Abstract
The mobilization of Ca2+ from intracellular stores by Ins(1,4,5)P3 in suspensions of permeabilized rat hepatocytes was potentiated by preincubating intact cells with adenosine 3':5'-cyclic phosphorothioate (cpt-cAMP), or by addition of the catalytic subunit of cyclic-AMP-dependent protein kinase (PKA) after cell permeabilization. This action of PKA involved both an enhancement in Ins(1,4,5)P3 sensitivity and an increase in the size of the Ins(1,4,5)P3-releasable Ca2+ pool. Inclusion of the protein phosphatase inhibitor okadaic acid in the permeabilization medium augmented the effects of PKA. Treatment with PKA catalytic subunit also increased the rate of ATP-dependent Ca2+ sequestration. To determine whether the effects of PKA on the Ca(2+)-release mechanism were secondary to alterations in the Ca2+ load of the Ins(1,4,5)P3-sensitive stores, a method was developed using Mn2+ as a Ca2+ surrogate to examine the permeability properties of the Ins(1,4,5)P3-gated channels independent of Ca2+ fluxes. This approach utilized the ability of Mn2+ to quench the fluorescence of fura-2 compartmentalized within intracellular Ca2+ stores in an Ins(1,4,5)P3-dependent manner, with thapsigargin added to block the ATP-activated Ca2+ pump and to ensure that the Ca2+ stores were fully depleted of Ca2+. The initial rate and extent of Mn2+ quenching of compartmentalized fura-2 was increased in a dose-dependent manner by Ins(1,4,5)P3. PKA activation increased both the initial rate and the extent of Mn2+ quenching at sub-maximal Ins(1,4,5)P3 doses, but there was no effect on the quench rate in the presence of saturating Ins(1,4,5)P3. However, the amount of compartmentalized fura-2 