Abstract
Four aspartic acid residues (Asp126, Asp156, Asp233 and Asp295) of Bacillus cereus sphingomyelinase (SMase) in the conservative regions were changed to glycine by in vitro mutagenesis, and the mutant SMases [D126G (Asp126-->Gly etc.), D156G, D233G and D295G] were produced in Bacillus brevis 47, a protein-producing strain. The sphingomyelin (SM)-hydrolysing activity of D295G was completely abolished and those of D126G and D156G were reduced by more than 80%, whereas that of D233G was not so profoundly affected. Two mutant enzymes (D126G and D156G) were purified and characterized further. The hydrolytic activities of D126G and D156G toward four phosphocholine-containing substrates with different hydrophobicities, SM, 2-hexadecanoylamino-4-nitrophenylphosphocholine(HNP), lysophosphatidylcholine (lysoPC) and p-nitro-phenylphosphocholine (p-NPPC), were compared with those of the wild-type. The activity of D126G toward water-soluble p-NPPC was comparable with that of the wild-type. On the other hand, D156G catalysed the hydrolysis of hydrophilic substrates such as HNP and p-NPPC more efficiently (> 4-fold) than the wild-type. These results suggested that Asp126 and Asp156, located in the highly conserved region, may well be involved in a substrate recognition process rather than catalytic action. Haemolytic activities of the mutant enzymes were found to be parallel with their SM-hydrolysing activities. Two regions, including the C-terminal region containing Asp295, were found to show considerable sequence