Abstract
The effects of pancreatic hormones and cyclic AMP on the induction of ketogenesis and long-chain fatty acid oxidation were studied in primary cultures of hepatocytes from fetal and newborn rabbits. Hepatocytes were cultivated during 4 days in the presence of glucagon (10(-6) M), forskolin (2 x 10(-5) M), dibutyryl cyclic AMP (10(-4) M), 8-bromo cyclic AMP (10(-4) M) or insulin (10(-7) M). Ketogenesis and fatty acid metabolism were measured using [1-14C]oleate (0.5 mM). In hepatocytes from fetuses at term, the rate of ketogenesis remained very low during the 4 days of culture. In hepatocytes from 24-h-old newborn, the rate of ketogenesis was high during the first 48 h of culture and then rapidly decreased to reach a low value similar to that measured in cultured hepatocytes from term fetuses. A 48 h exposure to glucagon, forskolin or cyclic AMP derivatives is necessary to induce ketone body production in cultured fetal hepatocytes at a rate similar to that found in cultured hepatocytes from newborn rabbits. In fetal liver cells, the induction of ketogenesis by glucagon or cyclic AMP results from changes in the partitioning of long-chain fatty acid from esterification towards oxidation. Indeed, glucagon, forskolin and cyclic AMP enhance oleate oxidation (basal, 12.7 +/- 1.6; glucagon, 50.0 +/- 5.5; forskolin, 70.6 +/- 5.4; cyclic AMP, 77.5 +/- 3.4% of oleate metabolized) at the expense of oleate esterification. In cultured fetal hepatocytes, the rate of fatty acid oxidation in the presence of cyclic AMP is similar to the rate of oleate oxidation present at the time of plating (85.1 +/- 2.6% of oleate metabolized) in newborn rabbit hepatocytes. In hepatocytes from term fetuses, the presence of insulin antagonizes in a dose-dependent fashion the glucagon-induced oleate oxidation. Neither