [Google Scholar]
- BRAY R. C., PALMER G., BEINERT H. DIRECT STUDIES ON THE ELECTRON TRANSFER SEQUENCE IN XANTHINE OXIDASE BY ELECTRON PARAMAGNETIC RESONANCE SPECTROSCOPY. II. KINETIC STUDIES EMPLOYING RAPID FREEZING. J Biol Chem. 1964 Aug;239:2667–2676. [PubMed] [Google Scholar]
- Barber M. J., Neame P. J. A conserved cysteine in molybdenum oxotransferases. J Biol Chem. 1990 Dec 5;265(34):20912–20915. [PubMed] [Google Scholar]
- Barber M. J., Salerno J. C., Siegel L. M. Magnetic interactions in milk xanthine oxidase. Biochemistry. 1982 Mar 30;21(7):1648–1656. doi: 10.1021/bi00536a027. [DOI] [PubMed] [Google Scholar]
- Bradford M. M. A rapid and sensitive method for the quantitation of microgram quantities of protein utilizing the principle of protein-dye binding. Anal Biochem. 1976 May 7;72:248–254. doi: 10.1016/0003-2697(76)90527-3. [DOI] [PubMed] [Google Scholar]
- Bray R. C. The inorganic biochemistry of molybdoenzymes. Q Rev Biophys. 1988 Aug;21(3):299–329. doi: 10.1017/s0033583500004479. [DOI] [PubMed] [Google Scholar]
- Bray R. C., Turner N. A., Le Gall J., Barata B. A., Moura J. J. Information from e.p.r. spectroscopy on the iron-sulphur centres of the iron-molybdenum protein (aldehyde oxidoreductase) of Desulfovibrio gigas. Biochem J. 1991 Dec 15;280(Pt 3):817–820. doi: 10.1042/bj2800817. [DOI] [PMC free article] [PubMed] [Google Scholar]
- Corbin J. L., Watt G. D. A chemical preparation of pure reduced viologens for use as biomolecular reducing reagents. Anal Biochem. 1990 Apr;186(1):86–89. doi: 10.1016/0003-2697(90)90577-v. [DOI] [PubMed] [Google Scholar]
- Coté B., Bender W., Curtis D., Chovnick A. Molecular mapping of the rosy locus in Drosophila melanogaster. Genetics. 1986 Apr;112(4):769–783. doi: 10.1093/genetics/112.4.769. [DOI] [PMC free article] [PubMed] [Google Scholar]
- Crawford N. M., Smith M., Bellissimo D., Davis R. W. Sequence and nitrate regulation of the Arabidopsis thaliana mRNA encoding nitrate reductase, a metalloflavoprotein with three functional domains. Proc Natl Acad Sci U S A. 1988 Jul;85(14):5006–5010. doi: 10.1073/pnas.85.14.5006. [DOI] [PMC free article] [PubMed] [Google Scholar]
- Gray M., Charpentier A., Walsh K., Wu P., Bender W. Mapping point mutations in the Drosophila rosy locus using denaturing gradient gel blots. Genetics. 1991 Jan;127(1):139–149. doi: 10.1093/genetics/127.1.139. [DOI] [PMC free article] [PubMed] [Google Scholar]
- Hilliker A. J., Clark S. H., Chovnick A., Gelbart W. M. Cytogenetic analysis of the chromosomal region immediately adjacent to the rosy locus in Drosophila melanogaster. Genetics. 1980 May;95(1):95–110. doi: 10.1093/genetics/95.1.95. [DOI] [PMC free article] [PubMed] [Google Scholar]
- Houde M., Tiveron M. C., Brégégère F. Divergence of the nucleotide sequences encoding xanthine dehydrogenase in Calliphora vicina and Drosophila melanogaster. Gene. 1989 Dec 28;85(2):391–402. doi: 10.1016/0378-1119(89)90432-0. [DOI] [PubMed] [Google Scholar]