identity with the corresponding regions of bovine pancreatic DNase I. Structural predictions indicated structural similarity between SMase and DNase I. An evolutionary relationship based on the catalytic function was suggested between the structures of these two phosphodiesterases.
Full text
PDF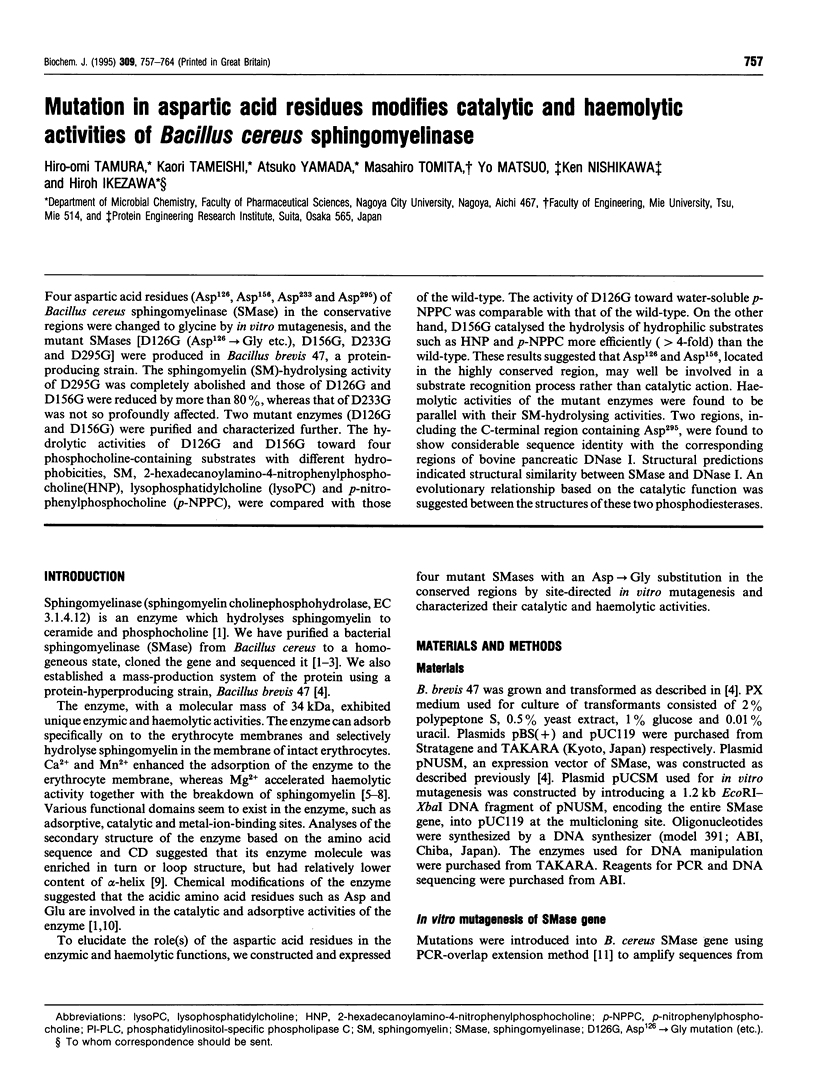
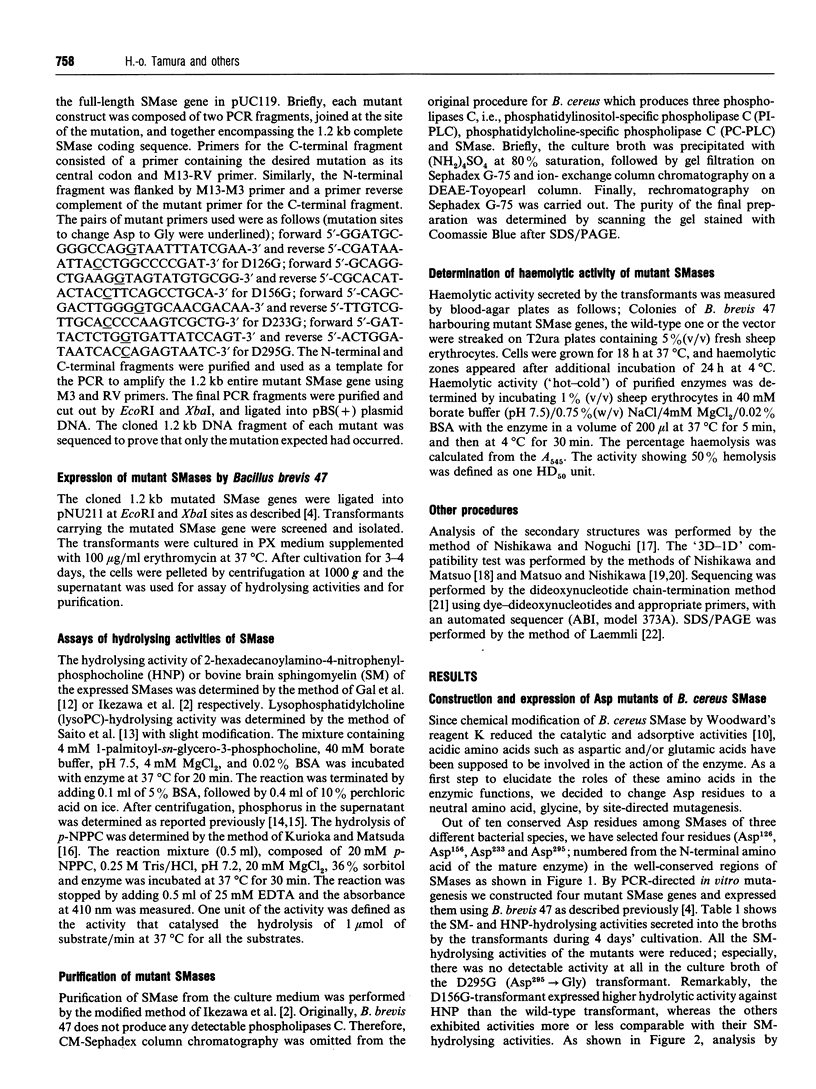
