Abstract
The activity of a hormone- and growth-factor-stimulated NADH oxidase of the rat liver plasma membrane responds to guanine nucleotides, but in a manner that differs from that of the classic trimeric and low-molecular-mass monomeric G-proteins. In the absence of added bivalent ions, both GTP and GDP as well as guanosine 5'-[gamma-thio]triphosphate (GTP[gamma-S]) but not guanosine 5'[beta-thio]diphosphate (GDP[beta-S]) stimulate the activity over the range 1 microM to 100 microM. Other di- and tri-nucleotides also stimulate, but only at concentrations of 100 microM or higher. Added bivalent ions are not required either for NADH oxidation or guanine nucleotide stimulation. Bivalent ions (Mg2+ > Mn2+ > or = Ca2+) alone stimulate only slightly at low concentrations and then inhibit at high concentrations. The inhibitions are augmented by GDP or GTP [gamma-S] but not by GTP. Although the activity is the same, or less, in the presence of 0.5 mM MgCl2, GTP at 1-100 nM and other nucleotides at 0.1 mM or 1 mM still stimulate in its presence. The NADH oxidase is activated by mastoparan but aluminum fluoride is weakly inhibitory. Cholera and pertussis toxins elicit only marginal responses. Both the Mg2+ and the GDP and GTP[gamma-S] inhibitions (but not the GTP stimulations) shift to higher concentrations when the membrane preparations are