Abstract
1. Human erythrocytes were incubated in autologous plasma containing [32P]Pi, and sampled by a method which avoids washing the cells. 2. In experiments of up to 3 h duration, the specific radioactivity of cellular Pi stabilized at a value below that of extracellular Pi. This can be explained on the basis of a single cellular Pi pool exchanging with a large unlabelled pool of cellular organic phosphates. 3. However, a rapid initial phase of labelling, occurring within 30 s, was inconsistent with the situation described in point 2. A possible explanation is that about 1/4 of cellular Pi occurs in a separate, fast-labelling pool. 4. When the extracellular Pi concentration was doubled, most of the corresponding increase in the steady-state cellular Pi concentration was accounted for by the apparent fast-labelling Pi pool, which also doubled. 5. The observed initial rate of labelling of cellular organic phosphates [which probably occurs through the reaction catalysed by glyceraldehyde-3-phosphate dehydrogenase (E.C. 1.2.1.12)] was considerably lower than that predicted from the flux through the Embden-Meyerhof pathway. This implies that the enzyme is exposed to Pi whose specific radioactivity is lower than the mean specific radioactivity of cellular Pi, and fails to support earlier suggestions that this enzyme uses extracellular Pi. 6. In 3 h incubations, the rate of organic phosphate labelling was roughly constant throughout, even though the specific radioactivity of cellular Pi had risen slowly to a plateau. Viewed in conjunction with point 5, this again suggests some inhomogeneity in cellular Pi. 7. Cellular Pi and extracellular