- Howes B. D., Bennett B., Koppenhöfer A., Lowe D. J., Bray R. C. 31P ENDOR studies of xanthine oxidase: coupling of phosphorus of the pterin cofactor to molybdenum (V). Biochemistry. 1991 Apr 23;30(16):3969–3975. doi: 10.1021/bi00230a024. [DOI] [PubMed] [Google Scholar]
- Hughes R. K., Bennett B., Doyle W. A., Burke J. F., Chovnick A., Bray R. C. Roles of molybdenum, FAD and iron-sulphur domains in molybdenum-containing hydroxylases: molecular genetic, kinetic and spectroscopic studies. Biochem Soc Trans. 1991 Aug;19(3):260S–260S. doi: 10.1042/bst019260s. [DOI] [PubMed] [Google Scholar]
- Hughes R. K. Xanthine dehydrogenase from Drosophila melanogaster: purification and properties of the wild-type enzyme and of a variant lacking iron-sulfur centers. Biochemistry. 1992 Mar 31;31(12):3073–3083. doi: 10.1021/bi00127a007. [DOI] [PubMed] [Google Scholar]
- Karplus P. A., Daniels M. J., Herriott J. R. Atomic structure of ferredoxin-NADP+ reductase: prototype for a structurally novel flavoenzyme family. Science. 1991 Jan 4;251(4989):60–66. [PubMed] [Google Scholar]
- Keith T. P., Riley M. A., Kreitman M., Lewontin R. C., Curtis D., Chambers G. Sequence of the structural gene for xanthine dehydrogenase (rosy locus) in Drosophila melanogaster. Genetics. 1987 May;116(1):67–73. doi: 10.1093/genetics/116.1.67. [DOI] [PMC free article] [PubMed] [Google Scholar]
- Lee C. S., Curtis D., McCarron M., Love C., Gray M., Bender W., Chovnick A. Mutations affecting expression of the rosy locus in Drosophila melanogaster. Genetics. 1987 May;116(1):55–66. doi: 10.1093/genetics/116.1.55. [DOI] [PMC free article] [PubMed] [Google Scholar]
- Liang N., Pielak G. J., Mauk A. G., Smith M., Hoffman B. M. Yeast cytochrome c with phenylalanine or tyrosine at position 87 transfers electrons to (zinc cytochrome c peroxidase)+ at a rate ten thousand times that of the serine-87 or glycine-87 variants. Proc Natl Acad Sci U S A. 1987 Mar;84(5):1249–1252. doi: 10.1073/pnas.84.5.1249. [DOI] [PMC free article] [PubMed] [Google Scholar]
- McCarron M., O'Donnell J., Chovnick A., Bhullar B. S., Hewitt J., Candido E. P. Organization of the rosy locus in Drosophila melanogaster: further evidence in support of a cis-acting control element adjacent to the xanthine dehydrogenase structural element. Genetics. 1979 Feb;91(2):275–293. doi: 10.1093/genetics/91.2.275. [DOI] [PMC free article] [PubMed] [Google Scholar]
- Meyer J., Bruschi M. H., Bonicel J. J., Bovier-Lapierre G. E. Amino acid sequence of [2Fe-2S] ferredoxin from Clostridium pasteurianum. Biochemistry. 1986 Oct 7;25(20):6054–6061. doi: 10.1021/bi00368a033. [DOI] [PubMed] [Google Scholar]
- Neame P. J., Barber M. J. Conserved domains in molybdenum hydroxylases. The amino acid sequence of chicken hepatic sulfite oxidase. J Biol Chem. 1989 Dec 15;264(35):20894–20901. [PubMed] [Google Scholar]
- Nishino T., Nishino T. The nicotinamide adenine dinucleotide-binding site of chicken liver xanthine dehydrogenase. Evidence for alteration of the redox potential of the flavin by NAD binding or modification of the NAD-binding site and isolation of a modified peptide. J Biol Chem. 1989 Apr 5;264(10):5468–5473. [PubMed] [Google Scholar]