first solubilized with Triton X-100. The results suggest a role for guanine nucleotides in the regulation of plasma membrane NADH oxidase, but with properties that differ from those of either trimeric or the low-molecular-mass G proteins thus far described.
Full text
PDF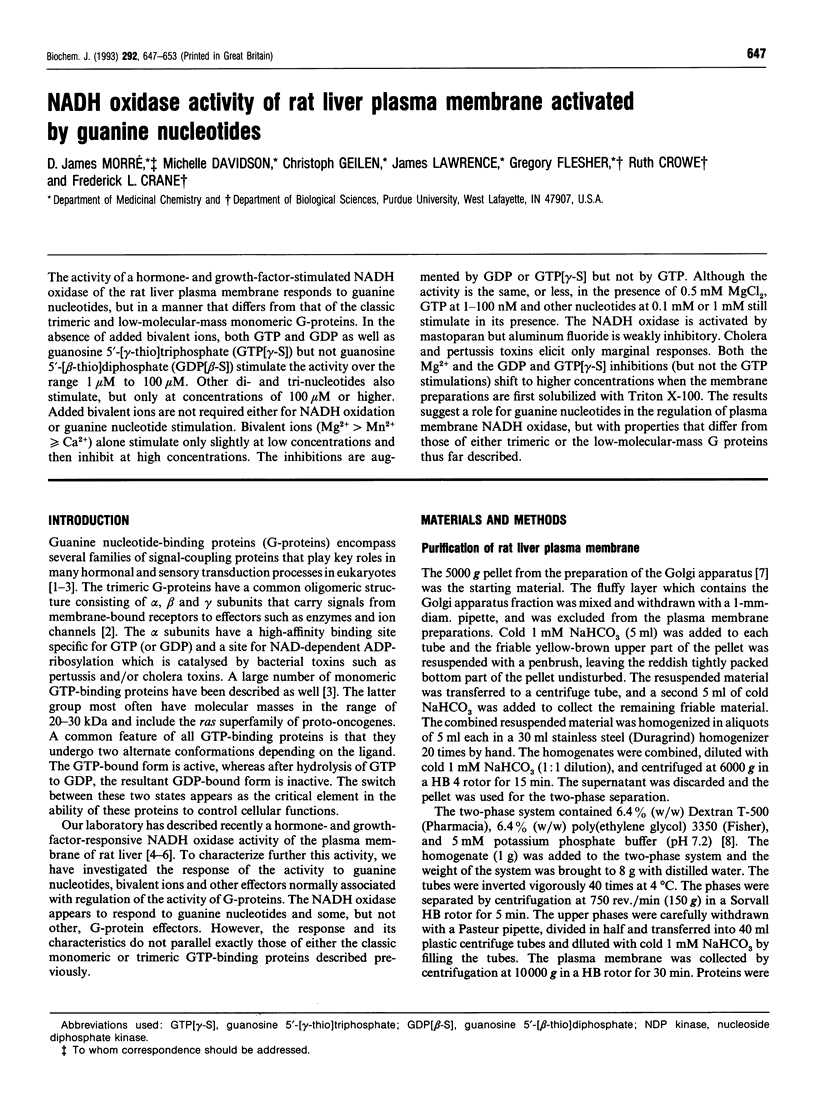
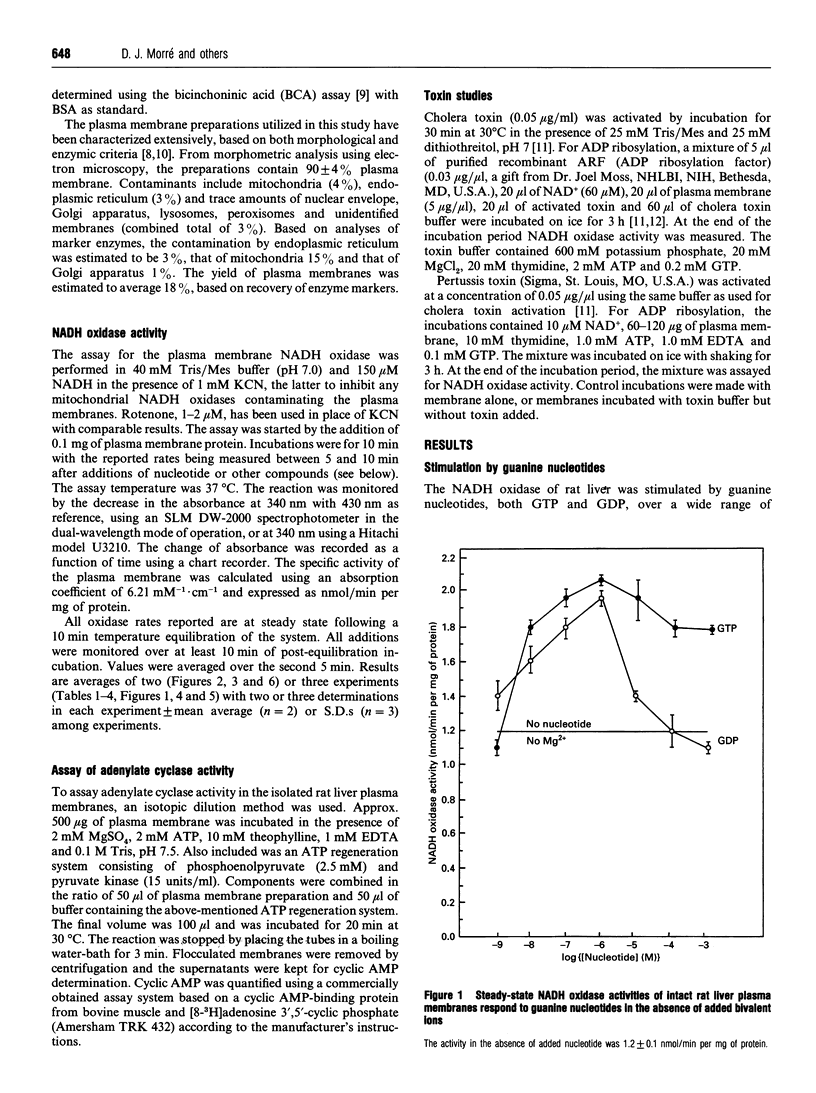