glucagon nor cyclic AMP affect the activity of carnitine palmitoyltransferase I (CPT I). The malonyl-CoA concentration inducing 50% inhibition of CPT I (IC50) is 14-fold higher in mitochondria isolated from cultured newborn hepatocytes (0.95 microM) compared with fetal hepatocytes (0.07 microM), indicating that the sensitivity of CPT I decreases markedly in the first 24 h after birth. The addition of glucagon or cyclic AMP into cultured fetal hepatocytes decreased by 80% and 90% respectively the sensitivity of CPT I to malonyl-CoA inhibition. In the presence of cyclic AMP, the sensitivity of CPT I to malonyl-CoA inhibition in cultured fetal hepatocytes is very similar to that measured in cultured hepatocytes from 24-h-old newborns.
Full text
PDF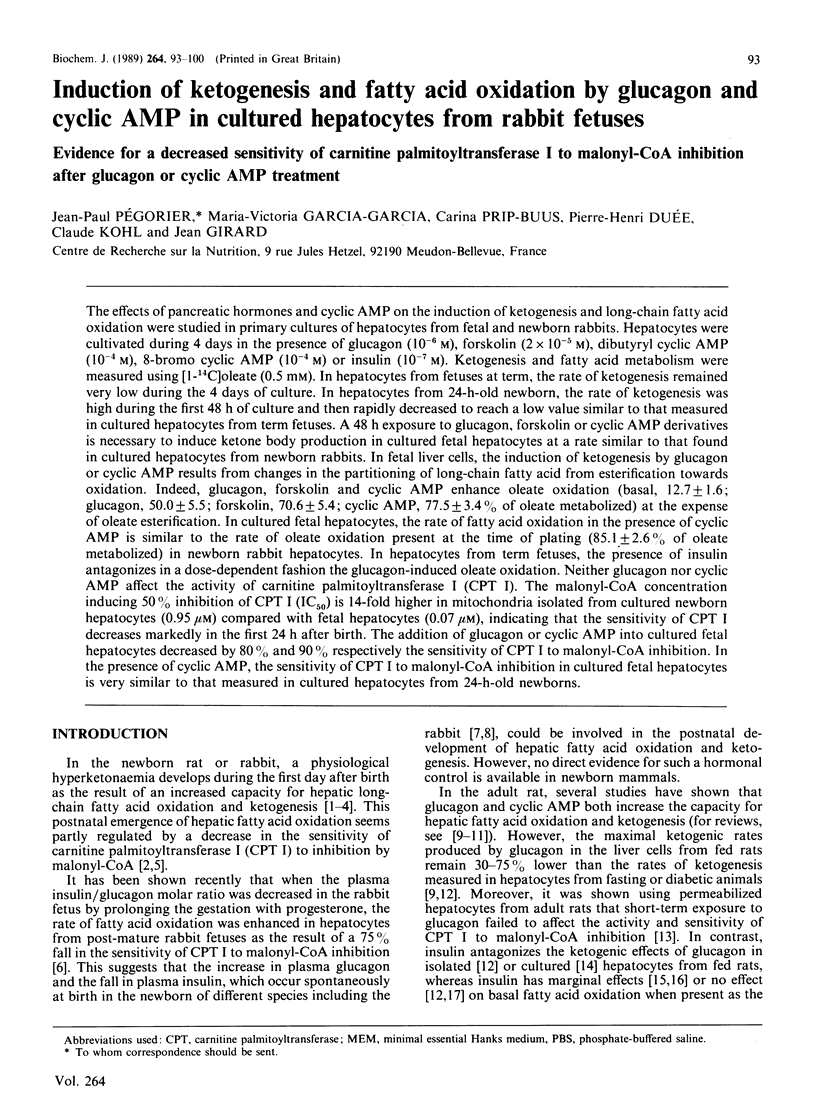
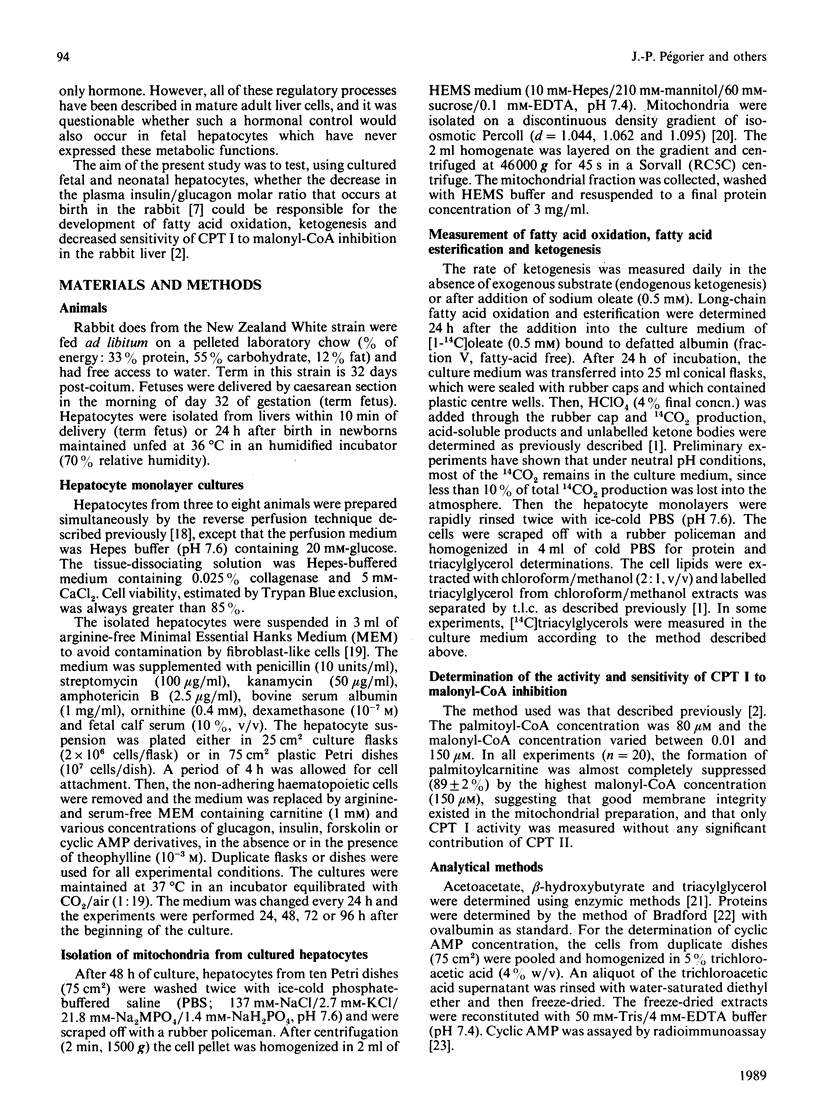
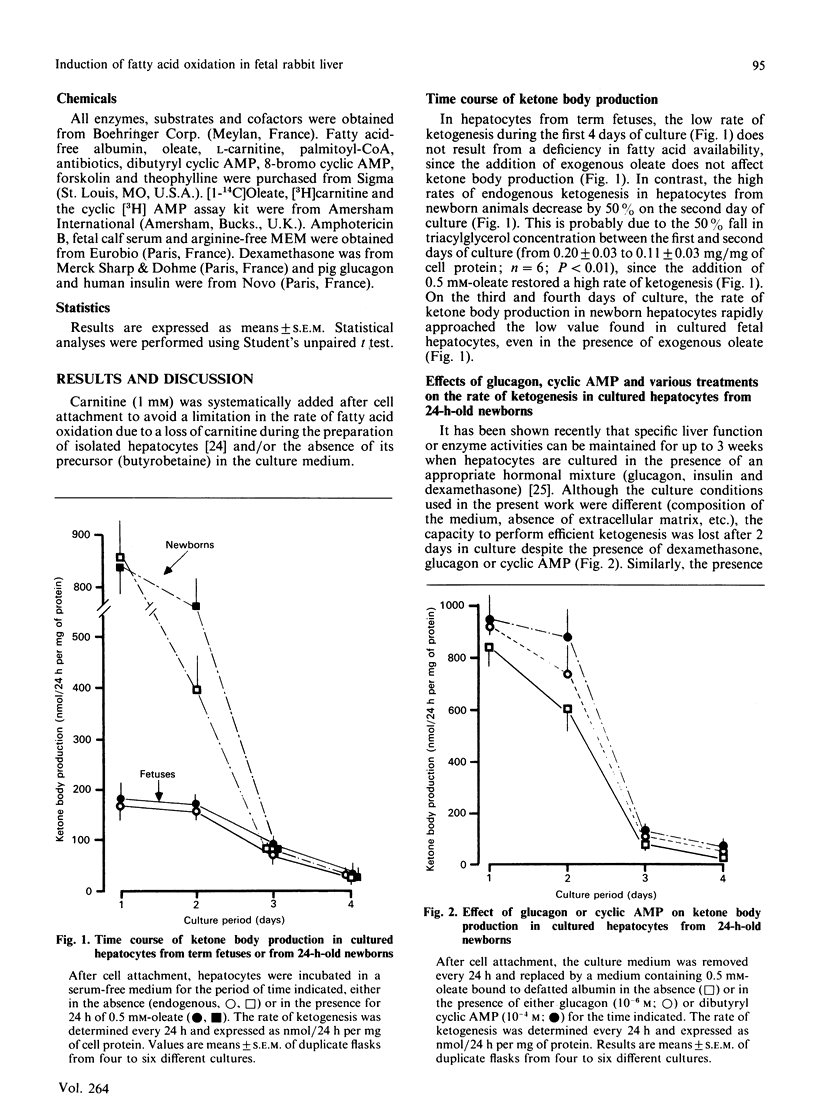
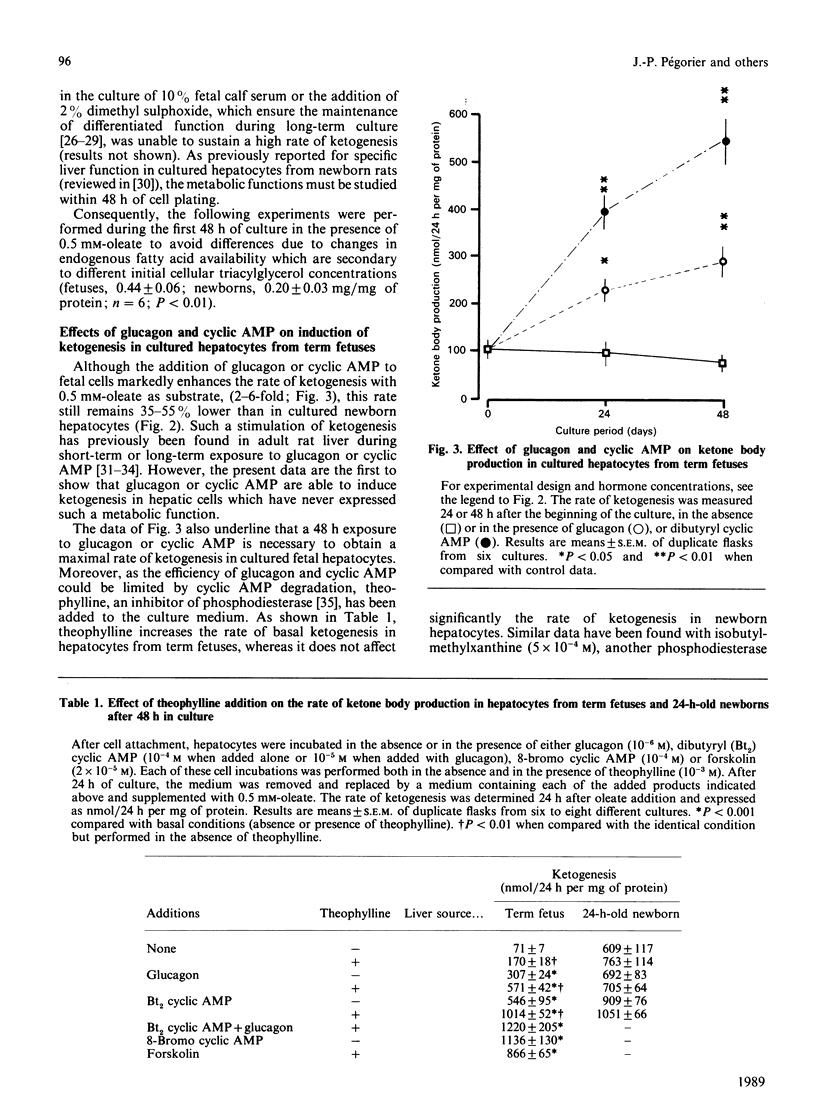
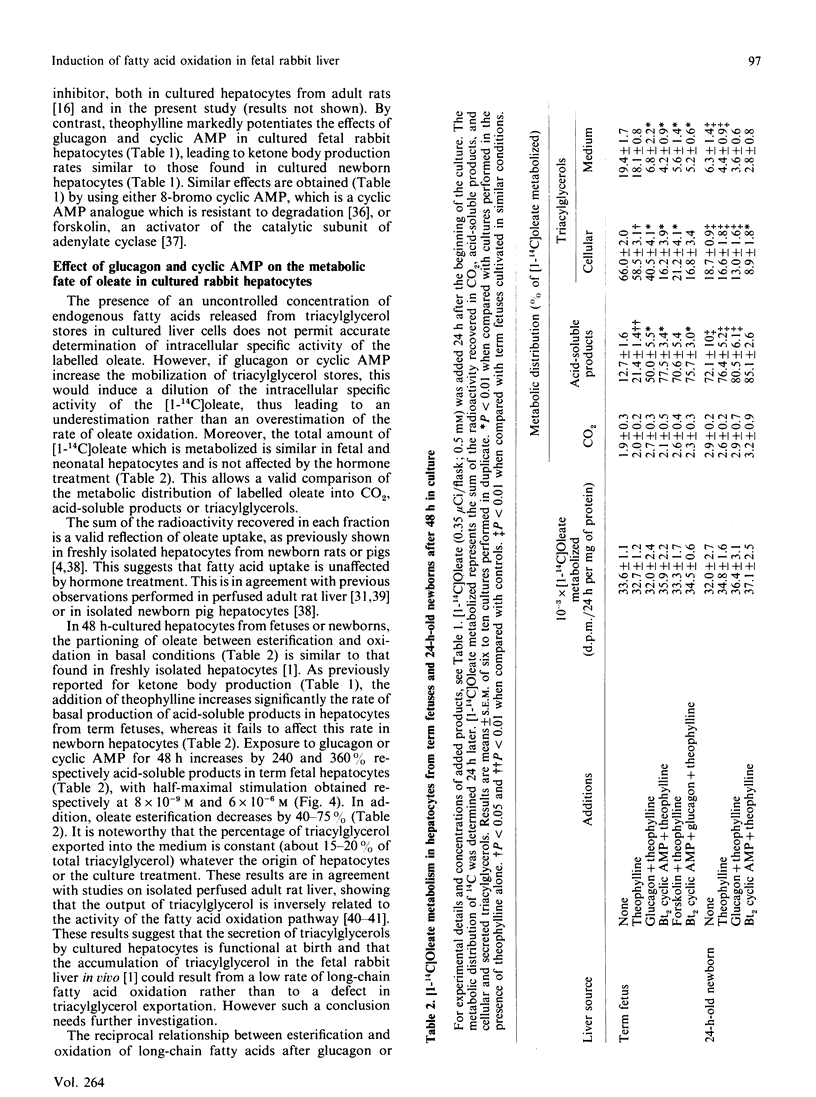
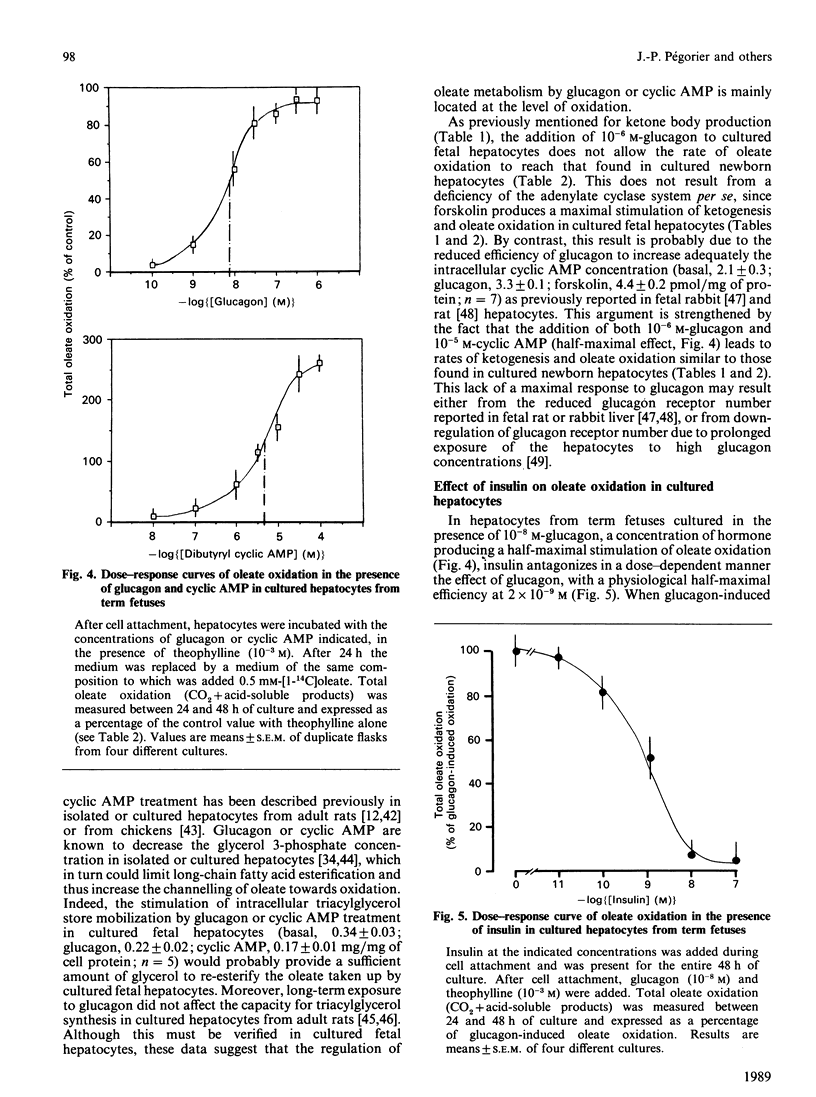
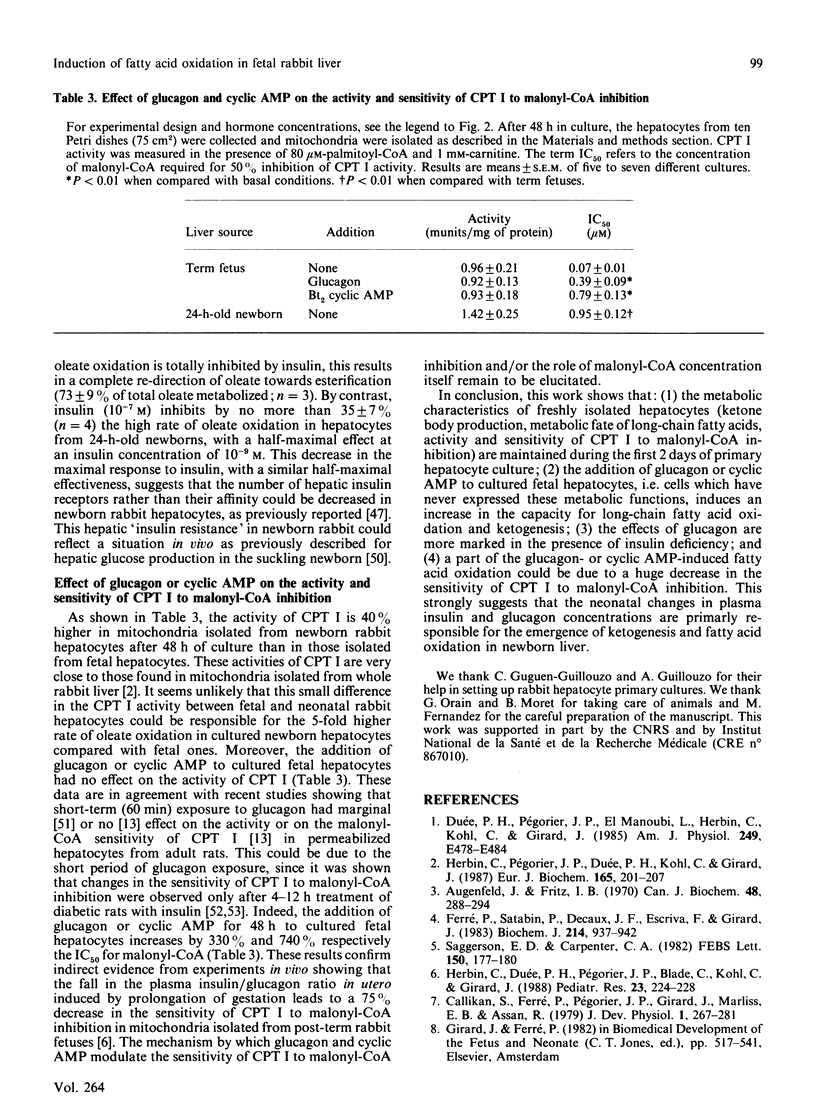
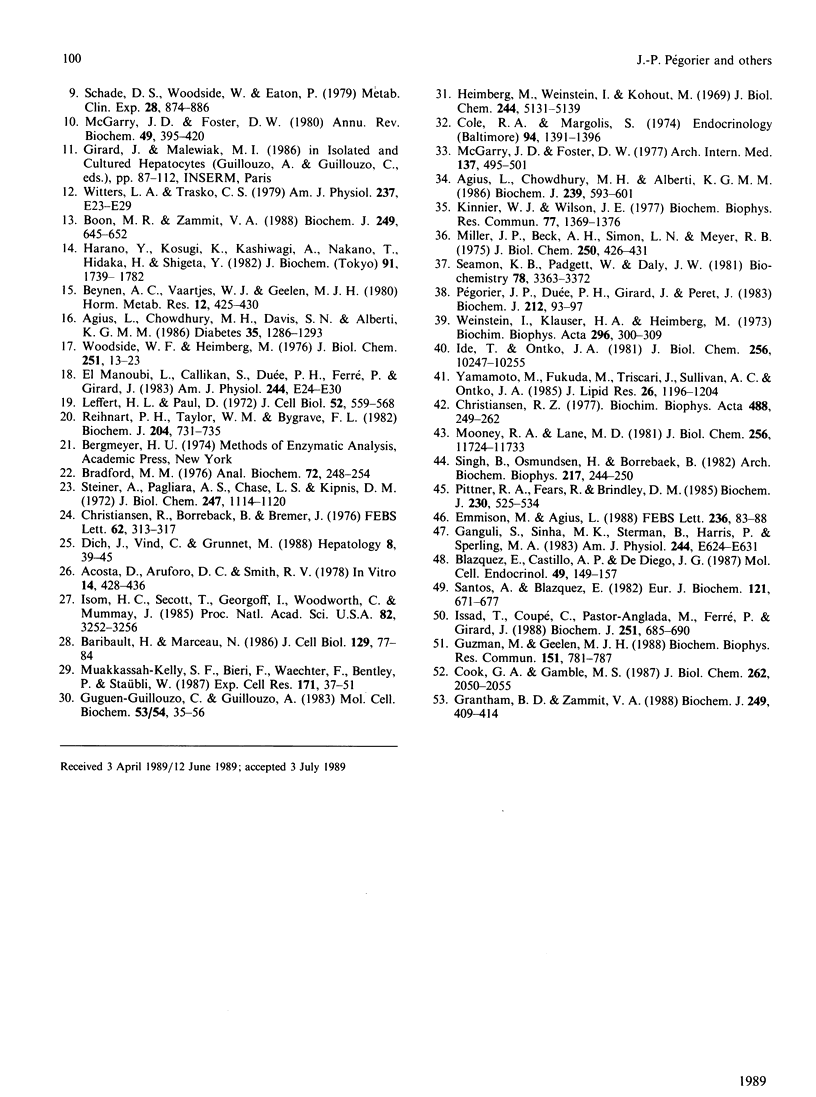
Selected References
These references are in PubMed. This may not be the complete list of references from this article.