- Nishino T., Tsushima K., Hille R., Massey V. Inhibition of milk xanthine oxidase by fluorodinitrobenzene. J Biol Chem. 1982 Jul 10;257(13):7348–7353. [PubMed] [Google Scholar]
- Olson J. S., Ballou D. P., Palmer G., Massey V. The mechanism of action of xanthine oxidase. J Biol Chem. 1974 Jul 25;249(14):4363–4382. [PubMed] [Google Scholar]
- Phillips M. K., Hederstedt L., Hasnain S., Rutberg L., Guest J. R. Nucleotide sequence encoding the flavoprotein and iron-sulfur protein subunits of the Bacillus subtilis PY79 succinate dehydrogenase complex. J Bacteriol. 1987 Feb;169(2):864–873. doi: 10.1128/jb.169.2.864-873.1987. [DOI] [PMC free article] [PubMed] [Google Scholar]
- Riley M. A. Nucleotide sequence of the Xdh region in Drosophila pseudoobscura and an analysis of the evolution of synonymous codons. Mol Biol Evol. 1989 Jan;6(1):33–52. doi: 10.1093/oxfordjournals.molbev.a040529. [DOI] [PubMed] [Google Scholar]
- Rypniewski W. R., Breiter D. R., Benning M. M., Wesenberg G., Oh B. H., Markley J. L., Rayment I., Holden H. M. Crystallization and structure determination to 2.5-A resolution of the oxidized [2Fe-2S] ferredoxin isolated from Anabaena 7120. Biochemistry. 1991 Apr 30;30(17):4126–4131. doi: 10.1021/bi00231a003. [DOI] [PubMed] [Google Scholar]
- Salerno J. C., Lim J., King T. E., Blum H., Ohnishi T. The spatial relationships and structure of the binuclear iron-sulfur clusters in succinate dehydrogenase. J Biol Chem. 1979 Jun 10;254(11):4828–4835. [PubMed] [Google Scholar]
- Synthesis and sideedness of membrane-bound respiratory nitrate reductase (EC1.7.99.4) in Escherichia coli lacking cytochromes. Biochem J. 1975 May;148(2):329–333. [PMC free article] [PubMed] [Google Scholar]
- Ventom A. M., Deistung J., Bray R. C. The isolation of demolybdo xanthine oxidase from bovine milk. Biochem J. 1988 Nov 1;255(3):949–956. doi: 10.1042/bj2550949. [DOI] [PMC free article] [PubMed] [Google Scholar]
- Werth M. T., Cecchini G., Manodori A., Ackrell B. A., Schröder I., Gunsalus R. P., Johnson M. K. Site-directed mutagenesis of conserved cysteine residues in Escherichia coli fumarate reductase: modification of the spectroscopic and electrochemical properties of the [2Fe-2S] cluster. Proc Natl Acad Sci U S A. 1990 Nov;87(22):8965–8969. doi: 10.1073/pnas.87.22.8965. [DOI] [PMC free article] [PubMed] [Google Scholar]
- Wootton J. C., Nicolson R. E., Cock J. M., Walters D. E., Burke J. F., Doyle W. A., Bray R. C. Enzymes depending on the pterin molybdenum cofactor: sequence families, spectroscopic properties of molybdenum and possible cofactor-binding domains. Biochim Biophys Acta. 1991 Mar 29;1057(2):157–185. doi: 10.1016/s0005-2728(05)80100-8. [DOI] [PubMed] [Google Scholar]
- Yubisui T., Miyata T., Iwanaga S., Tamura M., Yoshida S., Takeshita M., Nakajima H. Amino acid sequence of NADH-cytochrome b5 reductase of human erythrocytes. J Biochem. 1984 Aug;96(2):579–582. doi: 10.1093/oxfordjournals.jbchem.a134871. [DOI] [PubMed] [Google Scholar]