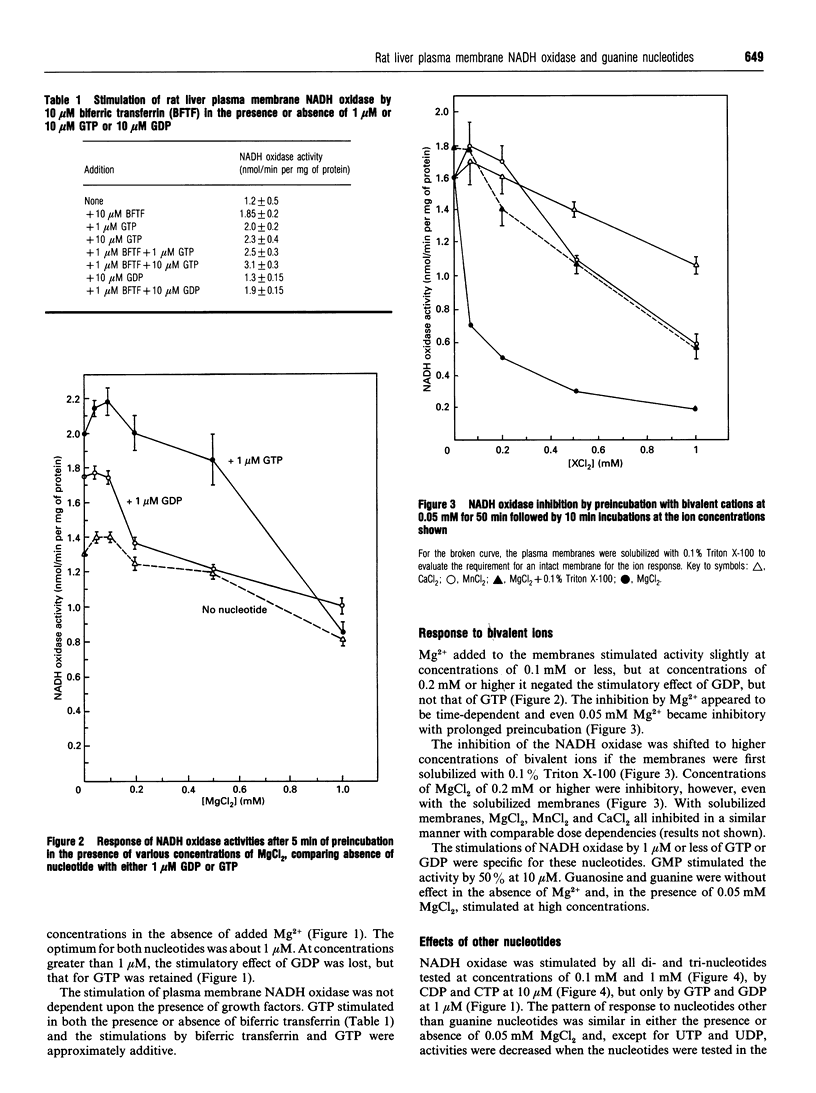
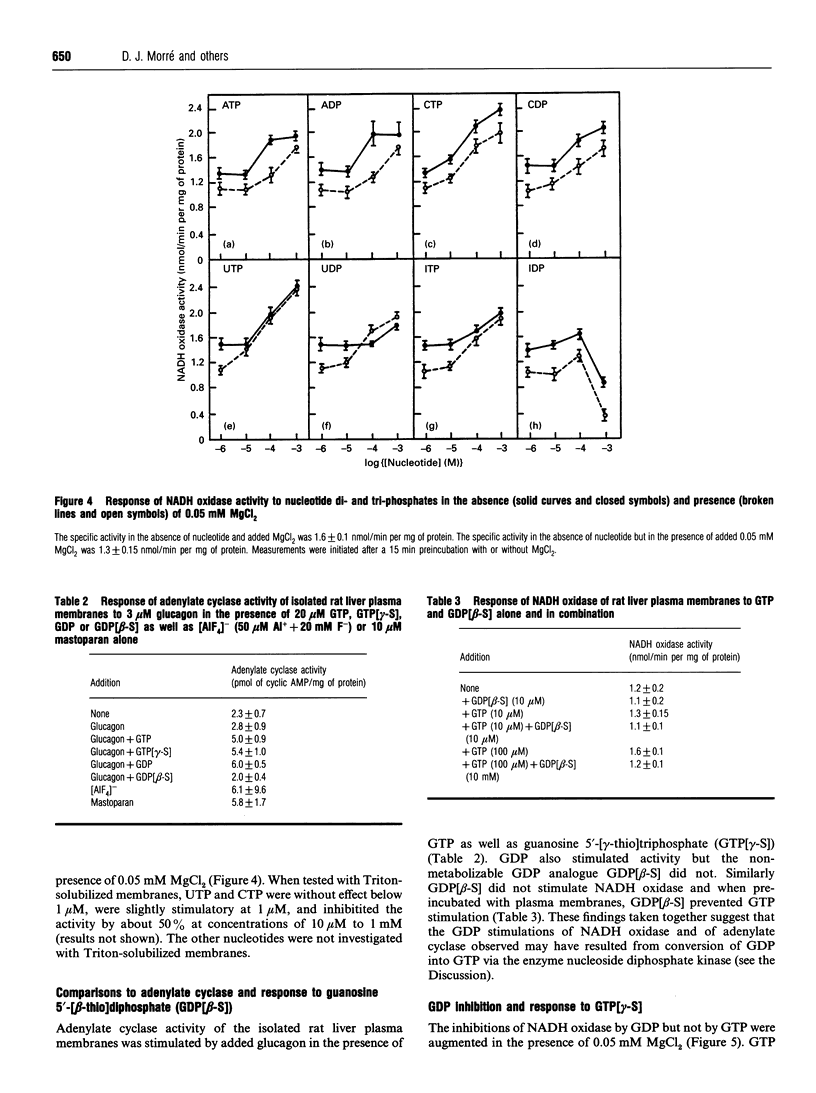
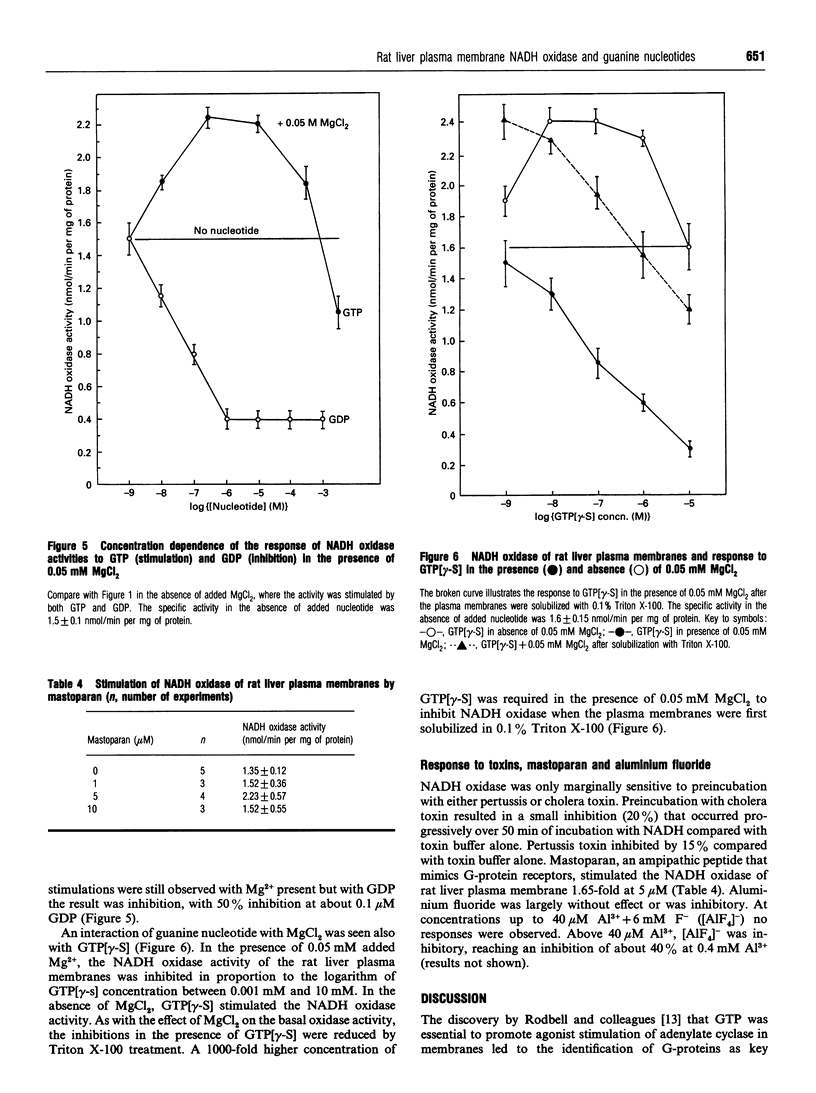
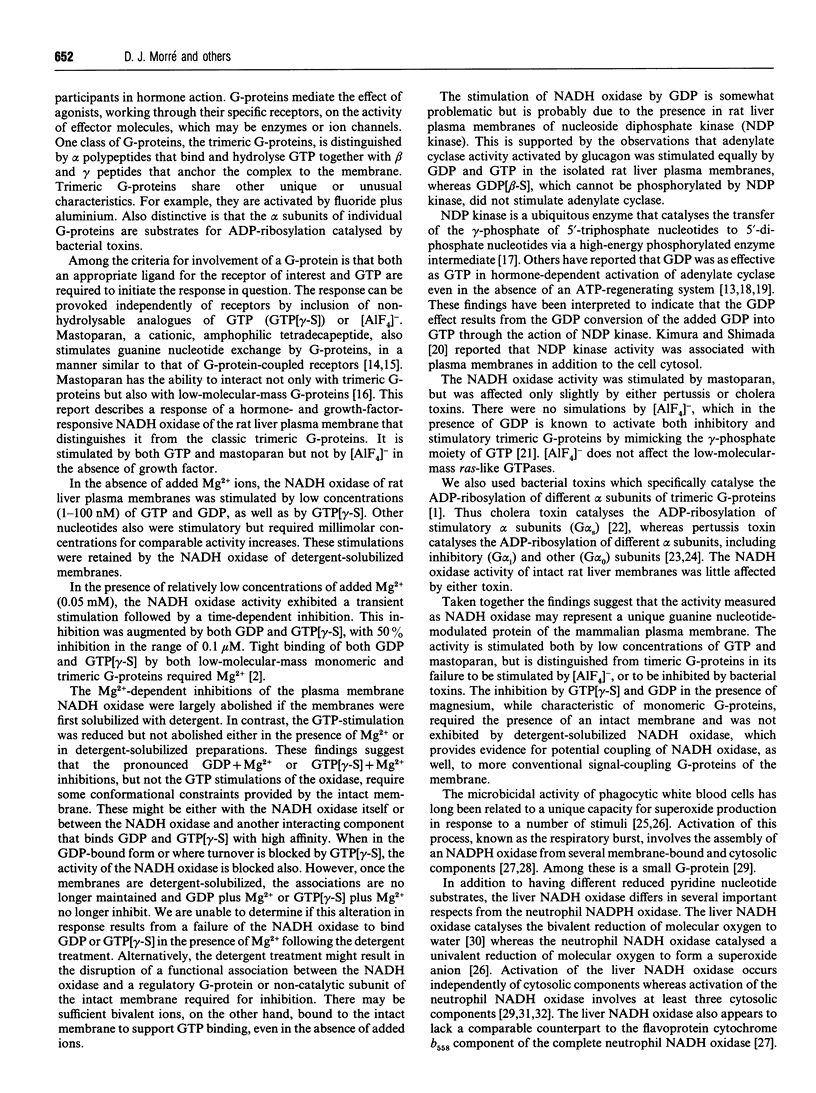
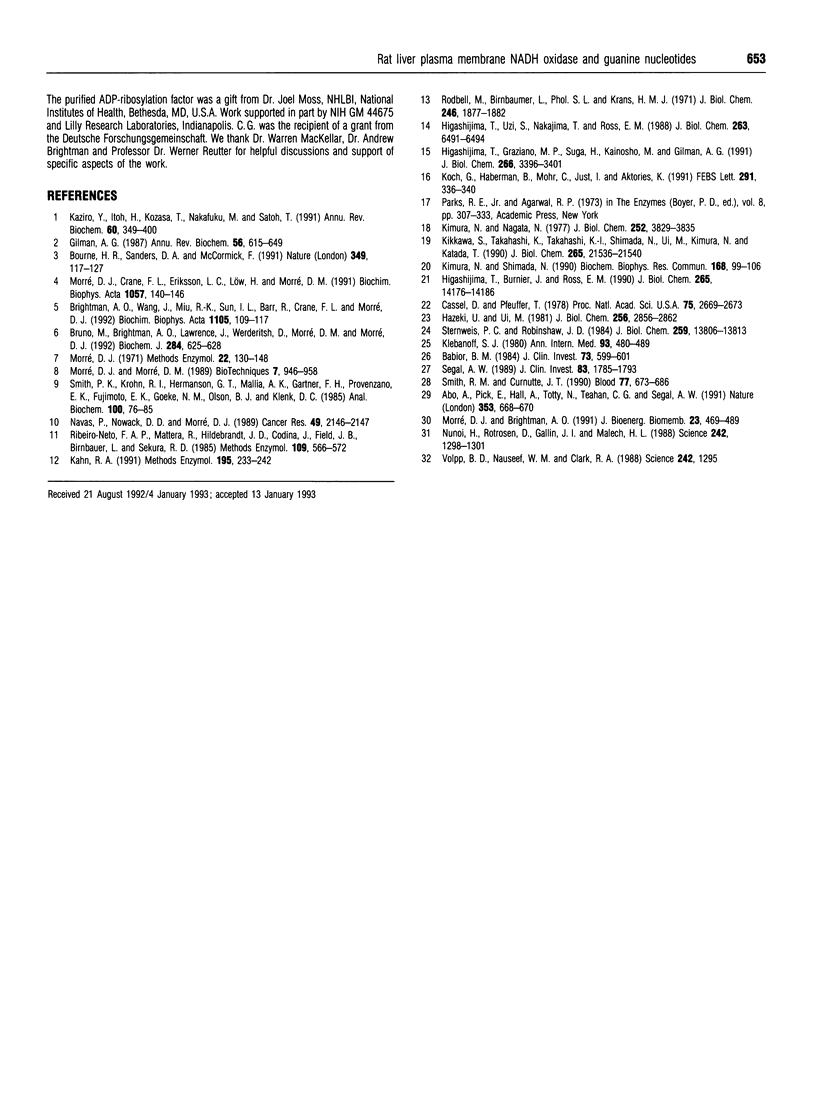
Selected References
These references are in PubMed. This may not be the complete list of references from this article.