that could be quenched by Mn2+ in the presence of maximal Ins(1,4,5)P3 was increased by PKA. These data suggest two distinct actions of PKA on the Ins(1,4,5)P3-sensitive Ca2+ stores. (1) Modification of the ion-permeability properties of the Ins(1,4,5)P3 receptor/channel through an increase in the sensitivity to Ins(1,4,5)P3 for channel opening. (2) A recruitment of Ca2+ stores from the Ins(1,4,5)P3-insensitive pool. Both actions were independent of the Ca(2+)-loading state of the stores. Imaging studies of single permeabilized hepatocytes showed that the Ins(1,4,5)P3-sensitive stores were distributed throughout the cell and PKA enhanced the rate of Ins(1,4,5)P3-stimulated Mn2+ quench in individual cells, without modifying the subcellular distribution of Ins(1,4,5)P3-sensitive stores.
Full text
PDF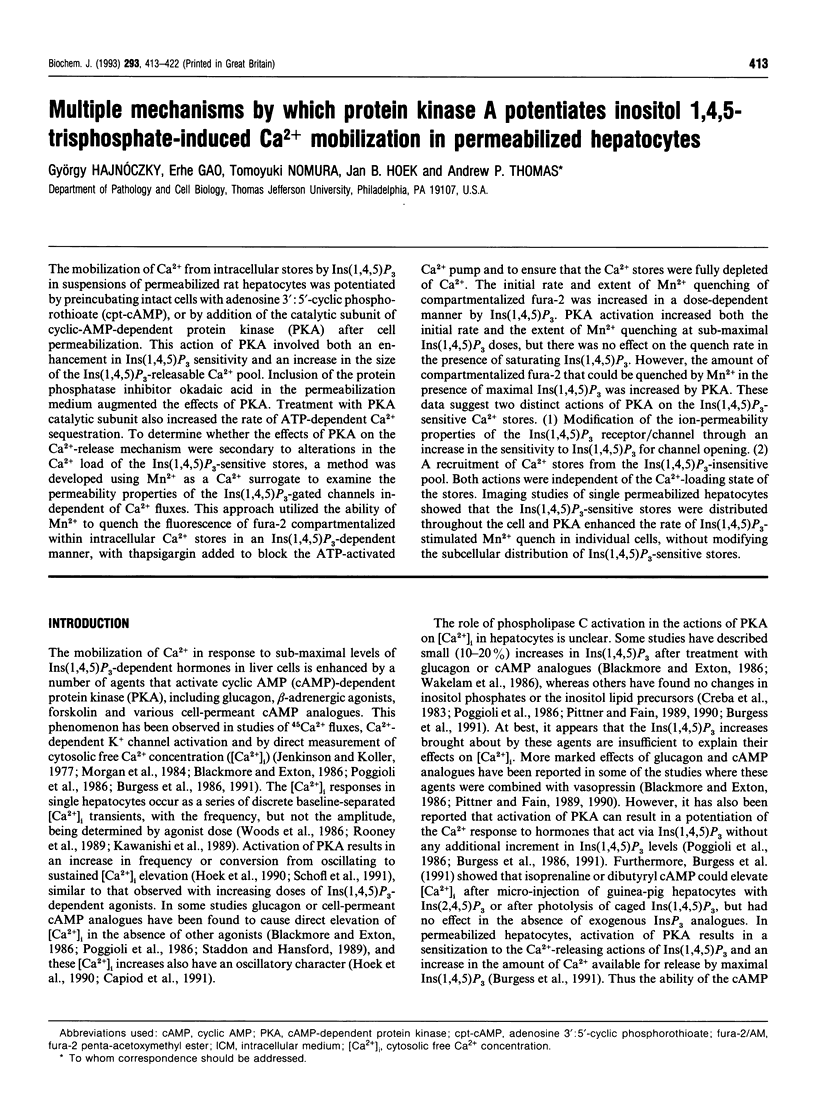
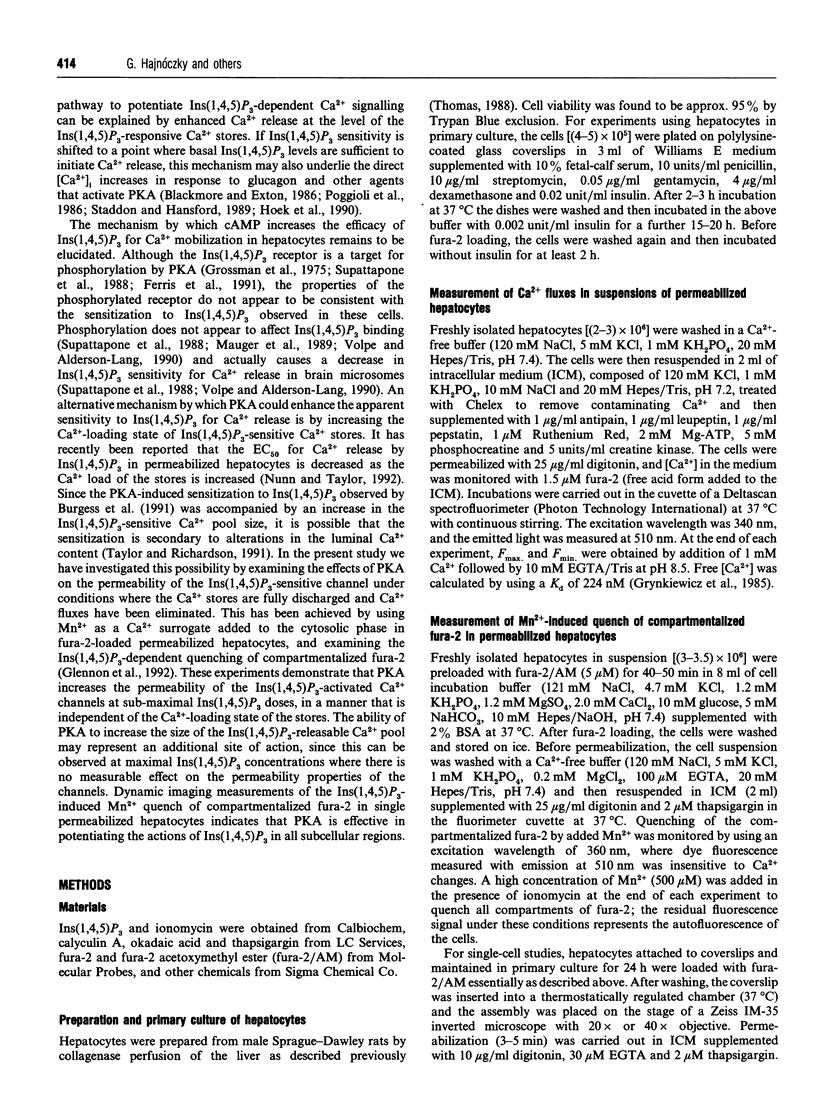
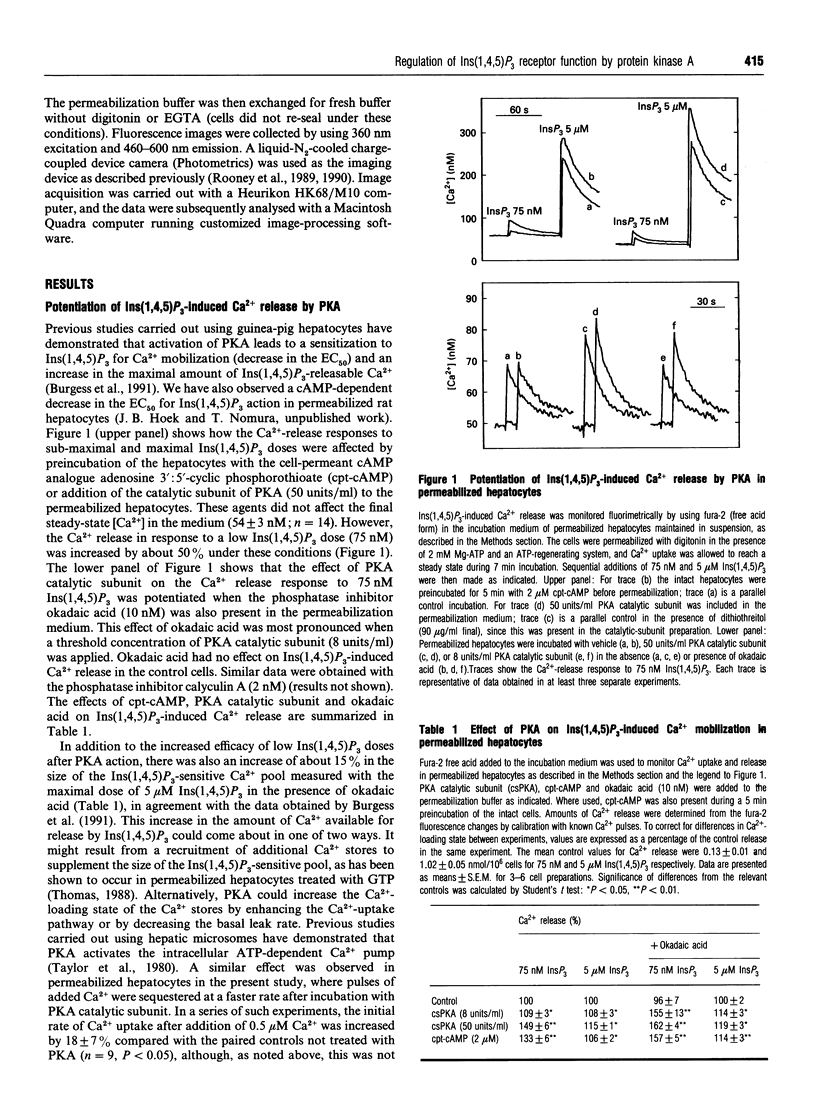
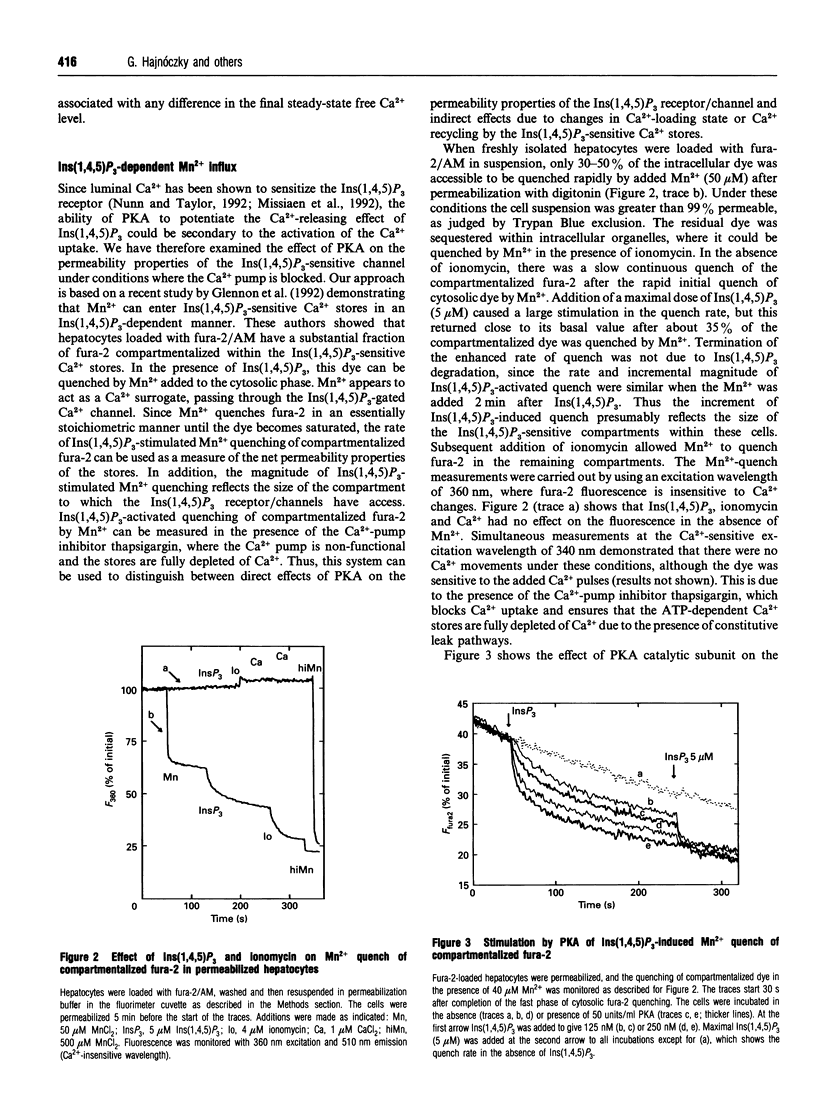
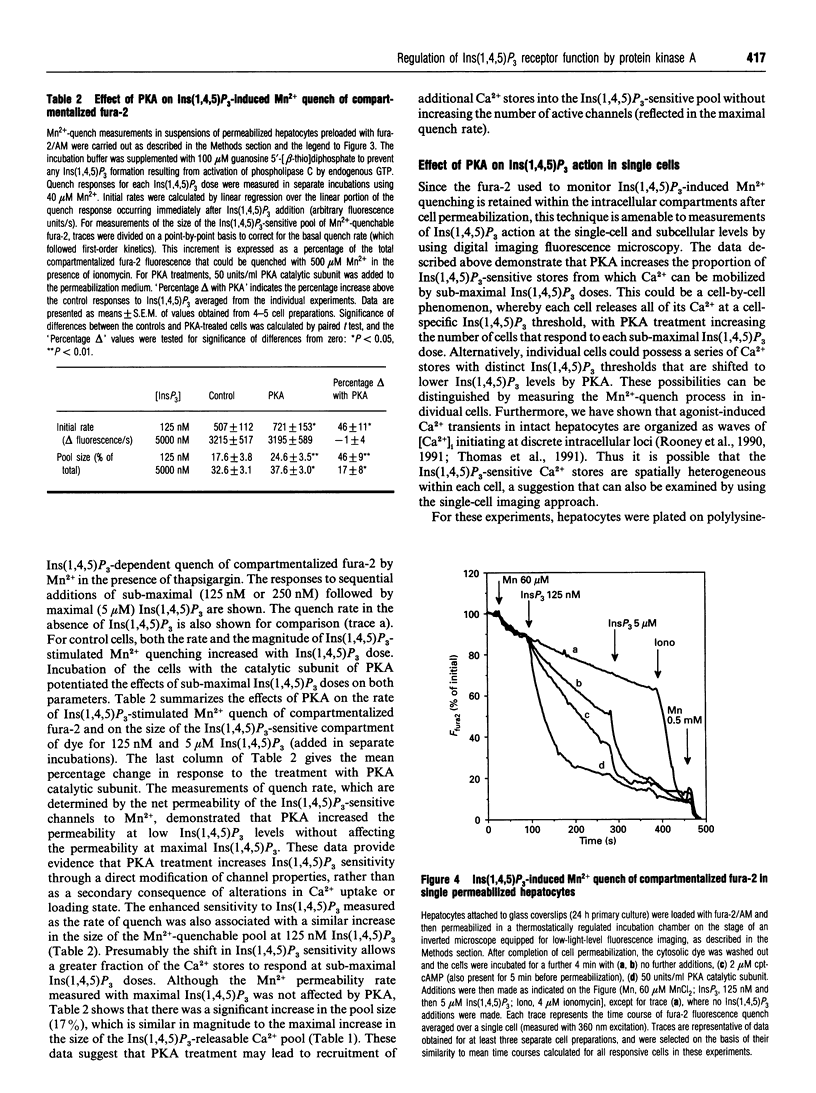
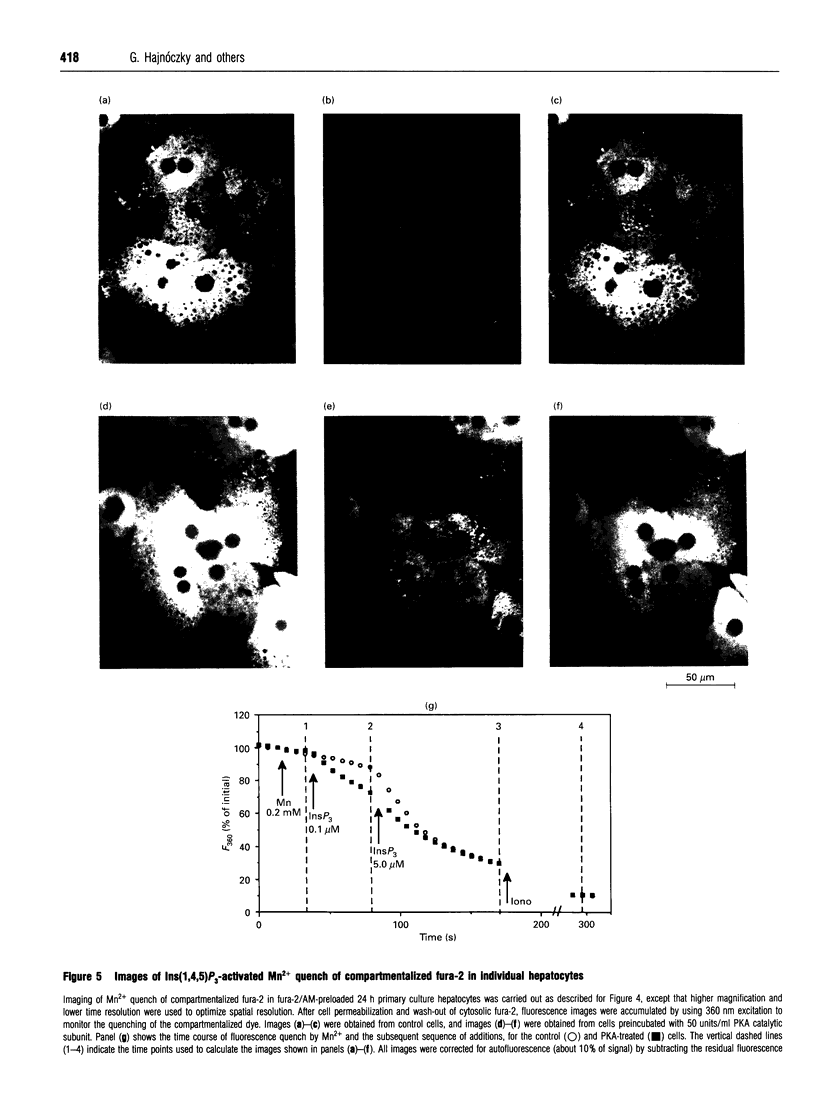
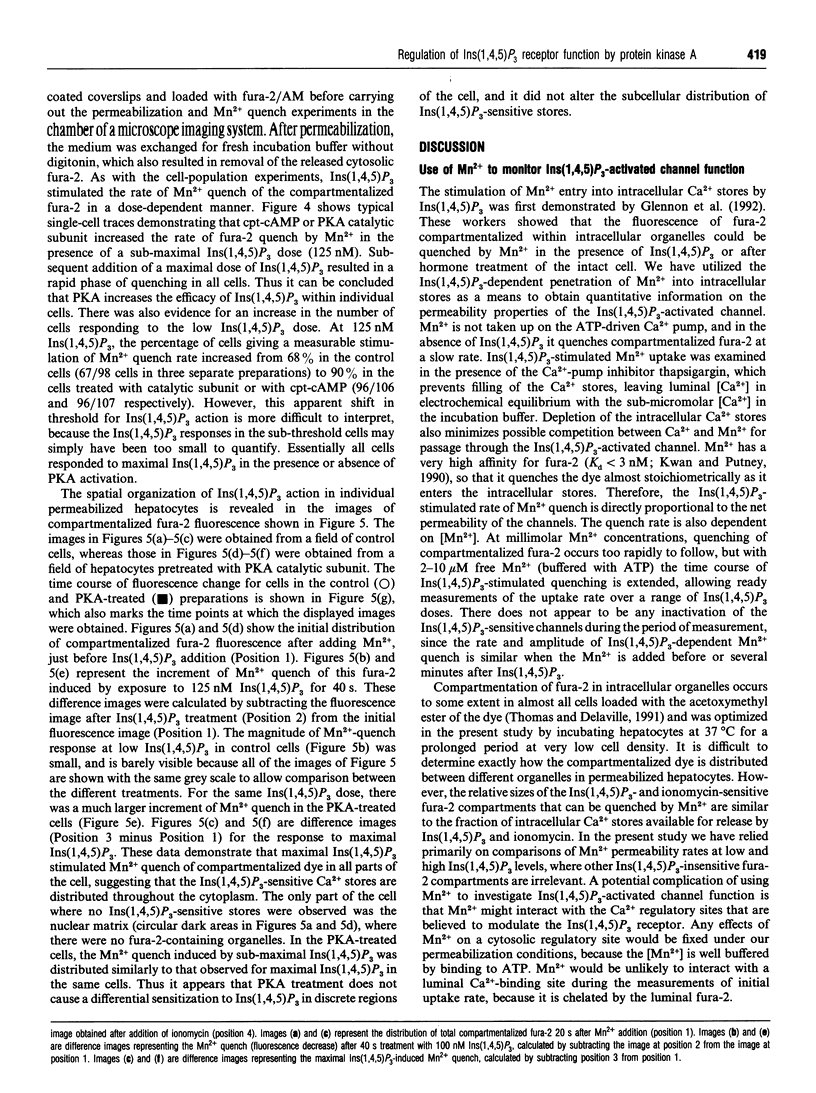
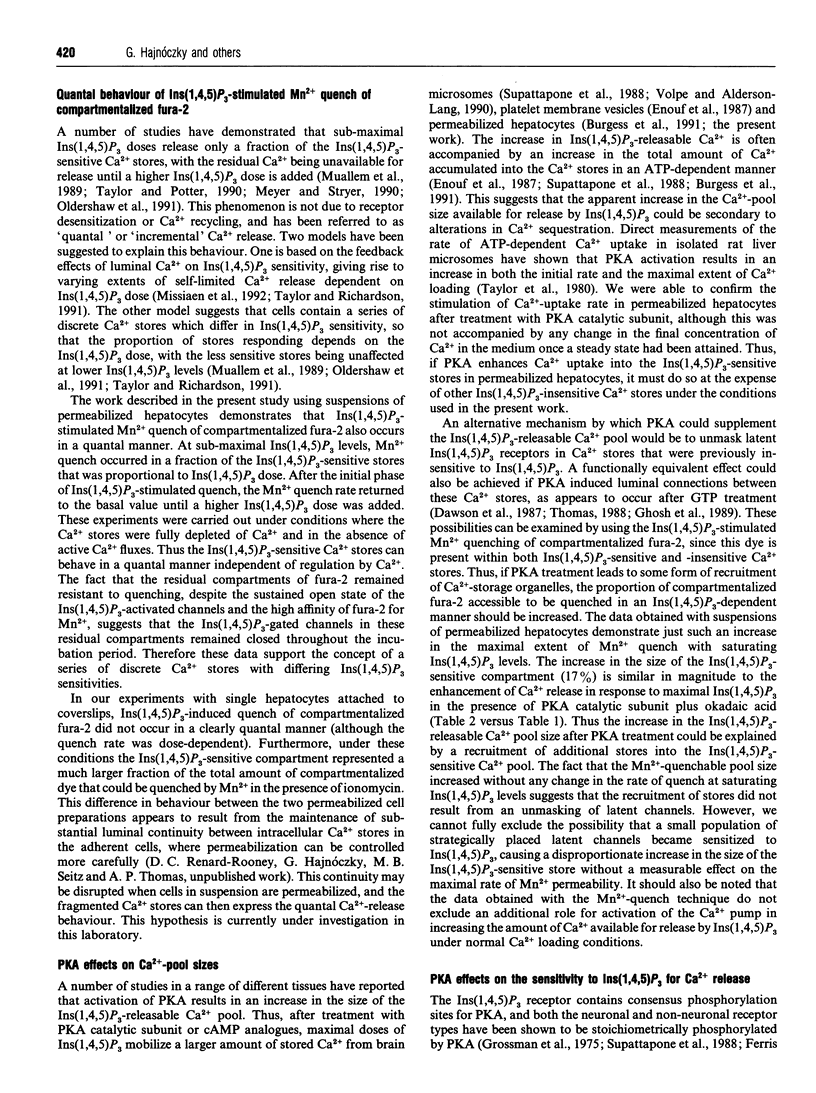
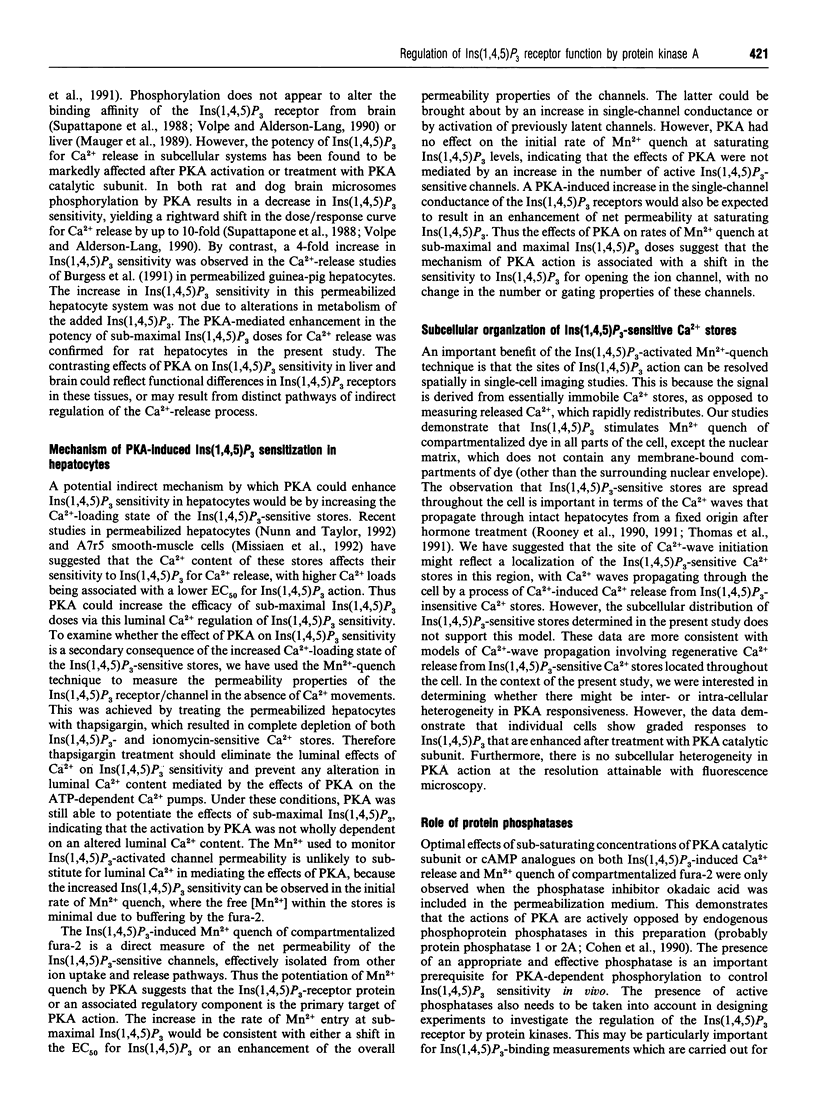
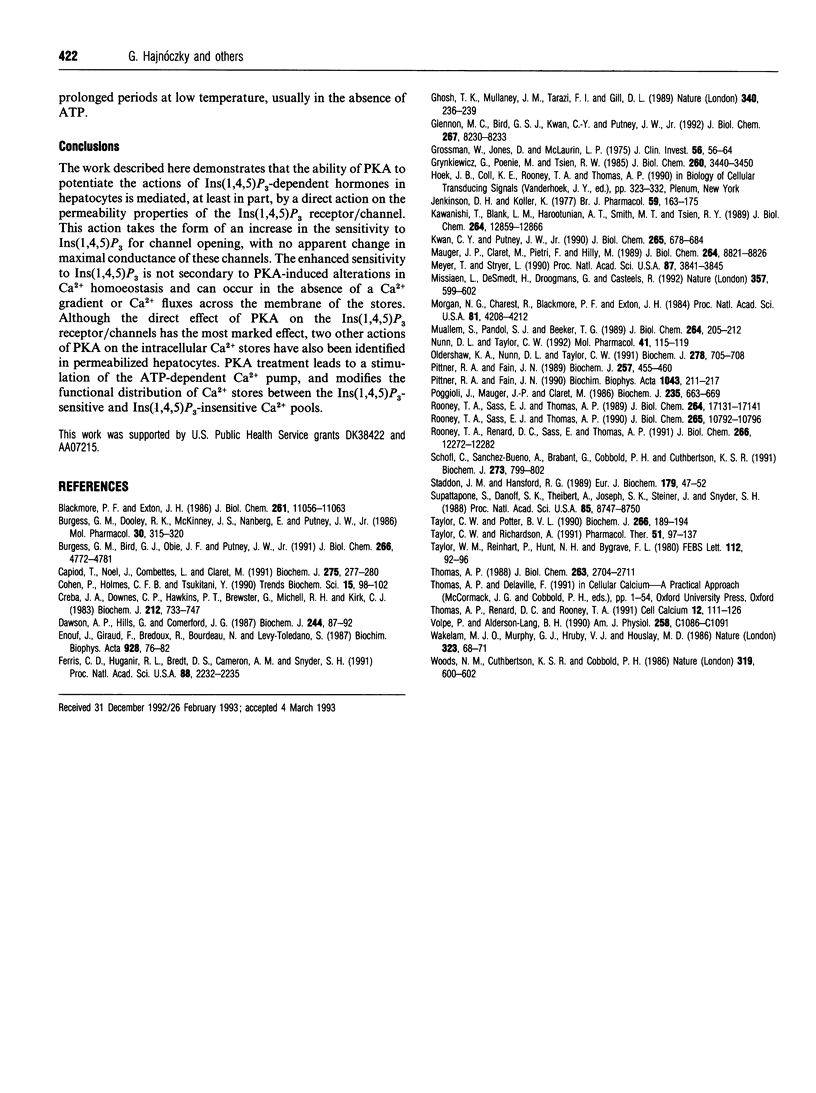
Images in this article
Selected References
These references are in PubMed. This may not be the complete list of references from this article.
- Blackmore P. F., Exton J. H. Studies on the hepatic calcium-mobilizing activity of aluminum fluoride and glucagon. Modulation by cAMP and phorbol myristate acetate. J Biol Chem. 1986 Aug 25;261(24):11056–11063. [PubMed] [Google Scholar]