Pi only reached isotopic steady state after 2 days. At this stage some organic phosphates were probably still incompletely labelled. 8. We conclude that, whatever their physical or technical reasons, such labelling inhomogeneities and slow attainment of isotopic steady state may cause serious misinterpretation of results if ignored during 32P-labelling of intact cells.
Full text
PDF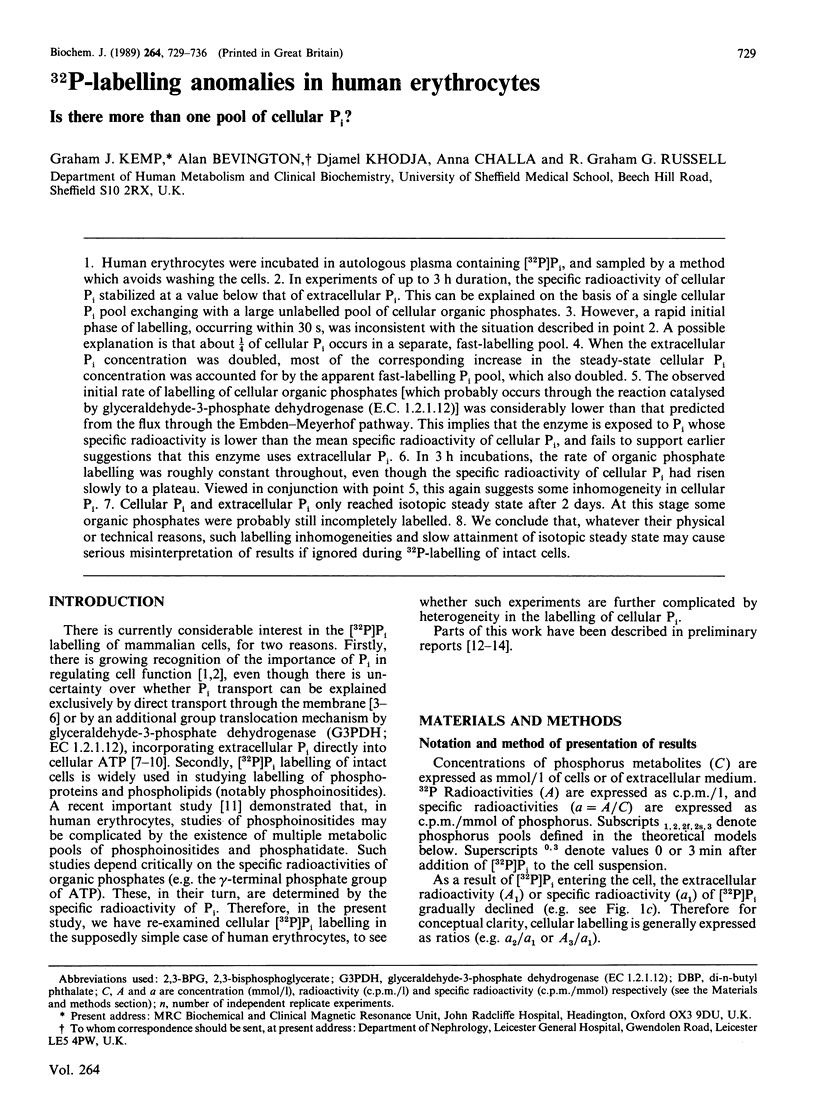