- Abo A., Pick E., Hall A., Totty N., Teahan C. G., Segal A. W. Activation of the NADPH oxidase involves the small GTP-binding protein p21rac1. Nature. 1991 Oct 17;353(6345):668–670. doi: 10.1038/353668a0. [DOI] [PubMed] [Google Scholar]
- Babior B. M. The respiratory burst of phagocytes. J Clin Invest. 1984 Mar;73(3):599–601. doi: 10.1172/JCI111249. [DOI] [PMC free article] [PubMed] [Google Scholar]
- Bourne H. R., Sanders D. A., McCormick F. The GTPase superfamily: conserved structure and molecular mechanism. Nature. 1991 Jan 10;349(6305):117–127. doi: 10.1038/349117a0. [DOI] [PubMed] [Google Scholar]
- Brightman A. O., Wang J., Miu R. K., Sun I. L., Barr R., Crane F. L., Morré D. J. A growth factor- and hormone-stimulated NADH oxidase from rat liver plasma membrane. Biochim Biophys Acta. 1992 Mar 23;1105(1):109–117. doi: 10.1016/0005-2736(92)90168-l. [DOI] [PubMed] [Google Scholar]
- Bruno M., Brightman A. O., Lawrence J., Werderitsh D., Morré D. M., Morre D. J. Stimulation of NADH oxidase activity from rat liver plasma membranes by growth factors and hormones is decreased or absent with hepatoma plasma membranes. Biochem J. 1992 Jun 15;284(Pt 3):625–628. doi: 10.1042/bj2840625. [DOI] [PMC free article] [PubMed] [Google Scholar]
- Cassel D., Pfeuffer T. Mechanism of cholera toxin action: covalent modification of the guanyl nucleotide-binding protein of the adenylate cyclase system. Proc Natl Acad Sci U S A. 1978 Jun;75(6):2669–2673. doi: 10.1073/pnas.75.6.2669. [DOI] [PMC free article] [PubMed] [Google Scholar]
- Gilman A. G. G proteins: transducers of receptor-generated signals. Annu Rev Biochem. 1987;56:615–649. doi: 10.1146/annurev.bi.56.070187.003151. [DOI] [PubMed] [Google Scholar]
- Hazeki O., Ui M. Modification by islet-activating protein of receptor-mediated regulation of cyclic AMP accumulation in isolated rat heart cells. J Biol Chem. 1981 Mar 25;256(6):2856–2862. [PubMed] [Google Scholar]
- Higashijima T., Burnier J., Ross E. M. Regulation of Gi and Go by mastoparan, related amphiphilic peptides, and hydrophobic amines. Mechanism and structural determinants of activity. J Biol Chem. 1990 Aug 25;265(24):14176–14186. [PubMed] [Google Scholar]
- Higashijima T., Graziano M. P., Suga H., Kainosho M., Gilman A. G. 19F and 31P NMR spectroscopy of G protein alpha subunits. Mechanism of activation by Al3+ and F-. J Biol Chem. 1991 Feb 25;266(6):3396–3401. [PubMed] [Google Scholar]
- Higashijima T., Uzu S., Nakajima T., Ross E. M. Mastoparan, a peptide toxin from wasp venom, mimics receptors by activating GTP-binding regulatory proteins (G proteins). J Biol Chem. 1988 May 15;263(14):6491–6494. [PubMed] [Google Scholar]
- Kahn R. A. Quantitation and purification of ADP-ribosylation factor. Methods Enzymol. 1991;195:233–242. doi: 10.1016/0076-6879(91)95169-k. [DOI] [PubMed] [Google Scholar]
- Kaziro Y., Itoh H., Kozasa T., Nakafuku M., Satoh T. Structure and function of signal-transducing GTP-binding proteins. Annu Rev Biochem. 1991;60:349–400. doi: 10.1146/annurev.bi.60.070191.002025. [DOI] [PubMed] [Google Scholar]
- Kikkawa S., Takahashi K., Takahashi K., Shimada N., Ui M., Kimura N., Katada T. Conversion of GDP into GTP by nucleoside diphosphate kinase on the GTP-binding proteins. J Biol Chem. 1990 Dec 15;265(35):21536–21540. [PubMed] [Google Scholar]
- Kimura N., Nagata N. The requirement of guanine nucleotides for glucagon stimulation of adenylate cyclase in rat liver plasma membranes. J Biol Chem. 1977 Jun 10;252(11):3829–3835. [PubMed] [Google Scholar]
