Abstract
Recombinant antithrombin produced by baby hamster kidney (BHK) or Chinese hamster ovary (CHO) cells was separated into two fractions, containing comparable amounts of protein, by affinity chromatography on matrix-linked heparin. Fluorescence titrations showed that the more tightly binding fraction had a heparin affinity similar to that of plasma antithrombin (Kd approximately 20 nM), whereas the affinity of the more weakly binding fraction was nearly 10-fold lower (Kd approximately 175 nM). Analyses of the heparin-catalysed rate of inhibition of thrombin further showed that the fractions differed only in their affinity for heparin and not in the intrinsic rate constant of either the uncatalysed or the heparin-catalysed inactivation of thrombin. The recombinant antithrombin fraction with lower heparin affinity migrated more slowly than both the fraction with higher affinity and plasma antithrombin in SDS/PAGE under reducing conditions, consistent with a slightly higher apparent relative molecular mass. This apparent size difference was abolished by the enzymic removal of the carbohydrate side chains from the proteins. Such removal also increased the heparin affinity of the weakly binding fraction, so that it eluted from matrix-linked heparin at a similar position to the deglycosylated tightly binding fraction or plasma antithrombin. Analyses of N-linked carbohydrate side chains showed that the weakly binding fraction from CHO cells had a higher proportion of tetra-antennary and