- Acosta D., Anuforo D. C., Smith R. V. Primary monolayer cultures of postnatal rat liver cells with extended differentiated functions. In Vitro. 1978 May;14(5):428–436. doi: 10.1007/BF02616104. [DOI] [PubMed] [Google Scholar]
- Agius L., Chowdhury M. H., Alberti K. G. Regulation of ketogenesis, gluconeogenesis and the mitochondrial redox state by dexamethasone in hepatocyte monolayer cultures. Biochem J. 1986 Nov 1;239(3):593–601. doi: 10.1042/bj2390593. [DOI] [PMC free article] [PubMed] [Google Scholar]
- Agius L., Chowdhury M. H., Davis S. N., Alberti K. G. Regulation of ketogenesis, gluconeogenesis, and glycogen synthesis by insulin and proinsulin in rat hepatocyte monolayer cultures. Diabetes. 1986 Nov;35(11):1286–1293. doi: 10.2337/diab.35.11.1286. [DOI] [PubMed] [Google Scholar]
- Augenfeld J., Fritz I. B. Carnitine palmitolyltransferase activity and fatty acid oxidation by livers from fetal and neonatal rats. Can J Biochem. 1970 Mar;48(3):288–294. doi: 10.1139/o70-050. [DOI] [PubMed] [Google Scholar]
- Baribault H., Marceau N. Dexamethasone and dimethylsulfoxide as distinct regulators of growth and differentiation of cultured suckling rat hepatocytes. J Cell Physiol. 1986 Oct;129(1):77–84. doi: 10.1002/jcp.1041290112. [DOI] [PubMed] [Google Scholar]
- Beynen A. C., Vaartjes W. J., Geelen M. J. Acute effects of insulin on fatty acid metabolism in isolated rat hepatocytes. Horm Metab Res. 1980 Sep;12(9):425–430. doi: 10.1055/s-2007-999166. [DOI] [PubMed] [Google Scholar]
- Blázquez E., Perez Castillo A., de Diego J. G. Characterization of glucagon receptors in liver membranes and isolated hepatocytes during rat ontogenic development. Mol Cell Endocrinol. 1987 Feb;49(2-3):149–157. doi: 10.1016/0303-7207(87)90208-5. [DOI] [PubMed] [Google Scholar]
- Boon M. R., Zammit V. A. Use of a selectively permeabilized isolated rat hepatocyte preparation to study changes in the properties of overt carnitine palmitoyltransferase activity in situ. Biochem J. 1988 Feb 1;249(3):645–652. doi: 10.1042/bj2490645. [DOI] [PMC free article] [PubMed] [Google Scholar]
- Bradford M. M. A rapid and sensitive method for the quantitation of microgram quantities of protein utilizing the principle of protein-dye binding. Anal Biochem. 1976 May 7;72:248–254. doi: 10.1006/abio.1976.9999. [DOI] [PubMed] [Google Scholar]
- Callikan S., Ferre P., Pegorier J. P., Girard J. R., Marliss E. B., Assan R. Fuel metabolism in fasted newborn rabbits. J Dev Physiol. 1979 Aug;1(4):267–281. [PubMed] [Google Scholar]
- Christiansen R. Z. Regulation of palmitate metabolism by carnitine and glucagon in hepatocytes isolated from fasted and carbohydrate refed rats. Biochim Biophys Acta. 1977 Aug 24;488(2):249–262. doi: 10.1016/0005-2760(77)90182-5. [DOI] [PubMed] [Google Scholar]
- Christiansen R., Borrebaek B., Bremer J. The effect of (-)carnitine on the metabolism of palmitate in liver cells isolated from fasted and refed rats. FEBS Lett. 1976 Mar 1;62(3):313–317. doi: 10.1016/0014-5793(76)80083-x. [DOI] [PubMed] [Google Scholar]
- Cole R. A., Margolis S. Stimulation of ketogenesis by dibutyryl cyclic AMP in isolated rat hepatocytes. Endocrinology. 1974 May;94(5):1391–1396. doi: 10.1210/endo-94-5-1391. [DOI] [PubMed] [Google Scholar]
- Cook G. A., Gamble M. S. Regulation of carnitine palmitoyltransferase by insulin results in decreased activity and decreased apparent Ki values for malonyl-CoA. J Biol Chem. 1987 Feb 15;262(5):2050–2055. [PubMed] [Google Scholar]
- Dich J., Vind C., Grunnet N. Long-term culture of hepatocytes: effect of hormones on enzyme activities and metabolic capacity. Hepatology. 1988 Jan-Feb;8(1):39–45. doi: 10.1002/hep.1840080109. [DOI] [PubMed] [Google Scholar]
- Duee P. H., Pegorier J. P., el Manoubi L., Herbin C., Kohl C., Girard J. Hepatic triglyceride hydrolysis and development of ketogenesis in rabbits. Am J Physiol. 1985 Nov;249(5 Pt 1):E478–E484. doi: 10.1152/ajpendo.1985.249.5.E478. [DOI] [PubMed] [Google Scholar]
- El Manoubi L., Callikan S., Duee P. H., Ferre P., Girard J. Development of gluconeogenesis in isolated hepatocytes from the rabbit. Am J Physiol. 1983 Jan;244(1):E24–E30. doi: 10.1152/ajpendo.1983.244.1.E24. [DOI] [PubMed] [Google Scholar]