- Burgess G. M., Bird G. S., Obie J. F., Putney J. W., Jr The mechanism for synergism between phospholipase C- and adenylylcyclase-linked hormones in liver. Cyclic AMP-dependent kinase augments inositol trisphosphate-mediated Ca2+ mobilization without increasing the cellular levels of inositol polyphosphates. J Biol Chem. 1991 Mar 15;266(8):4772–4781. [PubMed] [Google Scholar]
- Burgess G. M., Dooley R. K., McKinney J. S., Nånberg E., Putney J. W., Jr Further studies on the interactions between the calcium mobilization and cyclic AMP pathways in guinea pig hepatocytes. Mol Pharmacol. 1986 Oct;30(4):315–320. [PubMed] [Google Scholar]
- Capiod T., Noel J., Combettes L., Claret M. Cyclic AMP-evoked oscillations of intracellular [Ca2+] in guinea-pig hepatocytes. Biochem J. 1991 Apr 1;275(Pt 1):277–280. doi: 10.1042/bj2750277. [DOI] [PMC free article] [PubMed] [Google Scholar]
- Cohen P., Holmes C. F., Tsukitani Y. Okadaic acid: a new probe for the study of cellular regulation. Trends Biochem Sci. 1990 Mar;15(3):98–102. doi: 10.1016/0968-0004(90)90192-e. [DOI] [PubMed] [Google Scholar]
- Creba J. A., Downes C. P., Hawkins P. T., Brewster G., Michell R. H., Kirk C. J. Rapid breakdown of phosphatidylinositol 4-phosphate and phosphatidylinositol 4,5-bisphosphate in rat hepatocytes stimulated by vasopressin and other Ca2+-mobilizing hormones. Biochem J. 1983 Jun 15;212(3):733–747. doi: 10.1042/bj2120733. [DOI] [PMC free article] [PubMed] [Google Scholar]
- Dawson A. P., Hills G., Comerford J. G. The mechanism of action of GTP on Ca2+ efflux from rat liver microsomal vesicles. Biochem J. 1987 May 15;244(1):87–92. doi: 10.1042/bj2440087. [DOI] [PMC free article] [PubMed] [Google Scholar]