a lower proportion of biantennary oligosaccharides than the tightly binding fraction. We conclude that the recombinant antithrombin produced by the two cell lines is heterogeneously glycosylated and that the increased carbohydrate content of a large proportion of the molecules results in a substantial decrease in the affinity of these molecules for heparin. These findings are of particular relevance for studies aimed at characterizing the heparin-binding site of recombinant antithrombin by site-directed mutagenesis.
Full text
PDF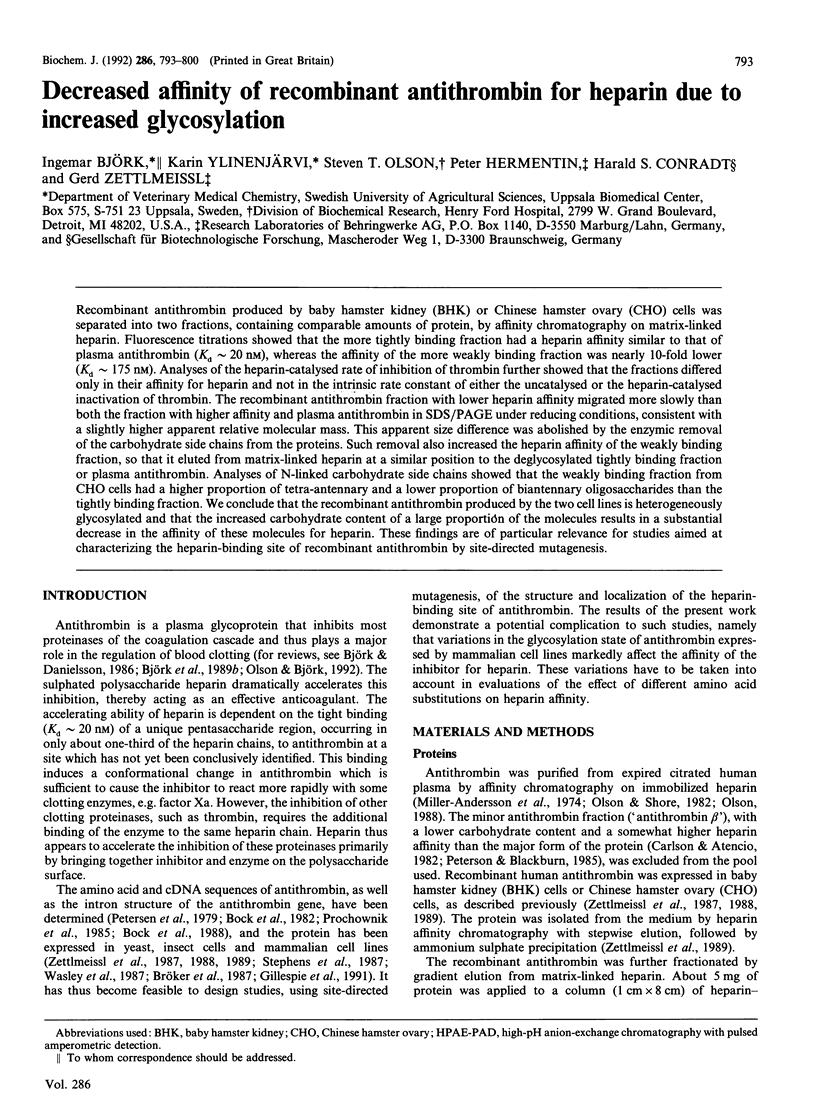
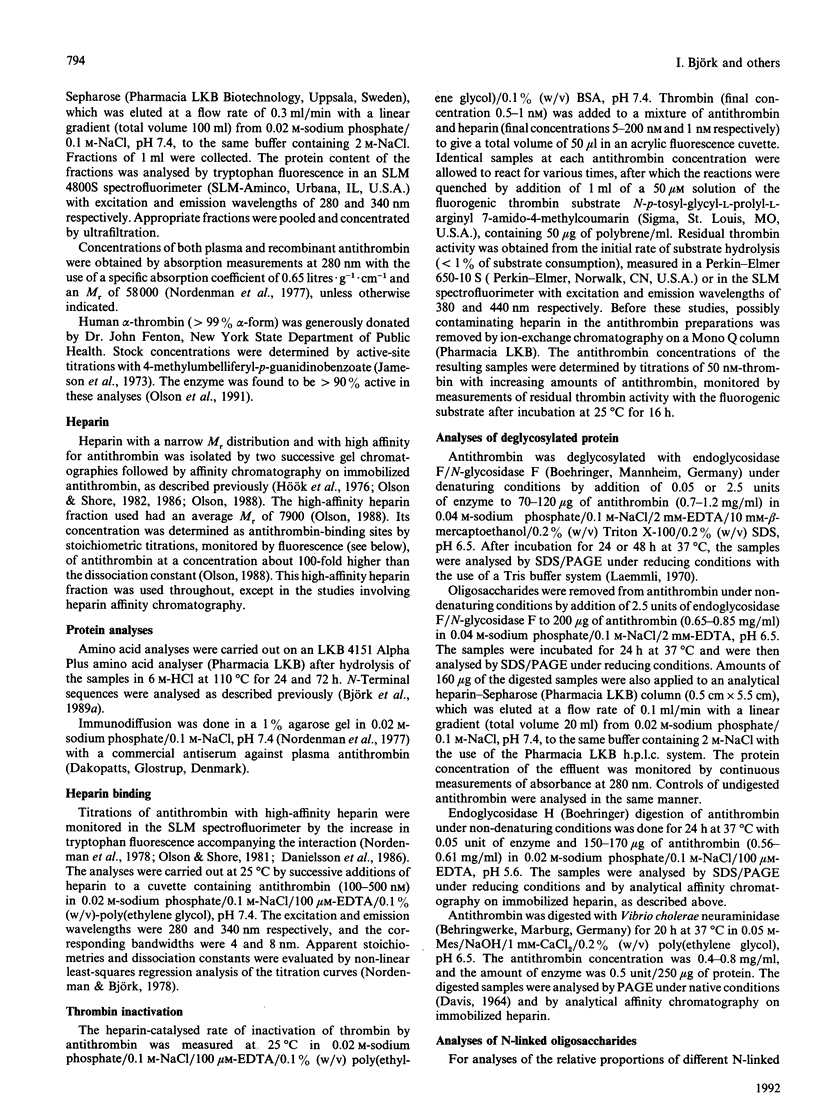
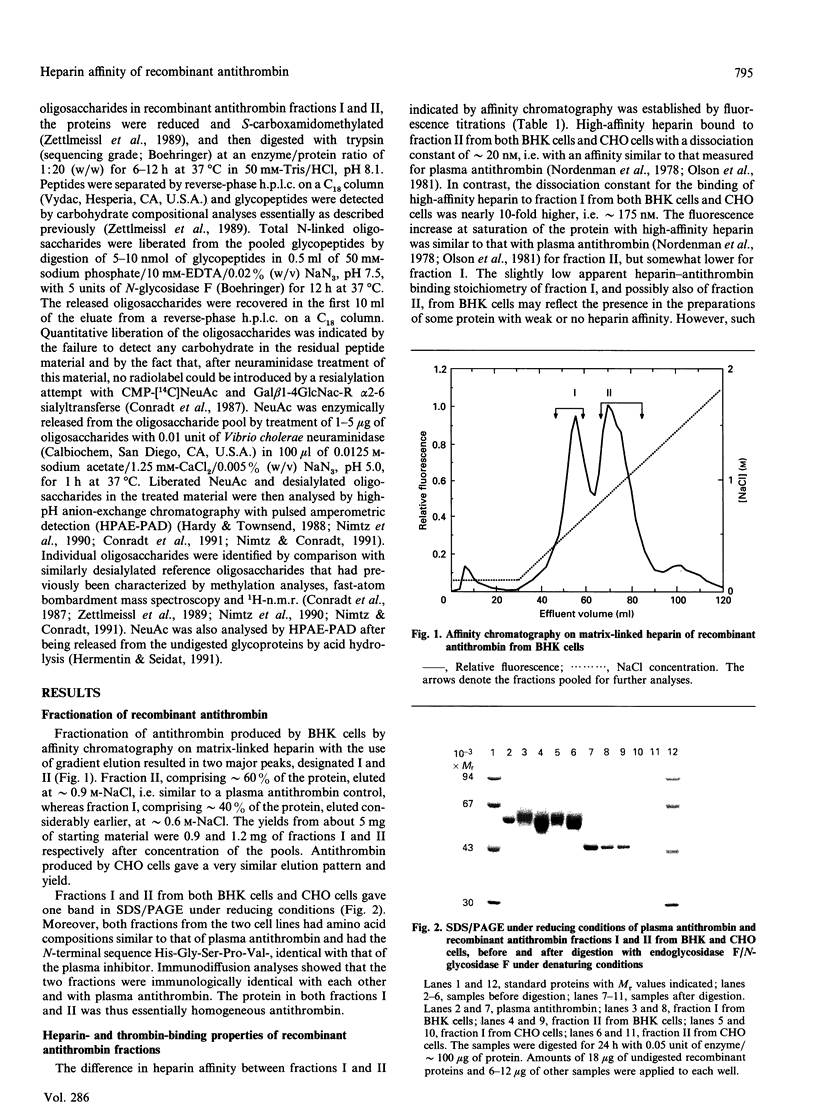
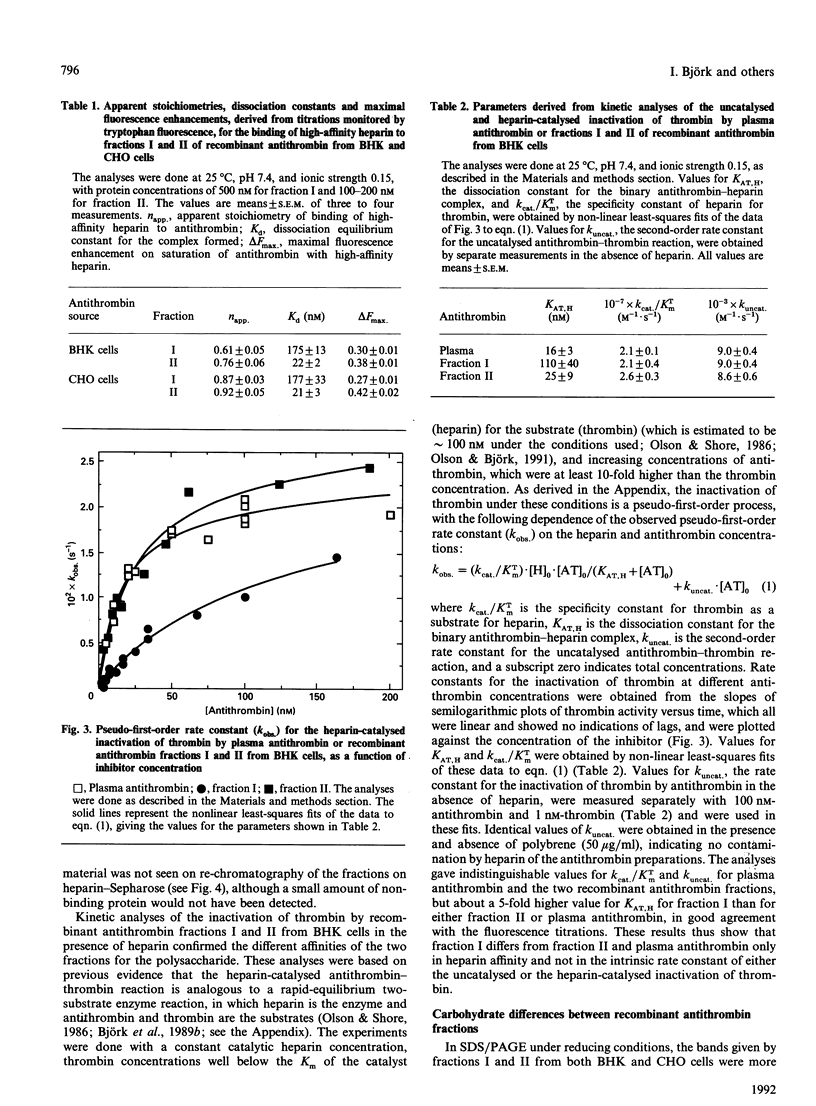
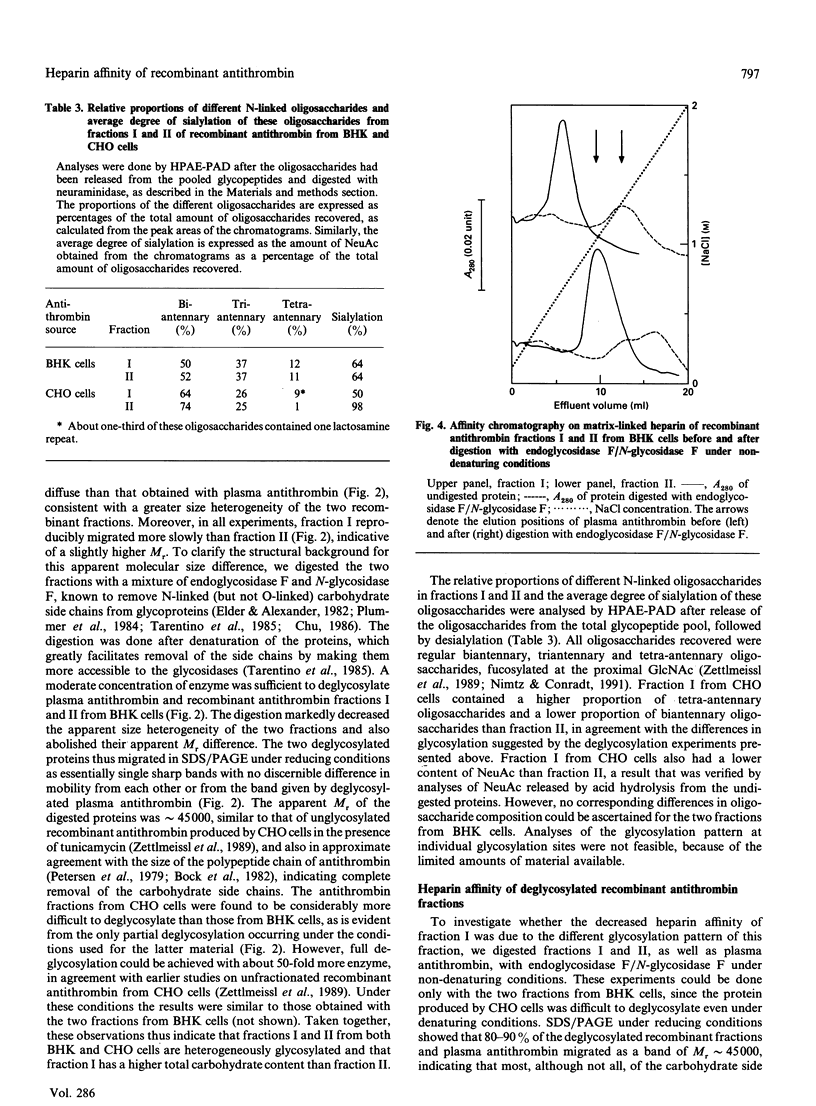
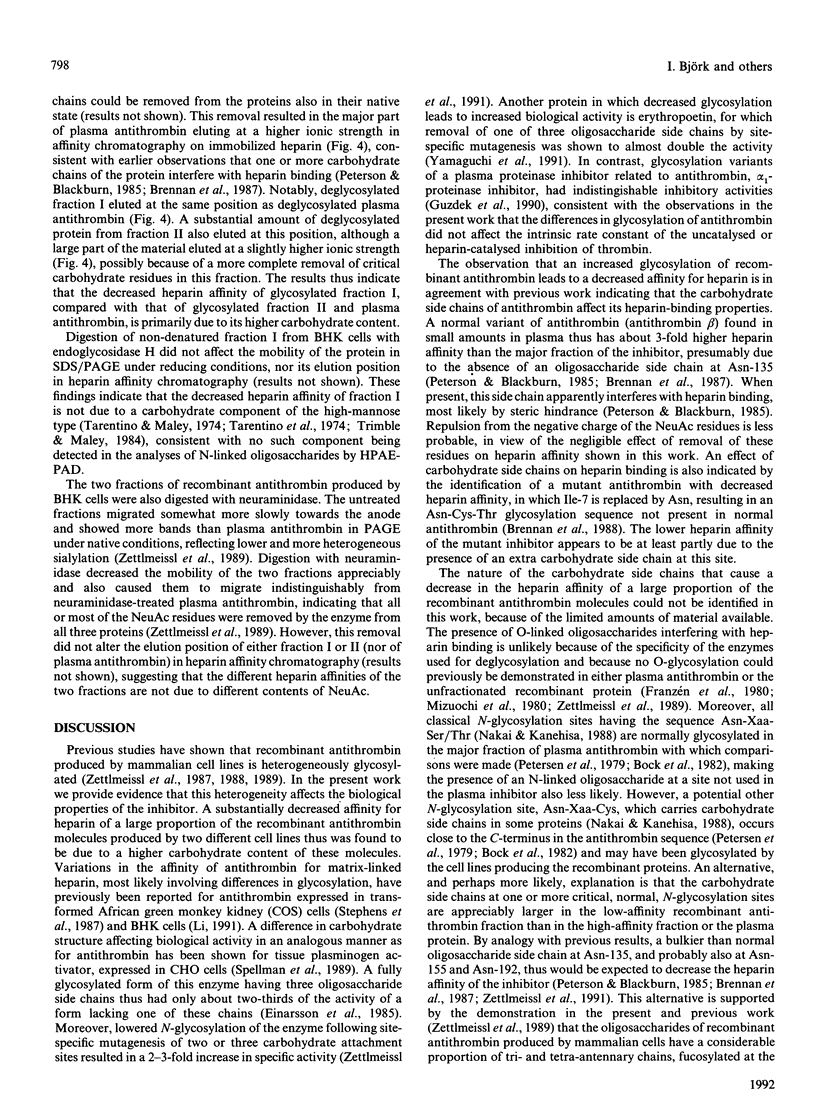
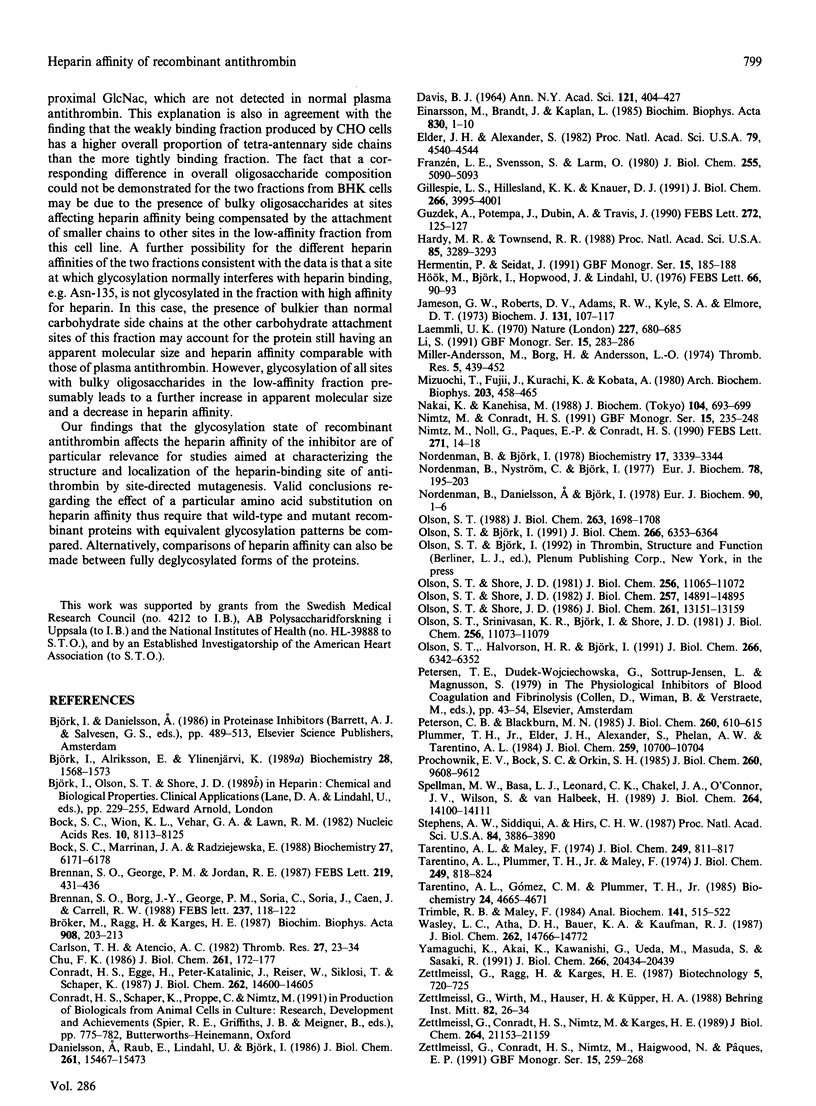
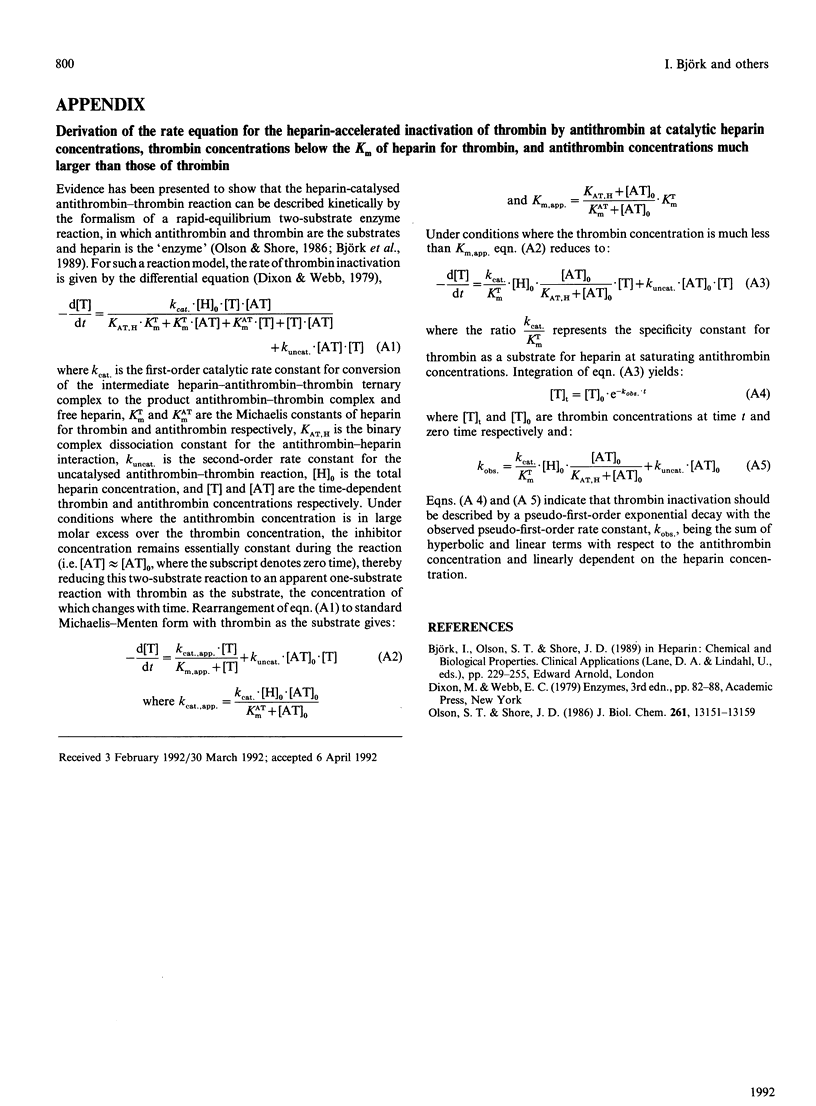
Images in this article
Selected References
These references are in PubMed. This may not be the complete list of references from this article.