- Emmison N., Agius L. Fatty acid uptake and metabolism to ketone bodies and triacyglycerol in rat and human hepatocyte cultures is dependent on chain-length and degree of saturation. Effects of carnitine and glucagon. FEBS Lett. 1988 Aug 15;236(1):83–88. doi: 10.1016/0014-5793(88)80289-8. [DOI] [PubMed] [Google Scholar]
- Ferré P., Satabin P., Decaux J. F., Escriva F., Girard J. Development and regulation of ketogenesis in hepatocytes isolated from newborn rats. Biochem J. 1983 Sep 15;214(3):937–942. doi: 10.1042/bj2140937. [DOI] [PMC free article] [PubMed] [Google Scholar]
- Ganguli S., Sinha M. K., Sterman B., Harris P., Sperling M. A. Ontogeny of hepatic insulin and glucagon receptors and adenylate cyclase in rabbit. Am J Physiol. 1983 Jun;244(6):E624–E631. doi: 10.1152/ajpendo.1983.244.6.E624. [DOI] [PubMed] [Google Scholar]
- Grantham B. D., Zammit V. A. Role of carnitine palmitoyltransferase I in the regulation of hepatic ketogenesis during the onset and reversal of chronic diabetes. Biochem J. 1988 Jan 15;249(2):409–414. doi: 10.1042/bj2490409. [DOI] [PMC free article] [PubMed] [Google Scholar]
- Guguen-Guillouzo C., Guillouzo A. Modulation of functional activities in cultured rat hepatocytes. Mol Cell Biochem. 1983;53-54(1-2):35–56. doi: 10.1007/BF00225245. [DOI] [PubMed] [Google Scholar]
- Guzmán M., Geelen M. J. Short-term regulation of carnitine palmitoyltransferase activity in isolated rat hepatocytes. Biochem Biophys Res Commun. 1988 Mar 15;151(2):781–787. doi: 10.1016/s0006-291x(88)80349-8. [DOI] [PubMed] [Google Scholar]
- Harano Y., Kosugi K., Kashiwagi A., Nakano T., Hidaka H., Shigeta Y. Regulatory mechanism of ketogenesis by glucagon and insulin in isolated and cultured hepatocytes. J Biochem. 1982 May;91(5):1739–1748. doi: 10.1093/oxfordjournals.jbchem.a133866. [DOI] [PubMed] [Google Scholar]
- Heimberg M., Weinstein I., Kohout M. The effects of glucagon, dibutyryl cyclic adenosine 3',5'-monophosphate, and concentration of free fatty acid on hepatic lipid metabolism. J Biol Chem. 1969 Oct 10;244(19):5131–5139. [PubMed] [Google Scholar]
- Herbin C., Duée P. H., Pégorier J. P., Bladé C., Kohl C., Girard J. Premature appearance of gluconeogenesis and fatty acid oxidation in the liver of the postterm rabbit fetus. Pediatr Res. 1988 Feb;23(2):224–228. doi: 10.1203/00006450-198802000-00019. [DOI] [PubMed] [Google Scholar]
- Herbin C., Pegorier J. P., Duee P. H., Kohl C., Girard J. Regulation of fatty acid oxidation in isolated hepatocytes and liver mitochondria from newborn rabbits. Eur J Biochem. 1987 May 15;165(1):201–207. doi: 10.1111/j.1432-1033.1987.tb11212.x. [DOI] [PubMed] [Google Scholar]
- Ide T., Ontko J. A. Increased secretion of very low density lipoprotein triglyceride following inhibition of long chain fatty acid oxidation in isolated rat liver. J Biol Chem. 1981 Oct 25;256(20):10247–10255. [PubMed] [Google Scholar]
- Isom H. C., Secott T., Georgoff I., Woodworth C., Mummaw J. Maintenance of differentiated rat hepatocytes in primary culture. Proc Natl Acad Sci U S A. 1985 May;82(10):3252–3256. doi: 10.1073/pnas.82.10.3252. [DOI] [PMC free article] [PubMed] [Google Scholar]
- Issad T., Coupé C., Pastor-Anglada M., Ferré P., Girard J. Development of insulin-sensitivity at weaning in the rat. Role of the nutritional transition. Biochem J. 1988 May 1;251(3):685–690. doi: 10.1042/bj2510685. [DOI] [PMC free article] [PubMed] [Google Scholar]
- Kinnier W. J., Wilson J. E. Effects of some inhibitiors of cyclic nucleotide phosphodiesterase on protein phosphorylation in isolated neurons and glia from rat brain. Biochem Biophys Res Commun. 1977 Aug 22;77(4):1369–1376. doi: 10.1016/s0006-291x(77)80130-7. [DOI] [PubMed] [Google Scholar]
- Leffert H. L., Paul D. Studies on primary cultures of differentiated fetal liver cells. J Cell Biol. 1972 Mar;52(3):559–568. doi: 10.1083/jcb.52.3.559. [DOI] [PMC free article] [PubMed] [Google Scholar]
- McGarry J. D., Foster D. W. Hormonal control of ketogenesis. Biochemical considerations. Arch Intern Med. 1977 Apr;137(4):495–501. [PubMed] [Google Scholar]
- McGarry J. D., Foster D. W. Regulation of hepatic fatty acid oxidation and ketone body production. Annu Rev Biochem. 1980;49:395–420. doi: 10.1146/annurev.bi.49.070180.002143. [DOI] [PubMed] [Google Scholar]