- Enouf J., Giraud F., Bredoux R., Bourdeau N., Levy-Toledano S. Possible role of a cAMP-dependent phosphorylation in the calcium release mediated by inositol 1,4,5-trisphosphate in human platelet membrane vesicles. Biochim Biophys Acta. 1987 Apr 2;928(1):76–82. doi: 10.1016/0167-4889(87)90087-5. [DOI] [PubMed] [Google Scholar]
- Ferris C. D., Huganir R. L., Bredt D. S., Cameron A. M., Snyder S. H. Inositol trisphosphate receptor: phosphorylation by protein kinase C and calcium calmodulin-dependent protein kinases in reconstituted lipid vesicles. Proc Natl Acad Sci U S A. 1991 Mar 15;88(6):2232–2235. doi: 10.1073/pnas.88.6.2232. [DOI] [PMC free article] [PubMed] [Google Scholar]
- Ghosh T. K., Mullaney J. M., Tarazi F. I., Gill D. L. GTP-activated communication between distinct inositol 1,4,5-trisphosphate-sensitive and -insensitive calcium pools. Nature. 1989 Jul 20;340(6230):236–239. doi: 10.1038/340236a0. [DOI] [PubMed] [Google Scholar]
- Glennon M. C., Bird G. S., Kwan C. Y., Putney J. W., Jr Actions of vasopressin and the Ca(2+)-ATPase inhibitor, thapsigargin, on Ca2+ signaling in hepatocytes. J Biol Chem. 1992 Apr 25;267(12):8230–8233. [PubMed] [Google Scholar]
- Grossman W., Jones D., McLaurin L. P. Wall stress and patterns of hypertrophy in the human left ventricle. J Clin Invest. 1975 Jul;56(1):56–64. doi: 10.1172/JCI108079. [DOI] [PMC free article] [PubMed] [Google Scholar]
- Grynkiewicz G., Poenie M., Tsien R. Y. A new generation of Ca2+ indicators with greatly improved fluorescence properties. J Biol Chem. 1985 Mar 25;260(6):3440–3450. [PubMed] [Google Scholar]
- Jenkinson D. H., Koller K. Interactions between the effects of alpha- and beta-adrenoceptor agonists and adenine nucleotides on the membrane potential of cells in guinea-pig liver slices. Br J Pharmacol. 1977 Jan;59(1):163–175. doi: 10.1111/j.1476-5381.1977.tb06991.x. [DOI] [PMC free article] [PubMed] [Google Scholar]