- Björk I., Alriksson E., Ylinenjärvi K. Kinetics of binding of chicken cystatin to papain. Biochemistry. 1989 Feb 21;28(4):1568–1573. doi: 10.1021/bi00430a022. [DOI] [PubMed] [Google Scholar]
- Bock S. C., Marrinan J. A., Radziejewska E. Antithrombin III Utah: proline-407 to leucine mutation in a highly conserved region near the inhibitor reactive site. Biochemistry. 1988 Aug 9;27(16):6171–6178. doi: 10.1021/bi00416a052. [DOI] [PubMed] [Google Scholar]
- Bock S. C., Wion K. L., Vehar G. A., Lawn R. M. Cloning and expression of the cDNA for human antithrombin III. Nucleic Acids Res. 1982 Dec 20;10(24):8113–8125. doi: 10.1093/nar/10.24.8113. [DOI] [PMC free article] [PubMed] [Google Scholar]
- Brennan S. O., Borg J. Y., George P. M., Soria C., Soria J., Caen J., Carrell R. W. New carbohydrate site in mutant antithrombin (7 Ile----Asn) with decreased heparin affinity. FEBS Lett. 1988 Sep 12;237(1-2):118–122. doi: 10.1016/0014-5793(88)80183-2. [DOI] [PubMed] [Google Scholar]
- Brennan S. O., George P. M., Jordan R. E. Physiological variant of antithrombin-III lacks carbohydrate sidechain at Asn 135. FEBS Lett. 1987 Jul 27;219(2):431–436. doi: 10.1016/0014-5793(87)80266-1. [DOI] [PubMed] [Google Scholar]
- Bröker M., Ragg H., Karges H. E. Expression of human antithrombin III in Saccharomyces cerevisiae and Schizosaccharomyces pombe. Biochim Biophys Acta. 1987 Apr 29;908(3):203–213. doi: 10.1016/0167-4781(87)90100-x. [DOI] [PubMed] [Google Scholar]
- Carlson T. H., Atencio A. C. Isolation and partial characterization of two distinct types of antithrombin III from rabbit. Thromb Res. 1982 Jul 1;27(1):23–34. doi: 10.1016/0049-3848(82)90274-2. [DOI] [PubMed] [Google Scholar]
- Chu F. K. Requirements of cleavage of high mannose oligosaccharides in glycoproteins by peptide N-glycosidase F. J Biol Chem. 1986 Jan 5;261(1):172–177. [PubMed] [Google Scholar]
- Conradt H. S., Egge H., Peter-Katalinic J., Reiser W., Siklosi T., Schaper K. Structure of the carbohydrate moiety of human interferon-beta secreted by a recombinant Chinese hamster ovary cell line. J Biol Chem. 1987 Oct 25;262(30):14600–14605. [PubMed] [Google Scholar]
- DAVIS B. J. DISC ELECTROPHORESIS. II. METHOD AND APPLICATION TO HUMAN SERUM PROTEINS. Ann N Y Acad Sci. 1964 Dec 28;121:404–427. doi: 10.1111/j.1749-6632.1964.tb14213.x. [DOI] [PubMed] [Google Scholar]
- Danielsson A., Raub E., Lindahl U., Björk I. Role of ternary complexes, in which heparin binds both antithrombin and proteinase, in the acceleration of the reactions between antithrombin and thrombin or factor Xa. J Biol Chem. 1986 Nov 25;261(33):15467–15473. [PubMed] [Google Scholar]
- Einarsson M., Brandt J., Kaplan L. Large-scale purification of human tissue-type plasminogen activator using monoclonal antibodies. Biochim Biophys Acta. 1985 Jul 18;830(1):1–10. doi: 10.1016/0167-4838(85)90123-2. [DOI] [PubMed] [Google Scholar]
- Elder J. H., Alexander S. endo-beta-N-acetylglucosaminidase F: endoglycosidase from Flavobacterium meningosepticum that cleaves both high-mannose and complex glycoproteins. Proc Natl Acad Sci U S A. 1982 Aug;79(15):4540–4544. doi: 10.1073/pnas.79.15.4540. [DOI] [PMC free article] [PubMed] [Google Scholar]
- Franzén L. E., Svensson S., Larm O. Structural studies on the carbohydrate portion of human antithrombin III. J Biol Chem. 1980 Jun 10;255(11):5090–5093. [PubMed] [Google Scholar]
- Gillespie L. S., Hillesland K. K., Knauer D. J. Expression of biologically active human antithrombin III by recombinant baculovirus in Spodoptera frugiperda cells. J Biol Chem. 1991 Feb 25;266(6):3995–4001. [PubMed] [Google Scholar]
- Guzdek A., Potempa J., Dubin A., Travis J. Comparative properties of human alpha-1-proteinase inhibitor glycosylation variants. FEBS Lett. 1990 Oct 15;272(1-2):125–127. doi: 10.1016/0014-5793(90)80464-t. [DOI] [PubMed] [Google Scholar]