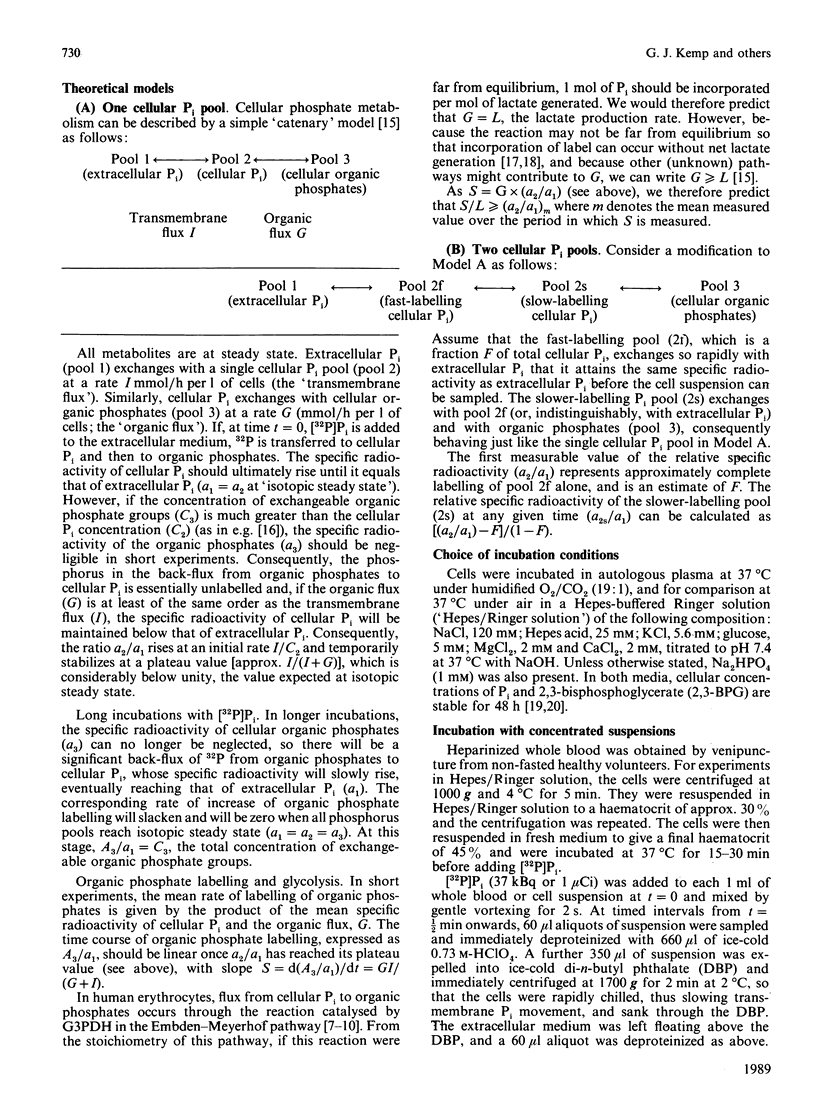
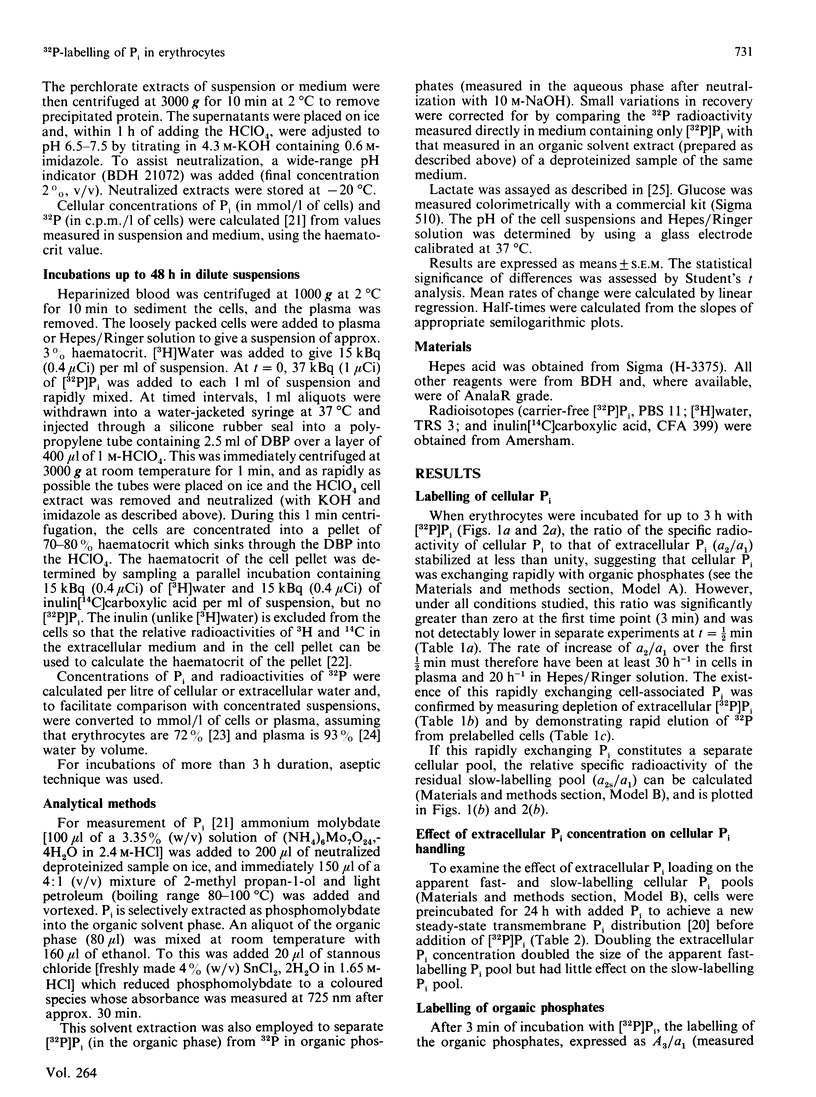
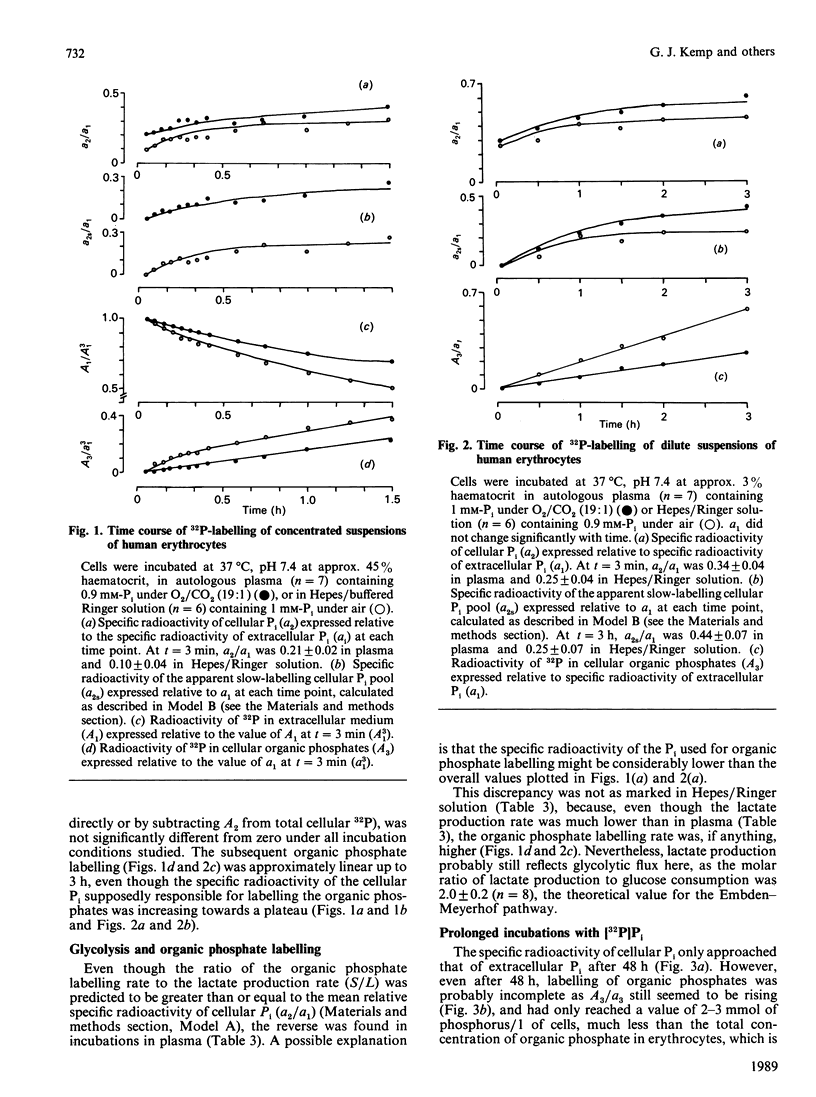
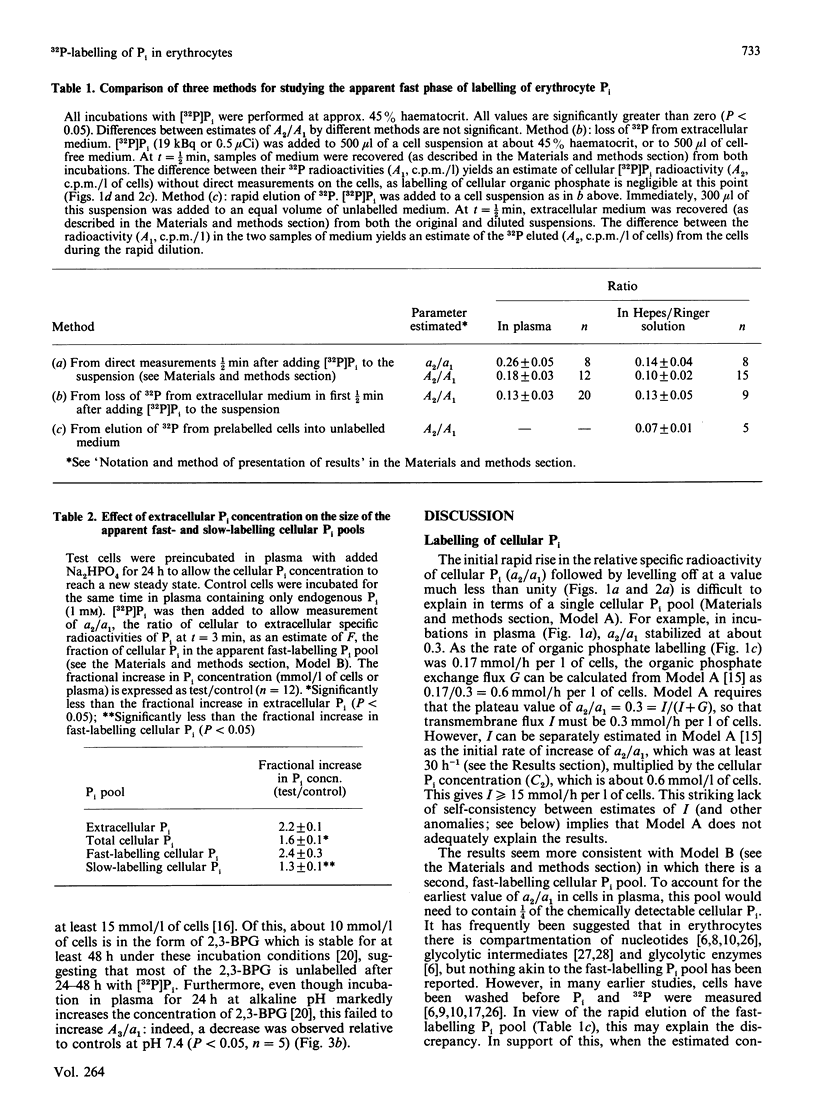
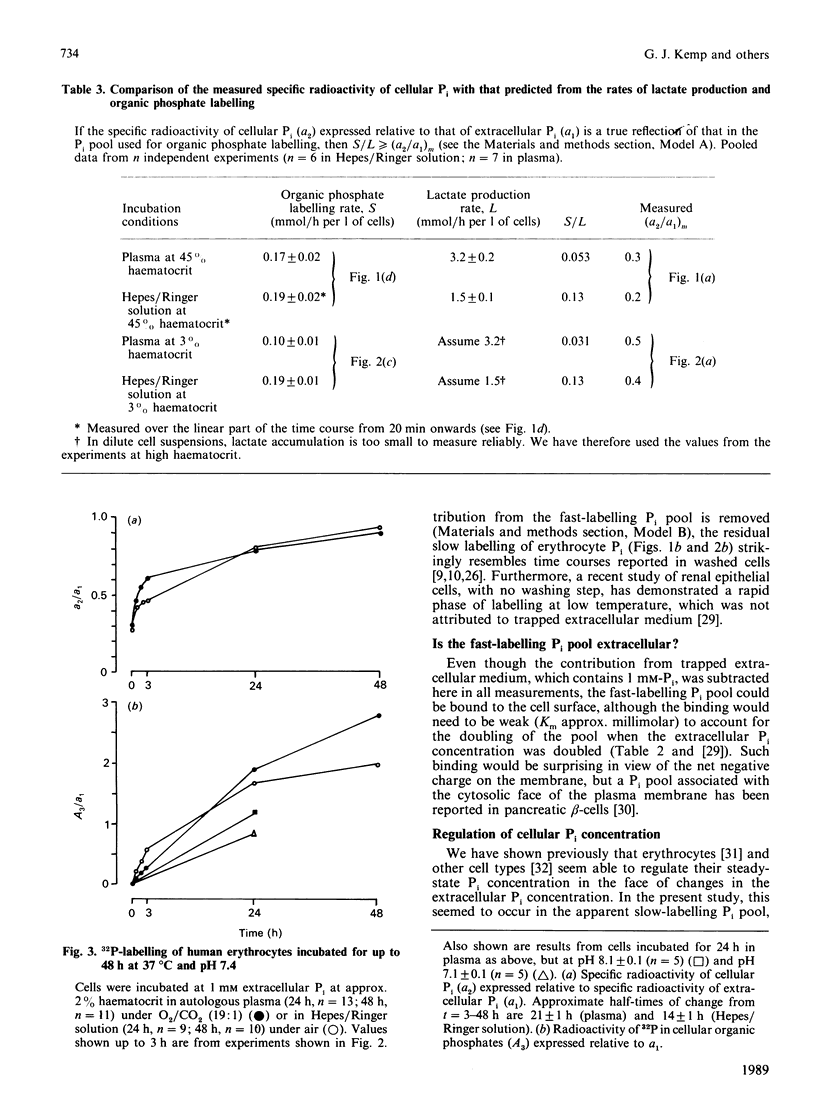
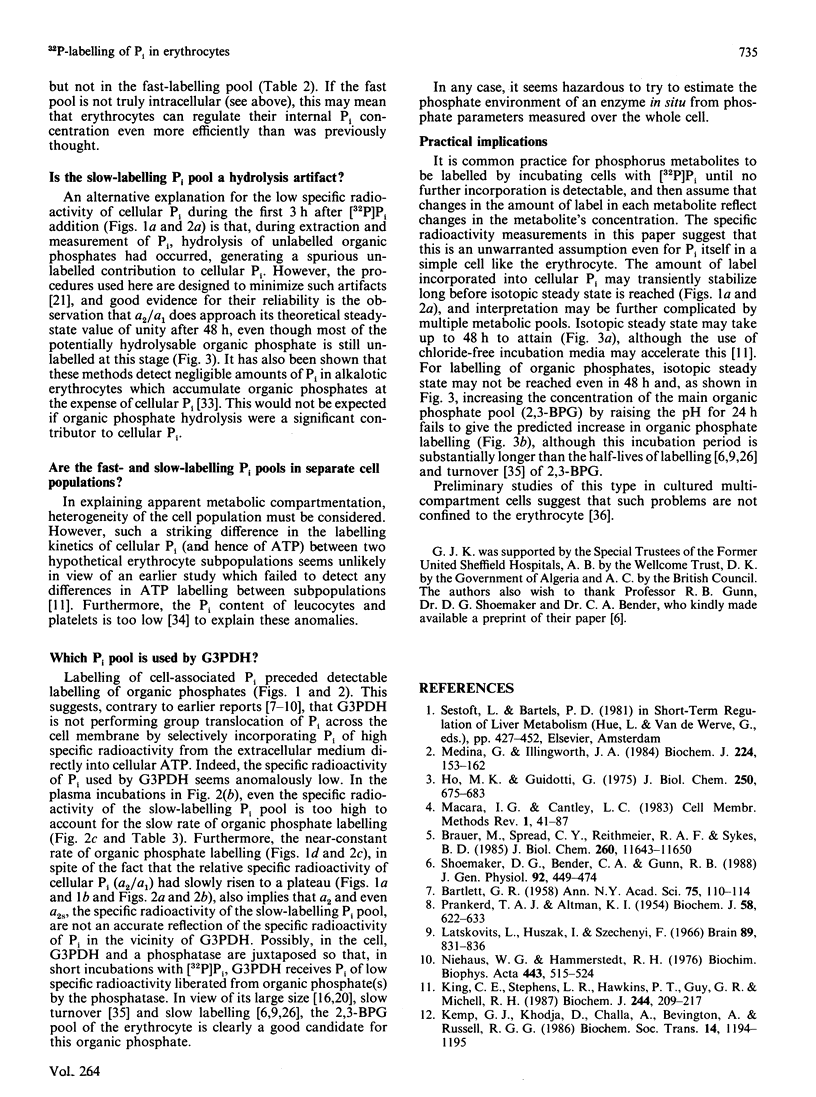
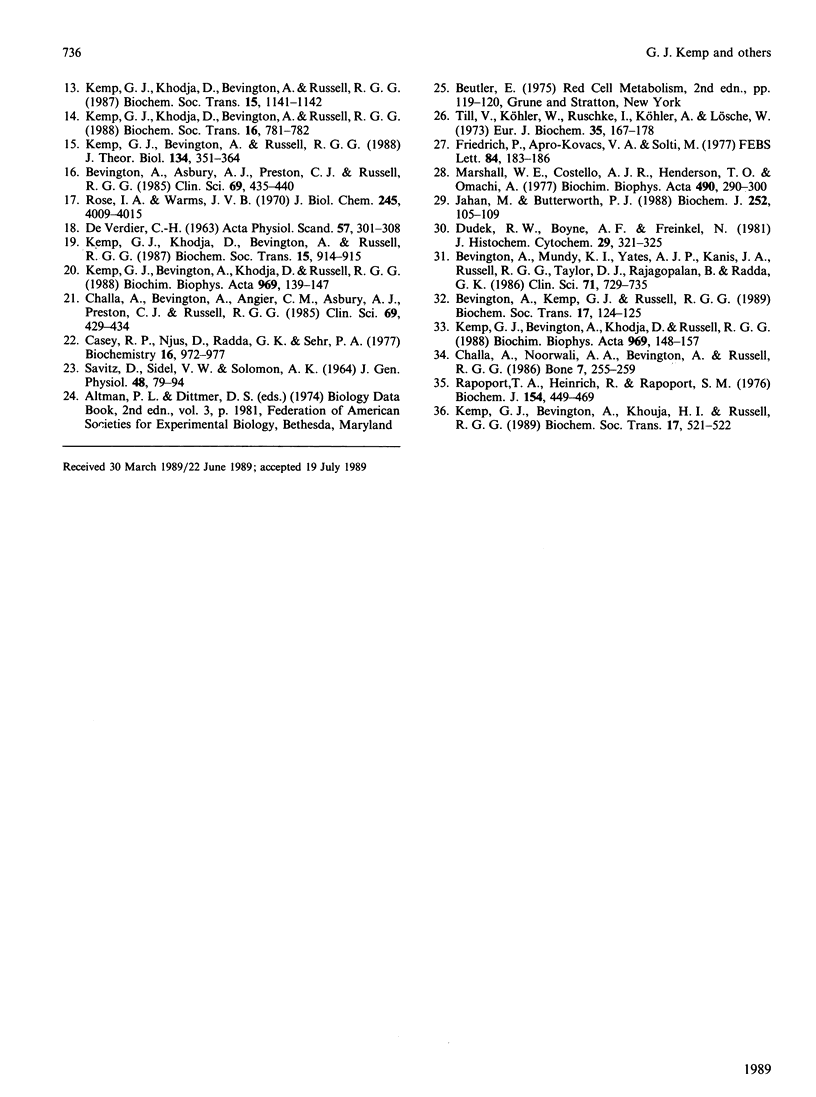
Selected References
These references are in PubMed. This may not be the complete list of references from this article.