- Kawanishi T., Blank L. M., Harootunian A. T., Smith M. T., Tsien R. Y. Ca2+ oscillations induced by hormonal stimulation of individual fura-2-loaded hepatocytes. J Biol Chem. 1989 Aug 5;264(22):12859–12866. [PubMed] [Google Scholar]
- Kwan C. Y., Putney J. W., Jr Uptake and intracellular sequestration of divalent cations in resting and methacholine-stimulated mouse lacrimal acinar cells. Dissociation by Sr2+ and Ba2+ of agonist-stimulated divalent cation entry from the refilling of the agonist-sensitive intracellular pool. J Biol Chem. 1990 Jan 15;265(2):678–684. [PubMed] [Google Scholar]
- Mauger J. P., Claret M., Pietri F., Hilly M. Hormonal regulation of inositol 1,4,5-trisphosphate receptor in rat liver. J Biol Chem. 1989 May 25;264(15):8821–8826. [PubMed] [Google Scholar]
- Meyer T., Stryer L. Transient calcium release induced by successive increments of inositol 1,4,5-trisphosphate. Proc Natl Acad Sci U S A. 1990 May;87(10):3841–3845. doi: 10.1073/pnas.87.10.3841. [DOI] [PMC free article] [PubMed] [Google Scholar]
- Missiaen L., De Smedt H., Droogmans G., Casteels R. Ca2+ release induced by inositol 1,4,5-trisphosphate is a steady-state phenomenon controlled by luminal Ca2+ in permeabilized cells. Nature. 1992 Jun 18;357(6379):599–602. doi: 10.1038/357599a0. [DOI] [PubMed] [Google Scholar]
- Morgan N. G., Charest R., Blackmore P. F., Exton J. H. Potentiation of alpha 1-adrenergic responses in rat liver by a cAMP-dependent mechanism. Proc Natl Acad Sci U S A. 1984 Jul;81(13):4208–4212. doi: 10.1073/pnas.81.13.4208. [DOI] [PMC free article] [PubMed] [Google Scholar]