- Hardy M. R., Townsend R. R. Separation of positional isomers of oligosaccharides and glycopeptides by high-performance anion-exchange chromatography with pulsed amperometric detection. Proc Natl Acad Sci U S A. 1988 May;85(10):3289–3293. doi: 10.1073/pnas.85.10.3289. [DOI] [PMC free article] [PubMed] [Google Scholar]
- Hök M., Björk I., Hopwood J., Lindahl U. Anticoagulant activity of heparin: separation of high-activity and low-activity heparin species by affinity chromatography on immobilized antithrombin. FEBS Lett. 1976 Jul 1;66(1):90–93. doi: 10.1016/0014-5793(76)80592-3. [DOI] [PubMed] [Google Scholar]
- Jameson G. W., Roberts D. V., Adams R. W., Kyle W. S., Elmore D. T. Determination of the operational molarity of solutions of bovine alpha-chymotrypsin, trypsin, thrombin and factor Xa by spectrofluorimetric titration. Biochem J. 1973 Jan;131(1):107–117. doi: 10.1042/bj1310107. [DOI] [PMC free article] [PubMed] [Google Scholar]
- Laemmli U. K. Cleavage of structural proteins during the assembly of the head of bacteriophage T4. Nature. 1970 Aug 15;227(5259):680–685. doi: 10.1038/227680a0. [DOI] [PubMed] [Google Scholar]
- Mizuochi T., Fujii J., Kurachi K., Kobata A. Structural studies of the carbohydrate moiety of human antithrombin III. Arch Biochem Biophys. 1980 Aug;203(1):458–465. doi: 10.1016/0003-9861(80)90199-x. [DOI] [PubMed] [Google Scholar]
- Nakai K., Kanehisa M. Prediction of in-vivo modification sites of proteins from their primary structures. J Biochem. 1988 Nov;104(5):693–699. doi: 10.1093/oxfordjournals.jbchem.a122535. [DOI] [PubMed] [Google Scholar]
- Nimtz M., Noll G., Pâques E. P., Conradt H. S. Carbohydrate structures of a human tissue plasminogen activator variant expressed in recombinant Chinese hamster ovary cells. FEBS Lett. 1990 Oct 1;271(1-2):14–18. doi: 10.1016/0014-5793(90)80361-l. [DOI] [PubMed] [Google Scholar]
- Nordenman B., Björk I. Binding of low-affinity and high-affinity heparin to antithrombin. Ultraviolet difference spectroscopy and circular dichroism studies. Biochemistry. 1978 Aug 8;17(16):3339–3344. doi: 10.1021/bi00609a026. [DOI] [PubMed] [Google Scholar]
- Nordenman B., Danielsson A., Björk I. The binding of low-affinity and high-affinity heparin to antithrombin. Fluorescence studies. Eur J Biochem. 1978 Sep 15;90(1):1–6. doi: 10.1111/j.1432-1033.1978.tb12567.x. [DOI] [PubMed] [Google Scholar]
- Nordenman B., Nyström C., Björk I. The size and shape of human and bovine antithrombin III. Eur J Biochem. 1977 Aug 15;78(1):195–203. doi: 10.1111/j.1432-1033.1977.tb11730.x. [DOI] [PubMed] [Google Scholar]
- Olson S. T., Björk I. Predominant contribution of surface approximation to the mechanism of heparin acceleration of the antithrombin-thrombin reaction. Elucidation from salt concentration effects. J Biol Chem. 1991 Apr 5;266(10):6353–6364. [PubMed] [Google Scholar]
- Olson S. T., Halvorson H. R., Björk I. Quantitative characterization of the thrombin-heparin interaction. Discrimination between specific and nonspecific binding models. J Biol Chem. 1991 Apr 5;266(10):6342–6352. [PubMed] [Google Scholar]
- Olson S. T., Shore J. D. Binding of high affinity heparin to antithrombin III. Characterization of the protein fluorescence enhancement. J Biol Chem. 1981 Nov 10;256(21):11065–11072. [PubMed] [Google Scholar]
- Olson S. T., Shore J. D. Demonstration of a two-step reaction mechanism for inhibition of alpha-thrombin by antithrombin III and identification of the step affected by heparin. J Biol Chem. 1982 Dec 25;257(24):14891–14895. [PubMed] [Google Scholar]
- Olson S. T., Shore J. D. Transient kinetics of heparin-catalyzed protease inactivation by antithrombin III. The reaction step limiting heparin turnover in thrombin neutralization. J Biol Chem. 1986 Oct 5;261(28):13151–13159. [PubMed] [Google Scholar]