- Kimura N., Shimada N. Evidence for complex formation between GTP binding protein(Gs) and membrane-associated nucleoside diphosphate kinase. Biochem Biophys Res Commun. 1990 Apr 16;168(1):99–106. doi: 10.1016/0006-291x(90)91680-q. [DOI] [PubMed] [Google Scholar]
- Klebanoff S. J. Oxygen metabolism and the toxic properties of phagocytes. Ann Intern Med. 1980 Sep;93(3):480–489. doi: 10.7326/0003-4819-93-3-480. [DOI] [PubMed] [Google Scholar]
- Koch G., Haberman B., Mohr C., Just I., Aktories K. Interaction of mastoparan with the low molecular mass GTP-binding proteins rho/rac. FEBS Lett. 1991 Oct 21;291(2):336–340. doi: 10.1016/0014-5793(91)81315-y. [DOI] [PubMed] [Google Scholar]
- Morré D. J., Brightman A. O. NADH oxidase of plasma membranes. J Bioenerg Biomembr. 1991 Jun;23(3):469–489. doi: 10.1007/BF00771015. [DOI] [PubMed] [Google Scholar]
- Morré D. J., Crane F. L., Eriksson L. C., Löw H., Morré D. M. NADH oxidase of liver plasma membrane stimulated by diferric transferrin and neoplastic transformation induced by the carcinogen 2-acetylaminofluorene. Biochim Biophys Acta. 1991 Mar 1;1057(1):140–146. doi: 10.1016/s0005-2728(05)80094-5. [DOI] [PubMed] [Google Scholar]
- Morré D. J., Morré D. M. Preparation of mammalian plasma membranes by aqueous two-phase partition. Biotechniques. 1989 Oct;7(9):946-8, 950-4, 956-8. [PubMed] [Google Scholar]
- Navas P., Nowack D. D., Morré D. J. Isolation of purified plasma membranes from cultured cells and hepatomas by two-phase partition and preparative free-flow electrophoresis. Cancer Res. 1989 Apr 15;49(8):2147–2156. [PubMed] [Google Scholar]
- Ribeiro-Neto F. A., Mattera R., Hildebrandt J. D., Codina J., Field J. B., Birnbaumer L., Sekura R. D. ADP-ribosylation of membrane components by pertussis and cholera toxin. Methods Enzymol. 1985;109:566–572. doi: 10.1016/0076-6879(85)09115-7. [DOI] [PubMed] [Google Scholar]
- Rodbell M., Birnbaumer L., Pohl S. L., Krans H. M. The glucagon-sensitive adenyl cyclase system in plasma membranes of rat liver. V. An obligatory role of guanylnucleotides in glucagon action. J Biol Chem. 1971 Mar 25;246(6):1877–1882. [PubMed] [Google Scholar]
- Segal A. W. The electron transport chain of the microbicidal oxidase of phagocytic cells and its involvement in the molecular pathology of chronic granulomatous disease. J Clin Invest. 1989 Jun;83(6):1785–1793. doi: 10.1172/JCI114083. [DOI] [PMC free article] [PubMed] [Google Scholar]
- Smith P. K., Krohn R. I., Hermanson G. T., Mallia A. K., Gartner F. H., Provenzano M. D., Fujimoto E. K., Goeke N. M., Olson B. J., Klenk D. C. Measurement of protein using bicinchoninic acid. Anal Biochem. 1985 Oct;150(1):76–85. doi: 10.1016/0003-2697(85)90442-7. [DOI] [PubMed] [Google Scholar]
- Smith R. M., Curnutte J. T. Molecular basis of chronic granulomatous disease. Blood. 1991 Feb 15;77(4):673–686. [PubMed] [Google Scholar]
- Sternweis P. C., Robishaw J. D. Isolation of two proteins with high affinity for guanine nucleotides from membranes of bovine brain. J Biol Chem. 1984 Nov 25;259(22):13806–13813. [PubMed] [Google Scholar]
- Volpp B. D., Nauseef W. M., Clark R. A. Two cytosolic neutrophil oxidase components absent in autosomal chronic granulomatous disease. Science. 1988 Dec 2;242(4883):1295–1297. doi: 10.1126/science.2848318. [DOI] [PubMed] [Google Scholar]