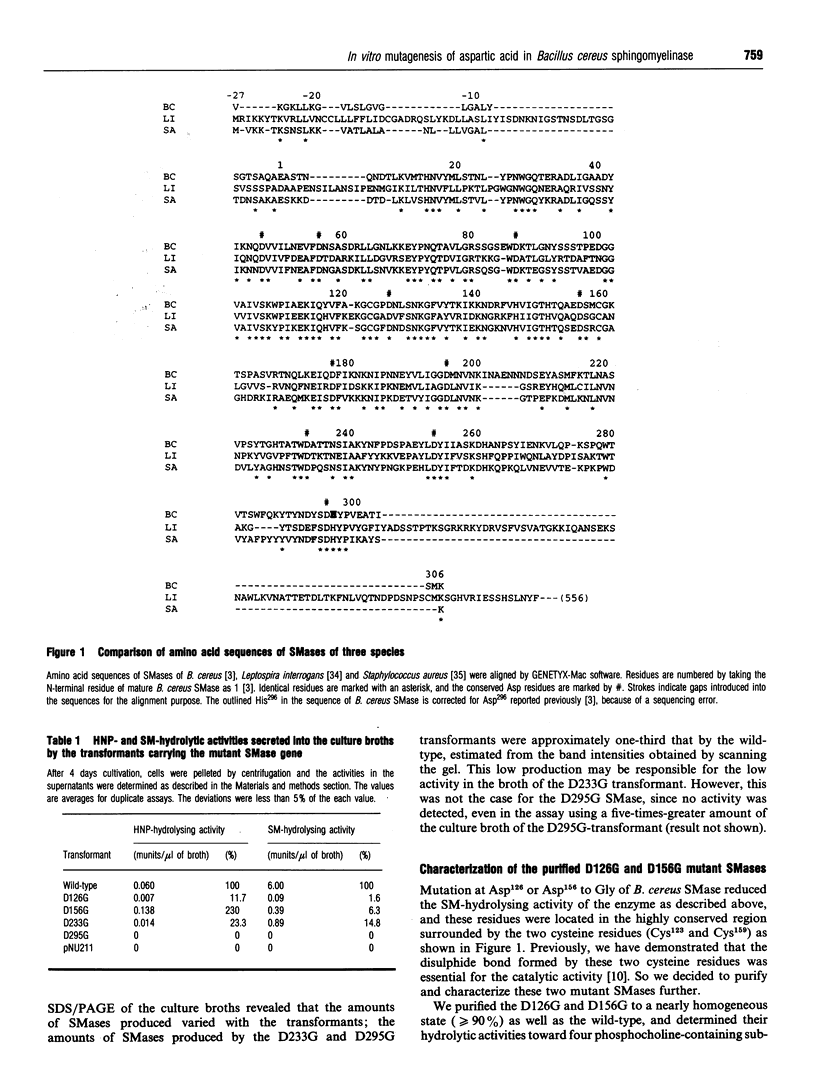
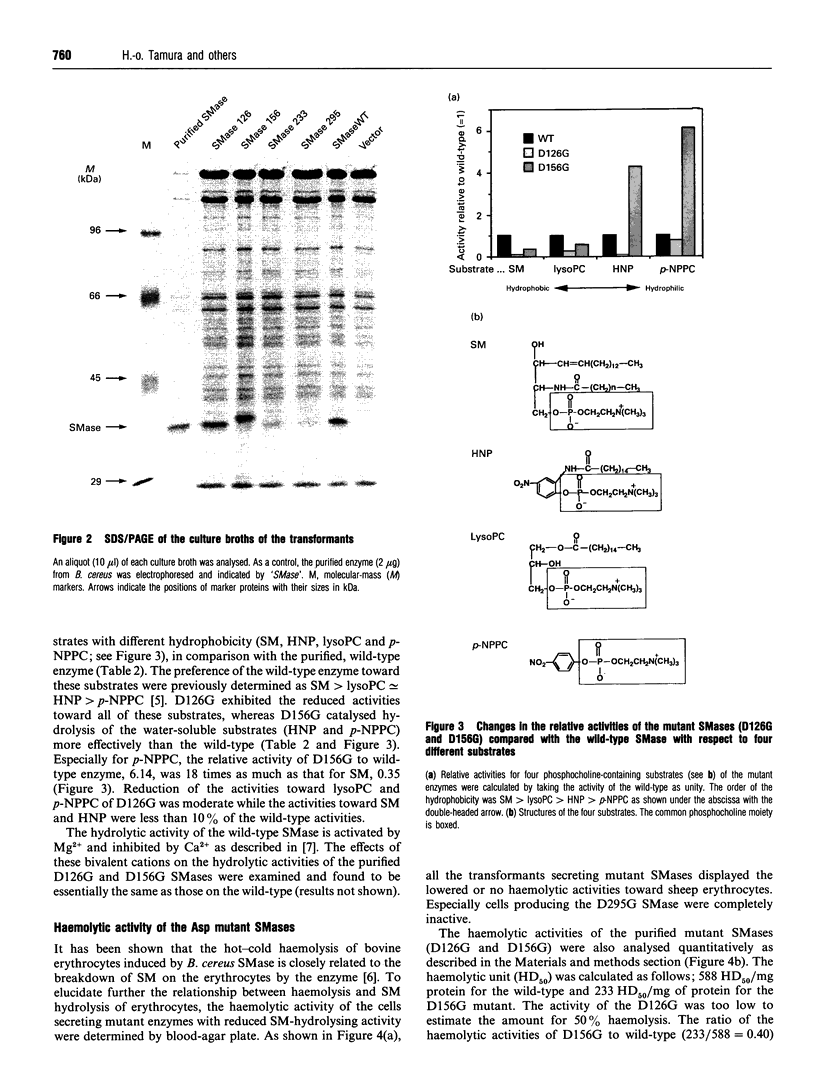
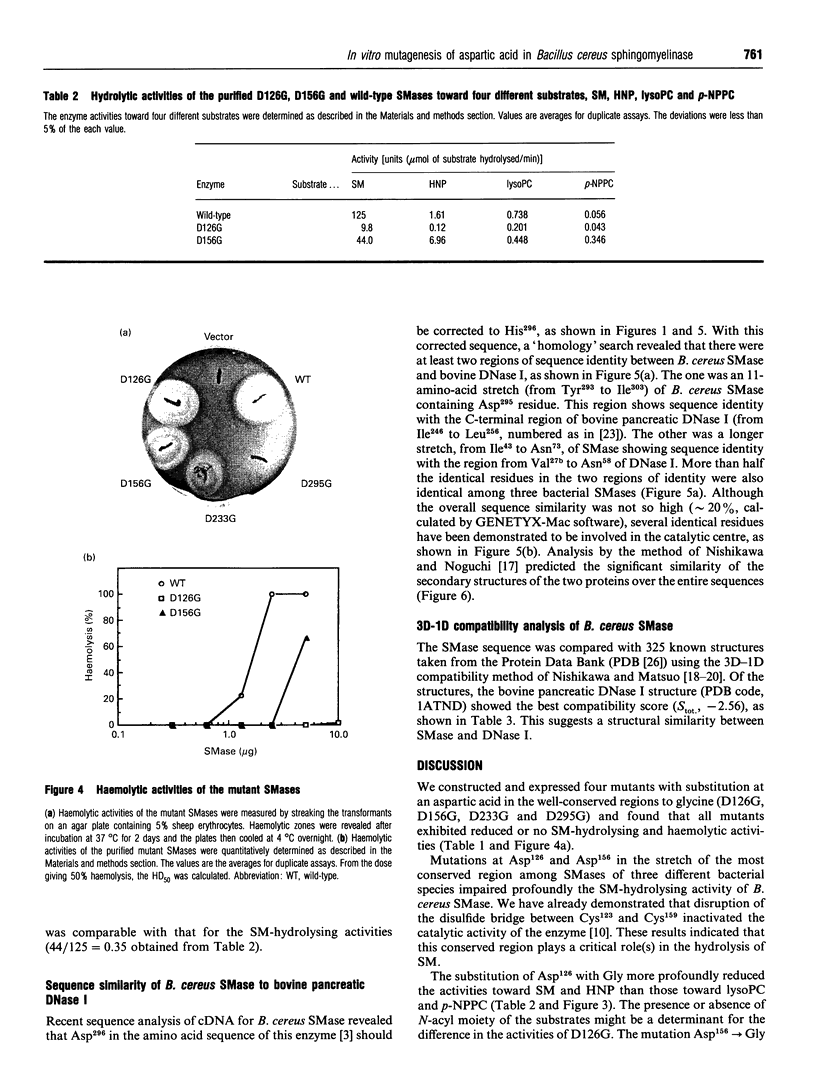
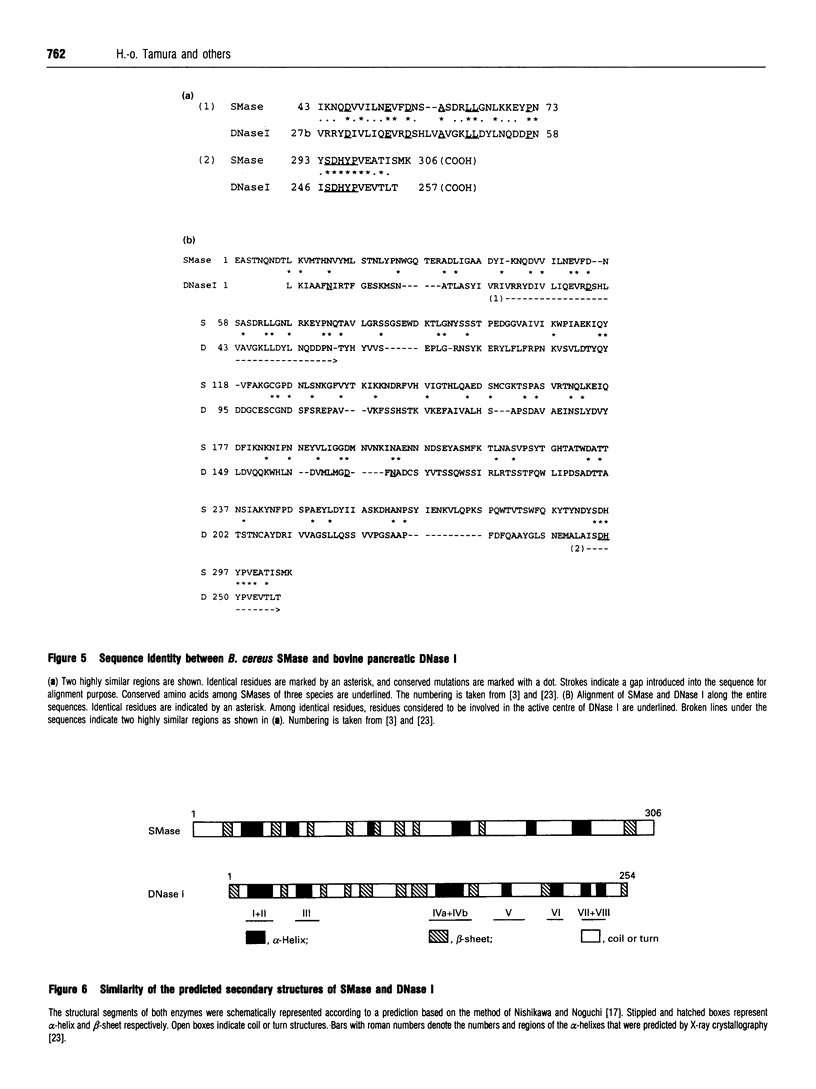
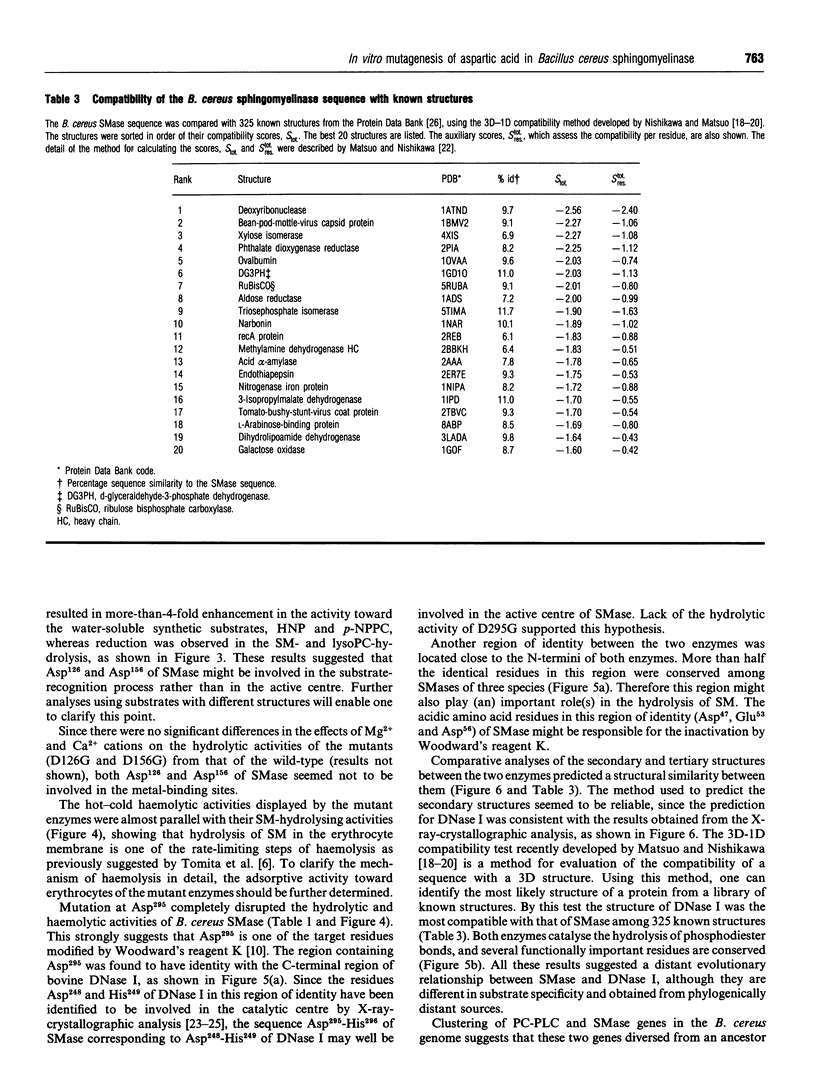
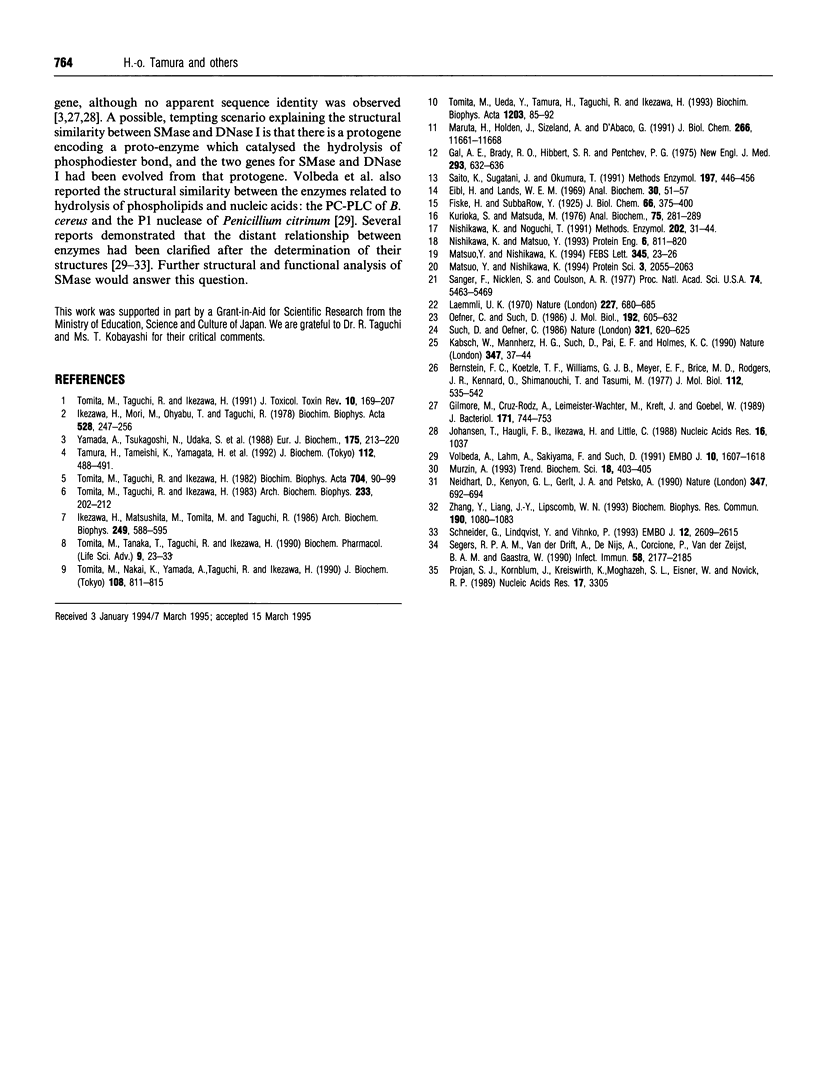
Images in this article
Selected References
These references are in PubMed. This may not be the complete list of references from this article.