- Muallem S., Pandol S. J., Beeker T. G. Hormone-evoked calcium release from intracellular stores is a quantal process. J Biol Chem. 1989 Jan 5;264(1):205–212. [PubMed] [Google Scholar]
- Nunn D. L., Taylor C. W. Luminal Ca2+ increases the sensitivity of Ca2+ stores to inositol 1,4,5-trisphosphate. Mol Pharmacol. 1992 Jan;41(1):115–119. [PubMed] [Google Scholar]
- Oldershaw K. A., Nunn D. L., Taylor C. W. Quantal Ca2+ mobilization stimulated by inositol 1,4,5-trisphosphate in permeabilized hepatocytes. Biochem J. 1991 Sep 15;278(Pt 3):705–708. doi: 10.1042/bj2780705. [DOI] [PMC free article] [PubMed] [Google Scholar]
- Pittner R. A., Fain J. N. Exposure of cultured hepatocytes to cyclic AMP enhances the vasopressin-mediated stimulation of inositol phosphate production. Biochem J. 1989 Jan 15;257(2):455–460. doi: 10.1042/bj2570455. [DOI] [PMC free article] [PubMed] [Google Scholar]
- Pittner R. A., Fain J. N. Vasopressin and norepinephrine stimulation of inositol phosphate accumulation in rat hepatocytes are modified differently by protein f1nase C and protein kinase A. Biochim Biophys Acta. 1990 Apr 2;1043(2):211–217. doi: 10.1016/0005-2760(90)90298-c. [DOI] [PubMed] [Google Scholar]
- Poggioli J., Mauger J. P., Claret M. Effect of cyclic AMP-dependent hormones and Ca2+-mobilizing hormones on the Ca2+ influx and polyphosphoinositide metabolism in isolated rat hepatocytes. Biochem J. 1986 May 1;235(3):663–669. doi: 10.1042/bj2350663. [DOI] [PMC free article] [PubMed] [Google Scholar]
- Rooney T. A., Renard D. C., Sass E. J., Thomas A. P. Oscillatory cytosolic calcium waves independent of stimulated inositol 1,4,5-trisphosphate formation in hepatocytes. J Biol Chem. 1991 Jul 5;266(19):12272–12282. [PubMed] [Google Scholar]