- BARTLETT G. R. Organization of red cell glycolytic enzymes; cell coat phosphorus transfer. Ann N Y Acad Sci. 1958 Oct 13;75(1):110–114. doi: 10.1111/j.1749-6632.1958.tb36855.x. [DOI] [PubMed] [Google Scholar]
- Bevington A., Asbury A. J., Preston C. J., Russell R. G. Phosphate metabolism in erythrocytes of critically ill patients. Clin Sci (Lond) 1985 Oct;69(4):435–440. doi: 10.1042/cs0690435. [DOI] [PubMed] [Google Scholar]
- Bevington A., Mundy K. I., Yates A. J., Kanis J. A., Russell R. G., Taylor D. J., Rajagopalan B., Radda G. K. A study of intracellular orthophosphate concentration in human muscle and erythrocytes by 31P nuclear magnetic resonance spectroscopy and selective chemical assay. Clin Sci (Lond) 1986 Dec;71(6):729–735. doi: 10.1042/cs0710729. [DOI] [PubMed] [Google Scholar]
- Brauer M., Spread C. Y., Reithmeier R. A., Sykes B. D. 31P and 35Cl nuclear magnetic resonance measurements of anion transport in human erythrocytes. J Biol Chem. 1985 Sep 25;260(21):11643–11650. [PubMed] [Google Scholar]
- Casey R. P., Njus D., Radda G. K., Sehr P. A. Active proton uptake by chromaffin granules: observation by amine distribution and phosphorus-31 nuclear magnetic resonance techniques. Biochemistry. 1977 Mar 8;16(5):972–977. doi: 10.1021/bi00624a025. [DOI] [PubMed] [Google Scholar]
- Challa A., Bevington A., Angier C. M., Asbury A. J., Preston C. J., Russell R. G. A technique for the measurement of orthophosphate in human erythrocytes, and some studies of its determinants. Clin Sci (Lond) 1985 Oct;69(4):429–434. doi: 10.1042/cs0690429. [DOI] [PubMed] [Google Scholar]
- Challa A., Noorwali A. A., Bevington A., Russell R. G. Cellular phosphate metabolism in patients receiving bisphosphonate therapy. Bone. 1986;7(4):255–259. doi: 10.1016/8756-3282(86)90204-8. [DOI] [PubMed] [Google Scholar]
- Dudek R. W., Boyne A. F., Freinkel N. Quick-freeze fixation and freeze-drying of isolated rat pancreatic islets: application to the ultrastructural localization of inorganic phosphate in the pancreatic beta cell. J Histochem Cytochem. 1981 Feb;29(2):321–325. doi: 10.1177/29.2.7019306. [DOI] [PubMed] [Google Scholar]
- Friedrich P., Apró-Kovács V. A., Solti M. Study of metabolite compartmentation in erythrocyte glycolysis. FEBS Lett. 1977 Dec 1;84(1):183–186. doi: 10.1016/0014-5793(77)81085-5. [DOI] [PubMed] [Google Scholar]
- Ho M. K., Guidotti G. A membrane protein from human erythrocytes involved in anion exchange. J Biol Chem. 1975 Jan 25;250(2):675–683. [PubMed] [Google Scholar]
- Jahan M., Butterworth P. J. Study of the mechanism by which the Na+-Pi co-transporter of mouse kidney proximal-tubule cells adjusts to phosphate depletion. Biochem J. 1988 May 15;252(1):105–109. doi: 10.1042/bj2520105. [DOI] [PMC free article] [PubMed] [Google Scholar]
- Kemp G. J., Bevington A., Khodja D., Russell R. G. Net fluxes of orthophosphate across the plasma membrane in human red cells following alteration of pH and extracellular Pi concentration. Biochim Biophys Acta. 1988 Apr 25;969(2):148–157. doi: 10.1016/0167-4889(88)90070-5. [DOI] [PubMed] [Google Scholar]
- Kemp G. J., Bevington A., Khodja D., Russell R. G. Regulation of phosphate metabolism in human red cells following prolonged incubation to steady state in vitro. Biochim Biophys Acta. 1988 Apr 25;969(2):139–147. doi: 10.1016/0167-4889(88)90069-9. [DOI] [PubMed] [Google Scholar]