- Bernstein F. C., Koetzle T. F., Williams G. J., Meyer E. F., Jr, Brice M. D., Rodgers J. R., Kennard O., Shimanouchi T., Tasumi M. The Protein Data Bank: a computer-based archival file for macromolecular structures. J Mol Biol. 1977 May 25;112(3):535–542. doi: 10.1016/s0022-2836(77)80200-3. [DOI] [PubMed] [Google Scholar]
- Eibl H., Lands W. E. A new, sensitive determination of phosphate. Anal Biochem. 1969 Jul;30(1):51–57. doi: 10.1016/0003-2697(69)90372-8. [DOI] [PubMed] [Google Scholar]
- Gal A. E., Brady R. O., Hibbert S. R., Pentchev P. G. A practical chromogenic procedure for the detection of homozygotes and heterozygous carriers of Niemann-Pick disease. N Engl J Med. 1975 Sep 25;293(13):632–636. doi: 10.1056/NEJM197509252931304. [DOI] [PubMed] [Google Scholar]
- Gilmore M. S., Cruz-Rodz A. L., Leimeister-Wächter M., Kreft J., Goebel W. A Bacillus cereus cytolytic determinant, cereolysin AB, which comprises the phospholipase C and sphingomyelinase genes: nucleotide sequence and genetic linkage. J Bacteriol. 1989 Feb;171(2):744–753. doi: 10.1128/jb.171.2.744-753.1989. [DOI] [PMC free article] [PubMed] [Google Scholar]
- Ikezawa H., Matsushita M., Tomita M., Taguchi R. Effects of metal ions on sphingomyelinase activity of Bacillus cereus. Arch Biochem Biophys. 1986 Sep;249(2):588–595. doi: 10.1016/0003-9861(86)90037-8. [DOI] [PubMed] [Google Scholar]
- Ikezawa H., Mori M., Ohyabu T., Taguchi R. Studies on sphingomyelinase of Bacillus cereus. I. Purification and properties. Biochim Biophys Acta. 1978 Feb 27;528(2):247–256. [PubMed] [Google Scholar]
- Kabsch W., Mannherz H. G., Suck D., Pai E. F., Holmes K. C. Atomic structure of the actin:DNase I complex. Nature. 1990 Sep 6;347(6288):37–44. doi: 10.1038/347037a0. [DOI] [PubMed] [Google Scholar]
- Kurioka S., Matsuda M. Phospholipase C assay using p-nitrophenylphosphoryl-choline together with sorbitol and its application to studying the metal and detergent requirement of the enzyme. Anal Biochem. 1976 Sep;75(1):281–289. doi: 10.1016/0003-2697(76)90078-6. [DOI] [PubMed] [Google Scholar]
- Laemmli U. K. Cleavage of structural proteins during the assembly of the head of bacteriophage T4. Nature. 1970 Aug 15;227(5259):680–685. doi: 10.1038/227680a0. [DOI] [PubMed] [Google Scholar]
- Maruta H., Holden J., Sizeland A., D'Abaco G. The residues of Ras and Rap proteins that determine their GAP specificities. J Biol Chem. 1991 Jun 25;266(18):11661–11668. [PubMed] [Google Scholar]
- Matsuo Y., Nishikawa K. Prediction of the structural similarity between spermidine/putrescine-binding protein and maltose-binding protein. FEBS Lett. 1994 May 23;345(1):23–26. doi: 10.1016/0014-5793(94)00435-8. [DOI] [PubMed] [Google Scholar]
- Matsuo Y., Nishikawa K. Protein structural similarities predicted by a sequence-structure compatibility method. Protein Sci. 1994 Nov;3(11):2055–2063. doi: 10.1002/pro.5560031118. [DOI] [PMC free article] [PubMed] [Google Scholar]
- Murzin A. G. Can homologous proteins evolve different enzymatic activities? Trends Biochem Sci. 1993 Nov;18(11):403–405. doi: 10.1016/0968-0004(93)90132-7. [DOI] [PubMed] [Google Scholar]
- Neidhart D. J., Kenyon G. L., Gerlt J. A., Petsko G. A. Mandelate racemase and muconate lactonizing enzyme are mechanistically distinct and structurally homologous. Nature. 1990 Oct 18;347(6294):692–694. doi: 10.1038/347692a0. [DOI] [PubMed] [Google Scholar]
- Nishikawa K., Matsuo Y. Development of pseudoenergy potentials for assessing protein 3-D-1-D compatibility and detecting weak homologies. Protein Eng. 1993 Nov;6(8):811–820. doi: 10.1093/protein/6.8.811. [DOI] [PubMed] [Google Scholar]
- Nishikawa K., Noguchi T. Predicting protein secondary structure based on amino acid sequence. Methods Enzymol. 1991;202:31–44. doi: 10.1016/0076-6879(91)02005-t. [DOI] [PubMed] [Google Scholar]