- Rooney T. A., Sass E. J., Thomas A. P. Agonist-induced cytosolic calcium oscillations originate from a specific locus in single hepatocytes. J Biol Chem. 1990 Jun 25;265(18):10792–10796. [PubMed] [Google Scholar]
- Rooney T. A., Sass E. J., Thomas A. P. Characterization of cytosolic calcium oscillations induced by phenylephrine and vasopressin in single fura-2-loaded hepatocytes. J Biol Chem. 1989 Oct 15;264(29):17131–17141. [PubMed] [Google Scholar]
- Schöfl C., Sanchez-Bueno A., Brabant G., Cobbold P. H., Cuthbertson K. S. Frequency and amplitude enhancement of calcium transients by cyclic AMP in hepatocytes. Biochem J. 1991 Feb 1;273(Pt 3):799–802. doi: 10.1042/bj2730799. [DOI] [PMC free article] [PubMed] [Google Scholar]
- Staddon J. M., Hansford R. G. Evidence indicating that the glucagon-induced increase in cytoplasmic free Ca2+ concentration in hepatocytes is mediated by an increase in cyclic AMP concentration. Eur J Biochem. 1989 Jan 15;179(1):47–52. doi: 10.1111/j.1432-1033.1989.tb14519.x. [DOI] [PubMed] [Google Scholar]
- Supattapone S., Danoff S. K., Theibert A., Joseph S. K., Steiner J., Snyder S. H. Cyclic AMP-dependent phosphorylation of a brain inositol trisphosphate receptor decreases its release of calcium. Proc Natl Acad Sci U S A. 1988 Nov;85(22):8747–8750. doi: 10.1073/pnas.85.22.8747. [DOI] [PMC free article] [PubMed] [Google Scholar]
- Taylor C. W., Potter B. V. The size of inositol 1,4,5-trisphosphate-sensitive Ca2+ stores depends on inositol 1,4,5-trisphosphate concentration. Biochem J. 1990 Feb 15;266(1):189–194. doi: 10.1042/bj2660189. [DOI] [PMC free article] [PubMed] [Google Scholar]