- Miller J. P., Beck A. H., Simon L. N., Meyer R. B., Jr Induction of hepatic tyrosine aminotransferase in vivo by derivatives of cyclic adenosine 3':5'-monophosphate. J Biol Chem. 1975 Jan 25;250(2):426–431. [PubMed] [Google Scholar]
- Mooney R. A., Lane M. D. Formation and turnover of triglyceride-rich vesicles in the chick liver cell. Effects of cAMP and carnitine on triglyceride mobilization and conversion to ketones. J Biol Chem. 1981 Nov 25;256(22):11724–11733. [PubMed] [Google Scholar]
- Muakkassah-Kelly S. F., Bieri F., Waechter F., Bentley P., Stäubli W. Long-term maintenance of hepatocytes in primary culture in the presence of DMSO: further characterization and effect of nafenopin, a peroxisome proliferator. Exp Cell Res. 1987 Jul;171(1):37–51. doi: 10.1016/0014-4827(87)90249-7. [DOI] [PubMed] [Google Scholar]
- Pittner R. A., Fears R., Brindley D. N. Interactions of insulin, glucagon and dexamethasone in controlling the activity of glycerol phosphate acyltransferase and the activity and subcellular distribution of phosphatidate phosphohydrolase in cultured rat hepatocytes. Biochem J. 1985 Sep 1;230(2):525–534. doi: 10.1042/bj2300525. [DOI] [PMC free article] [PubMed] [Google Scholar]
- Pégorier J. P., Duée P. H., Girard J., Peret J. Metabolic fate of non-esterified fatty acids in isolated hepatocytes from newborn and young pigs. Evidence for a limited capacity for oxidation and increased capacity for esterification. Biochem J. 1983 Apr 15;212(1):93–97. doi: 10.1042/bj2120093. [DOI] [PMC free article] [PubMed] [Google Scholar]
- Reinhart P. H., Taylor W. M., Bygrave F. L. A procedure for the rapid preparation of mitochondria from rat liver. Biochem J. 1982 Jun 15;204(3):731–735. doi: 10.1042/bj2040731. [DOI] [PMC free article] [PubMed] [Google Scholar]
- Saggerson E. D., Carpenter C. A. Regulation of hepatic carnitine palmitoyltransferase activity during the foetal-neonatal transition. FEBS Lett. 1982 Dec 13;150(1):177–180. doi: 10.1016/0014-5793(82)81329-x. [DOI] [PubMed] [Google Scholar]
- Santos A., Blazquez E. Direct evidence of a glucagon-dependent regulation of the concentration of glucagon receptors in the liver. Eur J Biochem. 1982 Jan;121(3):671–677. doi: 10.1111/j.1432-1033.1982.tb05838.x. [DOI] [PubMed] [Google Scholar]
- Schade D. S., Woodside W., Eaton R. P. The role of glucagon in the regulation of plasma lipids. Metabolism. 1979 Aug;28(8):874–886. doi: 10.1016/0026-0495(79)90215-4. [DOI] [PubMed] [Google Scholar]
- Seamon K. B., Padgett W., Daly J. W. Forskolin: unique diterpene activator of adenylate cyclase in membranes and in intact cells. Proc Natl Acad Sci U S A. 1981 Jun;78(6):3363–3367. doi: 10.1073/pnas.78.6.3363. [DOI] [PMC free article] [PubMed] [Google Scholar]
- Singh B., Osmundsen H., Borrebaek B. The time course of glucagon action on the utilization of [U-14C]palmitate by isolated hepatocytes. Arch Biochem Biophys. 1982 Aug;217(1):244–250. doi: 10.1016/0003-9861(82)90499-4. [DOI] [PubMed] [Google Scholar]
- Steiner A. L., Pagliara A. S., Chase L. R., Kipnis D. M. Radioimmunoassay for cyclic nucleotides. II. Adenosine 3',5'-monophosphate and guanosine 3',5'-monophosphate in mammalian tissues and body fluids. J Biol Chem. 1972 Feb 25;247(4):1114–1120. [PubMed] [Google Scholar]
- Weinstein I., Klausner H. A., Heimberg M. The effect of concentration of glucagon on output of triglyceride, ketone bodies, glucose, and urea by the liver. Biochim Biophys Acta. 1973 Feb 14;296(2):300–309. doi: 10.1016/0005-2760(73)90088-x. [DOI] [PubMed] [Google Scholar]
- Witters L. A., Trasko C. S. Regulation of hepatic free fatty acid metabolism by glucagon and insulin. Am J Physiol. 1979 Jul;237(1):E23–E29. doi: 10.1152/ajpendo.1979.237.1.E23. [DOI] [PubMed] [Google Scholar]
- Woodside W. F., Heimberg M. Effects of anti-insulin serum, insulin, and glucose on output of triglycerides and on ketogenesis by the perfused rat liver. J Biol Chem. 1976 Jan 10;251(1):13–23. [PubMed] [Google Scholar]
- Yamamoto M., Fukuda N., Triscari J., Sullivan A. C., Ontko J. A. Decreased hepatic production of very low density lipoproteins following activation of fatty acid oxidation by Ro 22-0654. J Lipid Res. 1985 Oct;26(10):1196–1204. [PubMed] [Google Scholar]