- Oefner C., Suck D. Crystallographic refinement and structure of DNase I at 2 A resolution. J Mol Biol. 1986 Dec 5;192(3):605–632. doi: 10.1016/0022-2836(86)90280-9. [DOI] [PubMed] [Google Scholar]
- Projan S. J., Kornblum J., Kreiswirth B., Moghazeh S. L., Eisner W., Novick R. P. Nucleotide sequence: the beta-hemolysin gene of Staphylococcus aureus. Nucleic Acids Res. 1989 Apr 25;17(8):3305–3305. doi: 10.1093/nar/17.8.3305. [DOI] [PMC free article] [PubMed] [Google Scholar]
- Saito K., Sugatani J., Okumura T. Phospholipase B from Penicillium notatum. Methods Enzymol. 1991;197:446–456. doi: 10.1016/0076-6879(91)97170-4. [DOI] [PubMed] [Google Scholar]
- Sanger F., Nicklen S., Coulson A. R. DNA sequencing with chain-terminating inhibitors. Proc Natl Acad Sci U S A. 1977 Dec;74(12):5463–5467. doi: 10.1073/pnas.74.12.5463. [DOI] [PMC free article] [PubMed] [Google Scholar]
- Schneider G., Lindqvist Y., Vihko P. Three-dimensional structure of rat acid phosphatase. EMBO J. 1993 Jul;12(7):2609–2615. doi: 10.1002/j.1460-2075.1993.tb05921.x. [DOI] [PMC free article] [PubMed] [Google Scholar]
- Segers R. P., van der Drift A., de Nijs A., Corcione P., van der Zeijst B. A., Gaastra W. Molecular analysis of a sphingomyelinase C gene from Leptospira interrogans serovar hardjo. Infect Immun. 1990 Jul;58(7):2177–2185. doi: 10.1128/iai.58.7.2177-2185.1990. [DOI] [PMC free article] [PubMed] [Google Scholar]
- Suck D., Oefner C. Structure of DNase I at 2.0 A resolution suggests a mechanism for binding to and cutting DNA. Nature. 1986 Jun 5;321(6070):620–625. doi: 10.1038/321620a0. [DOI] [PubMed] [Google Scholar]
- Tamura H., Tameishi K., Yamagata H., Udaka S., Kobayashi T., Tomita M., Ikezawa H. Mass production of sphingomyelinase of Bacillus cereus by a protein-hyperproducing strain, Bacillus brevis 47, and its purification. J Biochem. 1992 Oct;112(4):488–491. doi: 10.1093/oxfordjournals.jbchem.a123926. [DOI] [PubMed] [Google Scholar]
- Tomita M., Nakai K., Yamada A., Taguchi R., Ikezawa H. Secondary structure of sphingomyelinase from Bacillus cereus. J Biochem. 1990 Nov;108(5):811–815. doi: 10.1093/oxfordjournals.jbchem.a123285. [DOI] [PubMed] [Google Scholar]
- Tomita M., Taguchi R., Ikezawa H. Adsorption of sphingomyelinase of Bacillus cereus onto erythrocyte membranes. Arch Biochem Biophys. 1983 May;223(1):202–212. doi: 10.1016/0003-9861(83)90586-6. [DOI] [PubMed] [Google Scholar]
- Tomita M., Taguchi R., Ikezawa H. Molecular properties and kinetic studies on sphingomyelinase of Bacillus cereus. Biochim Biophys Acta. 1982 May 21;704(1):90–99. doi: 10.1016/0167-4838(82)90135-2. [DOI] [PubMed] [Google Scholar]
- Tomita M., Ueda Y., Tamura H., Taguchi R., Ikezawa H. The role of acidic amino-acid residues in catalytic and adsorptive sites of Bacillus cereus sphingomyelinase. Biochim Biophys Acta. 1993 Nov 10;1203(1):85–92. doi: 10.1016/0167-4838(93)90039-t. [DOI] [PubMed] [Google Scholar]
- Volbeda A., Lahm A., Sakiyama F., Suck D. Crystal structure of Penicillium citrinum P1 nuclease at 2.8 A resolution. EMBO J. 1991 Jul;10(7):1607–1618. doi: 10.1002/j.1460-2075.1991.tb07683.x. [DOI] [PMC free article] [PubMed] [Google Scholar]
- Yamada A., Tsukagoshi N., Udaka S., Sasaki T., Makino S., Nakamura S., Little C., Tomita M., Ikezawa H. Nucleotide sequence and expression in Escherichia coli of the gene coding for sphingomyelinase of Bacillus cereus. Eur J Biochem. 1988 Aug 1;175(2):213–220. doi: 10.1111/j.1432-1033.1988.tb14186.x. [DOI] [PubMed] [Google Scholar]
- Zhang Y., Liang J. Y., Lipscomb W. N. Structural similarities between fructose-1,6-bisphosphatase and inositol monophosphatase. Biochem Biophys Res Commun. 1993 Feb 15;190(3):1080–1083. doi: 10.1006/bbrc.1993.1159. [DOI] [PubMed] [Google Scholar]