- Olson S. T., Shore J. D. Transient kinetics of heparin-catalyzed protease inactivation by antithrombin III. The reaction step limiting heparin turnover in thrombin neutralization. J Biol Chem. 1986 Oct 5;261(28):13151–13159. [PubMed] [Google Scholar]
- Olson S. T., Srinivasan K. R., Björk I., Shore J. D. Binding of high affinity heparin to antithrombin III. Stopped flow kinetic studies of the binding interaction. J Biol Chem. 1981 Nov 10;256(21):11073–11079. [PubMed] [Google Scholar]
- Olson S. T. Transient kinetics of heparin-catalyzed protease inactivation by antithrombin III. Linkage of protease-inhibitor-heparin interactions in the reaction with thrombin. J Biol Chem. 1988 Feb 5;263(4):1698–1708. [PubMed] [Google Scholar]
- Peterson C. B., Blackburn M. N. Isolation and characterization of an antithrombin III variant with reduced carbohydrate content and enhanced heparin binding. J Biol Chem. 1985 Jan 10;260(1):610–615. [PubMed] [Google Scholar]
- Plummer T. H., Jr, Elder J. H., Alexander S., Phelan A. W., Tarentino A. L. Demonstration of peptide:N-glycosidase F activity in endo-beta-N-acetylglucosaminidase F preparations. J Biol Chem. 1984 Sep 10;259(17):10700–10704. [PubMed] [Google Scholar]
- Prochownik E. V., Bock S. C., Orkin S. H. Intron structure of the human antithrombin III gene differs from that of other members of the serine protease inhibitor superfamily. J Biol Chem. 1985 Aug 15;260(17):9608–9612. [PubMed] [Google Scholar]
- Spellman M. W., Basa L. J., Leonard C. K., Chakel J. A., O'Connor J. V., Wilson S., van Halbeek H. Carbohydrate structures of human tissue plasminogen activator expressed in Chinese hamster ovary cells. J Biol Chem. 1989 Aug 25;264(24):14100–14111. [PubMed] [Google Scholar]
- Stephens A. W., Siddiqui A., Hirs C. H. Expression of functionally active human antithrombin III. Proc Natl Acad Sci U S A. 1987 Jun;84(11):3886–3890. doi: 10.1073/pnas.84.11.3886. [DOI] [PMC free article] [PubMed] [Google Scholar]
- Tarentino A. L., Gómez C. M., Plummer T. H., Jr Deglycosylation of asparagine-linked glycans by peptide:N-glycosidase F. Biochemistry. 1985 Aug 13;24(17):4665–4671. doi: 10.1021/bi00338a028. [DOI] [PubMed] [Google Scholar]
- Tarentino A. L., Maley F. Purification and properties of an endo-beta-N-acetylglucosaminidase from Streptomyces griseus. J Biol Chem. 1974 Feb 10;249(3):811–817. [PubMed] [Google Scholar]
- Tarentino A. L., Plummer T. H., Jr, Maley F. The release of intact oligosaccharides from specific glycoproteins by endo-beta-N-acetylglucosaminidase H. J Biol Chem. 1974 Feb 10;249(3):818–824. [PubMed] [Google Scholar]
- Trimble R. B., Maley F. Optimizing hydrolysis of N-linked high-mannose oligosaccharides by endo-beta-N-acetylglucosaminidase H. Anal Biochem. 1984 Sep;141(2):515–522. doi: 10.1016/0003-2697(84)90080-0. [DOI] [PubMed] [Google Scholar]
- Wasley L. C., Atha D. H., Bauer K. A., Kaufman R. J. Expression and characterization of human antithrombin III synthesized in mammalian cells. J Biol Chem. 1987 Oct 25;262(30):14766–14772. [PubMed] [Google Scholar]
- Yamaguchi K., Akai K., Kawanishi G., Ueda M., Masuda S., Sasaki R. Effects of site-directed removal of N-glycosylation sites in human erythropoietin on its production and biological properties. J Biol Chem. 1991 Oct 25;266(30):20434–20439. [PubMed] [Google Scholar]
- Zettlmeissl G., Conradt H. S., Nimtz M., Karges H. E. Characterization of recombinant human antithrombin III synthesized in Chinese hamster ovary cells. J Biol Chem. 1989 Dec 15;264(35):21153–21159. [PubMed] [Google Scholar]
- Zettlmeissl G., Wirth M., Hauser H., Küpper H. A. Efficient expression system for human antithrombin III in baby hamster kidney cells. Behring Inst Mitt. 1988 Apr;(82):26–34. [PubMed] [Google Scholar]