- Kemp G. J., Bevington A., Russell R. G. Theoretical interpretation of isotope labelling experiments in cells in which the label is chemically incorporated: the example of orthophosphate. J Theor Biol. 1988 Oct 7;134(3):351–364. doi: 10.1016/s0022-5193(88)80066-3. [DOI] [PubMed] [Google Scholar]
- King C. E., Stephens L. R., Hawkins P. T., Guy G. R., Michell R. H. Multiple metabolic pools of phosphoinositides and phosphatidate in human erythrocytes incubated in a medium that permits rapid transmembrane exchange of phosphate. Biochem J. 1987 May 15;244(1):209–217. doi: 10.1042/bj2440209. [DOI] [PMC free article] [PubMed] [Google Scholar]
- Latzkovits L., Huszák I., Széchenyi F. In vitro examinations of phosphate exchange between plasma and erythrocytes in the blood of multiple sclerotic patients. Brain. 1966 Dec;89(4):831–836. doi: 10.1093/brain/89.4.831. [DOI] [PubMed] [Google Scholar]
- Marshall W. E., Costello A. J., Henderson T. O., Omachi A. Organic phosphate binding to hemoglobin in intact human erythrocytes determined by 31P nuclear magnetic resonance spectroscopy. Biochim Biophys Acta. 1977 Feb 22;490(2):290–300. doi: 10.1016/0005-2795(77)90004-6. [DOI] [PubMed] [Google Scholar]
- Medina G., Illingworth J. A. Some hormonal effects on myocardial phosphate efflux. Biochem J. 1984 Nov 15;224(1):153–162. doi: 10.1042/bj2240153. [DOI] [PMC free article] [PubMed] [Google Scholar]
- Niehaus W. G., Jr, Hammerstedt R. H. Mode of orthophosphate uptake and ATP labeling by mammalian cells. Biochim Biophys Acta. 1976 Sep 7;443(3):515–524. doi: 10.1016/0005-2736(76)90469-7. [DOI] [PubMed] [Google Scholar]
- PRANKERD T. A., ALTMAN K. I. A study of the metabolism of phosphorus in mammalian red cells. Biochem J. 1954 Dec;58(4):622–633. doi: 10.1042/bj0580622. [DOI] [PMC free article] [PubMed] [Google Scholar]
- Rapoport T. A., Heinrich R., Rapoport S. M. The regulatory principles of glycolysis in erythrocytes in vivo and in vitro. A minimal comprehensive model describing steady states, quasi-steady states and time-dependent processes. Biochem J. 1976 Feb 15;154(2):449–469. doi: 10.1042/bj1540449. [DOI] [PMC free article] [PubMed] [Google Scholar]
- Rose I. A., Warms J. V. Control of red cell glycolysis. The cause of triose phosphate accumulation. J Biol Chem. 1970 Aug 25;245(16):4009–4015. [PubMed] [Google Scholar]
- SAVITZ D., SIDEL V. W., SOLOMON A. K. OSMOTIC PROPERTIES OF HUMAN RED CELLS. J Gen Physiol. 1964 Sep;48:79–94. doi: 10.1085/jgp.48.1.79. [DOI] [PMC free article] [PubMed] [Google Scholar]
- Shoemaker D. G., Bender C. A., Gunn R. B. Sodium-phosphate cotransport in human red blood cells. Kinetics and role in membrane metabolism. J Gen Physiol. 1988 Oct;92(4):449–474. doi: 10.1085/jgp.92.4.449. [DOI] [PMC free article] [PubMed] [Google Scholar]
- Till U., Köhler W., Ruschke I., Köhler A., Lösche W. Compartmentation of orthophosphate and adenine nucleotides in human red cells. Eur J Biochem. 1973 May;35(1):167–178. doi: 10.1111/j.1432-1033.1973.tb02822.x. [DOI] [PubMed] [Google Scholar]
- de VERDIER C. Exchange of phosphate-groups between inorganic phosphate and adenosine triphosphate in red blood cells. Acta Physiol Scand. 1963 Apr;57:301–308. doi: 10.1111/j.1748-1716.1963.tb02593.x. [DOI] [PubMed] [Google Scholar]