- Taylor C. W., Richardson A. Structure and function of inositol trisphosphate receptors. Pharmacol Ther. 1991;51(1):97–137. doi: 10.1016/0163-7258(91)90043-l. [DOI] [PubMed] [Google Scholar]
- Taylor W. M., Reinhart P., Hunt N. H., Bygrave F. L. Role of 3',5'-cyclic AMP in glucagon-induced stimulation of ruthenium red-insensitive calcium transport in an endoplasmic reticulum-rich fraction of rat liver. FEBS Lett. 1980 Mar 24;112(1):92–96. doi: 10.1016/0014-5793(80)80136-0. [DOI] [PubMed] [Google Scholar]
- Thomas A. P. Enhancement of the inositol 1,4,5-trisphosphate-releasable Ca2+ pool by GTP in permeabilized hepatocytes. J Biol Chem. 1988 Feb 25;263(6):2704–2711. [PubMed] [Google Scholar]
- Thomas A. P., Renard D. C., Rooney T. A. Spatial and temporal organization of calcium signalling in hepatocytes. Cell Calcium. 1991 Feb-Mar;12(2-3):111–126. doi: 10.1016/0143-4160(91)90013-5. [DOI] [PubMed] [Google Scholar]
- Volpe P., Alderson-Lang B. H. Regulation of inositol 1,4,5-trisphosphate-induced Ca2+ release. II. Effect of cAMP-dependent protein kinase. Am J Physiol. 1990 Jun;258(6 Pt 1):C1086–C1091. doi: 10.1152/ajpcell.1990.258.6.C1086. [DOI] [PubMed] [Google Scholar]
- Wakelam M. J., Murphy G. J., Hruby V. J., Houslay M. D. Activation of two signal-transduction systems in hepatocytes by glucagon. Nature. 1986 Sep 4;323(6083):68–71. doi: 10.1038/323068a0. [DOI] [PubMed] [Google Scholar]
- Woods N. M., Cuthbertson K. S., Cobbold P. H. Repetitive transient rises in cytoplasmic free calcium in hormone-stimulated hepatocytes. Nature. 1986 Feb 13;319(6054):600–602. doi: 10.1038/319600a0. [DOI] [PubMed] [Google Scholar]