Abstract
Purification and biophysical characterization of mitochondrial creatine kinase (Mi-CK) from sperm of the sea urchin Psammechinus miliaris, as well as gel-permeation chromatography of human heart Mi-CK demonstrate that these two Mi-CK isoenzymes form highly symmetrical octameric molecules with an M(r) of approx. 350,000, a value similar to that found for all other Mi-CK isoenzymes investigated so far. The absolute evolutionary conservation of this oligomeric form from sea urchins to mammals points both to its essentiality for Mi-CK function and to an important role of octameric Mi-CK in the energy metabolism of tissues and cells with high and fluctuating energy demands. To investigate whether a similar physiological principle also operates in an even more distantly related animal phylum, the arginine kinase (ArgK) isoenzyme system of Drosophila flight muscle was investigated with two independent subcellular fractionation procedures and subsequent analysis of the fractions by SDS/PAGE, immunoblotting and native isoenzyme electrophoresis. In contrast with a previous report [Munneke and Collier (1988) Biochem. Genet. 26, 131-141], strong evidence against the occurrence of a Mi-ArgK isoenzyme in Drosophila was obtained. The findings of the present study are discussed in the context of CK and ArgK function in general and of structural and bioenergetic differences between vertebrate striated muscles and arthropod flight muscles.
Full text
PDF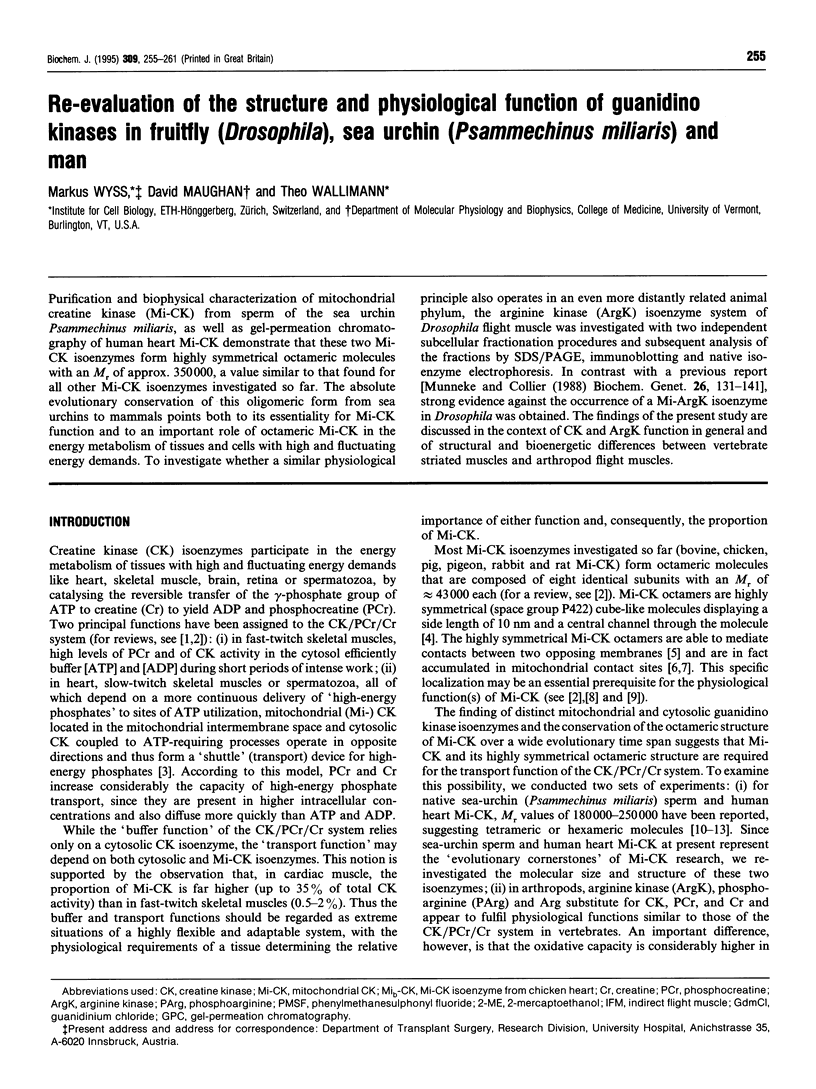
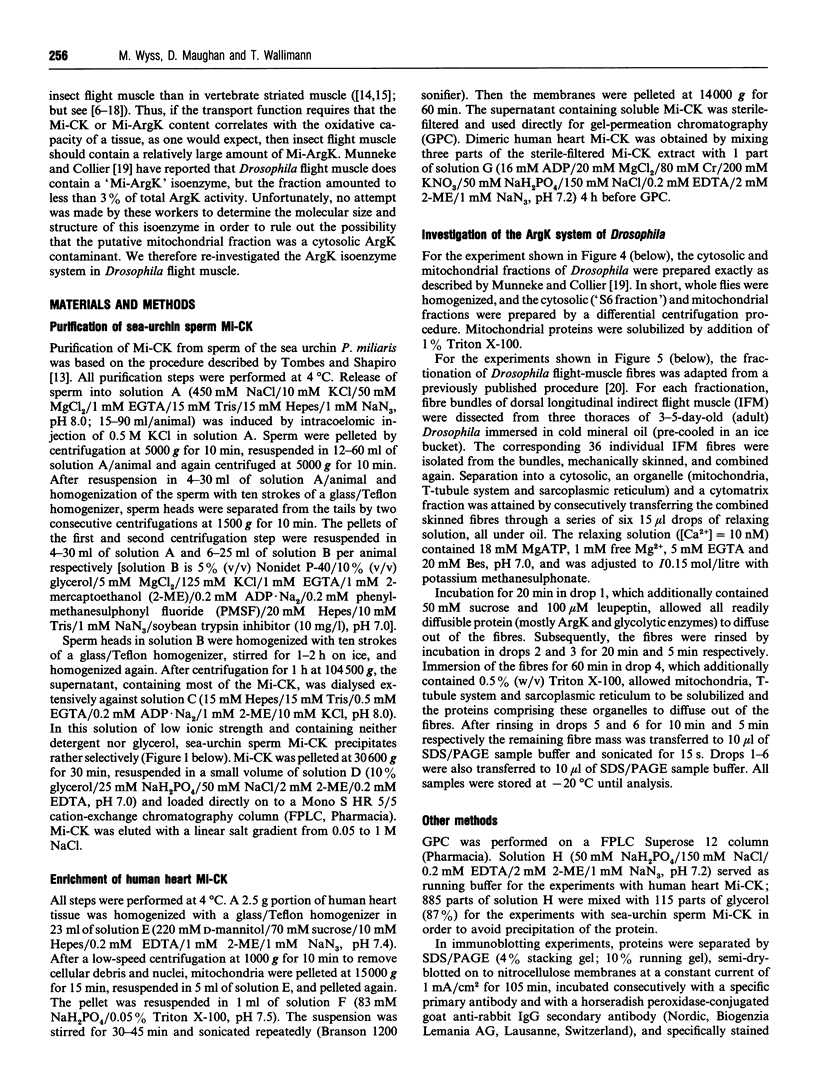
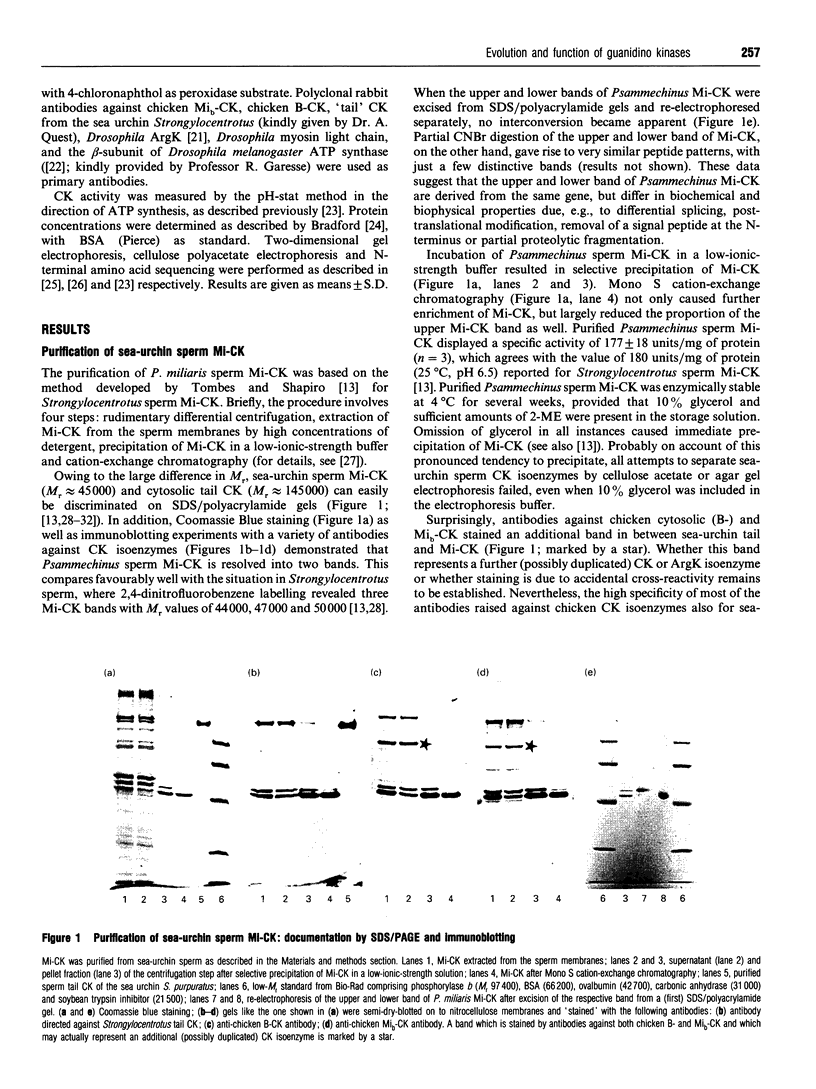
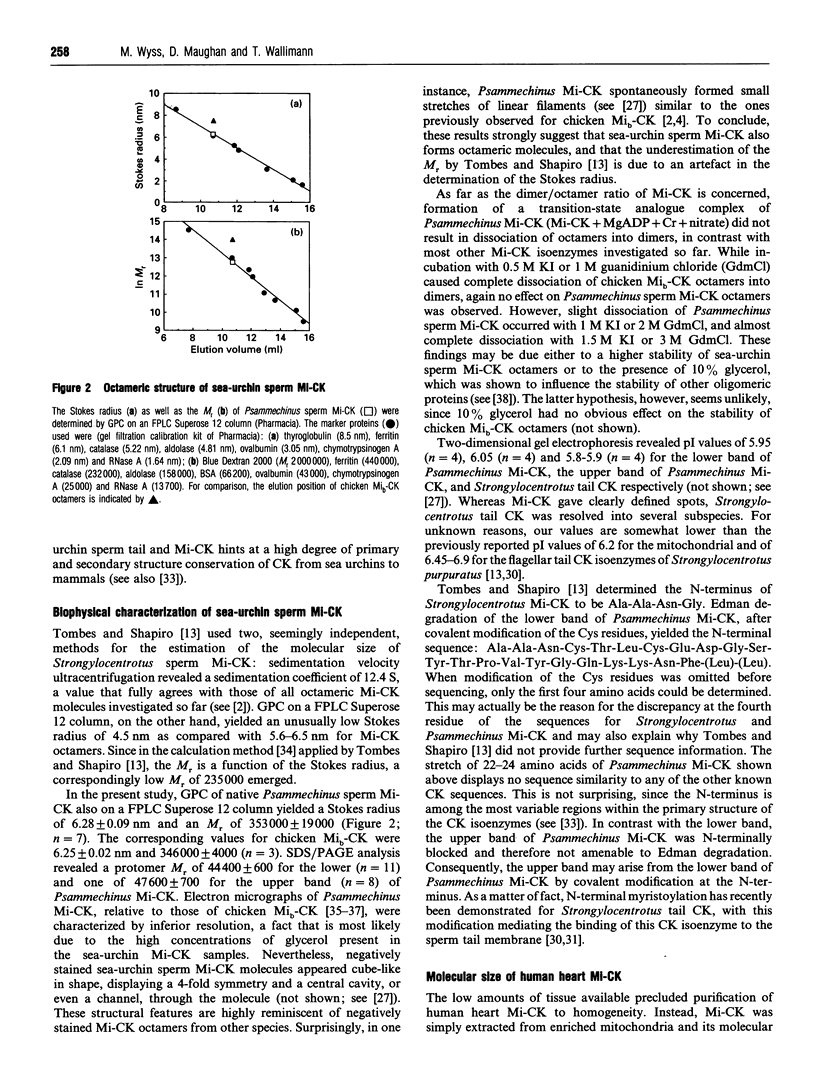
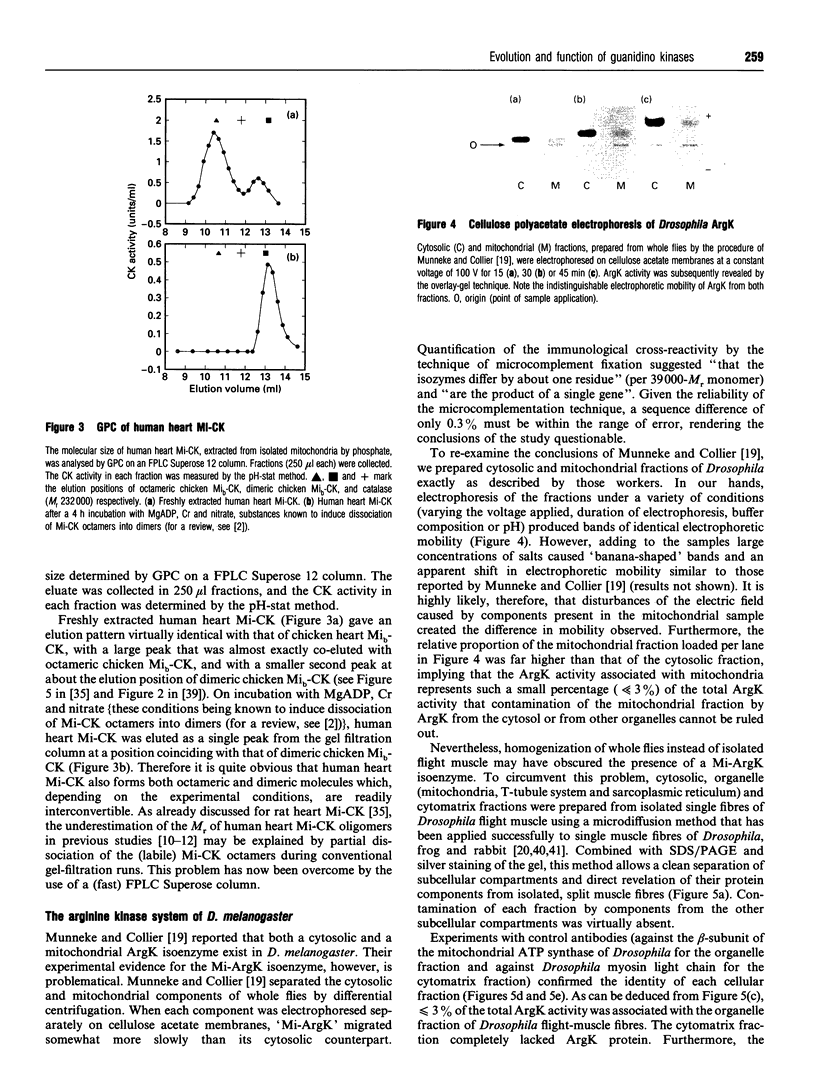
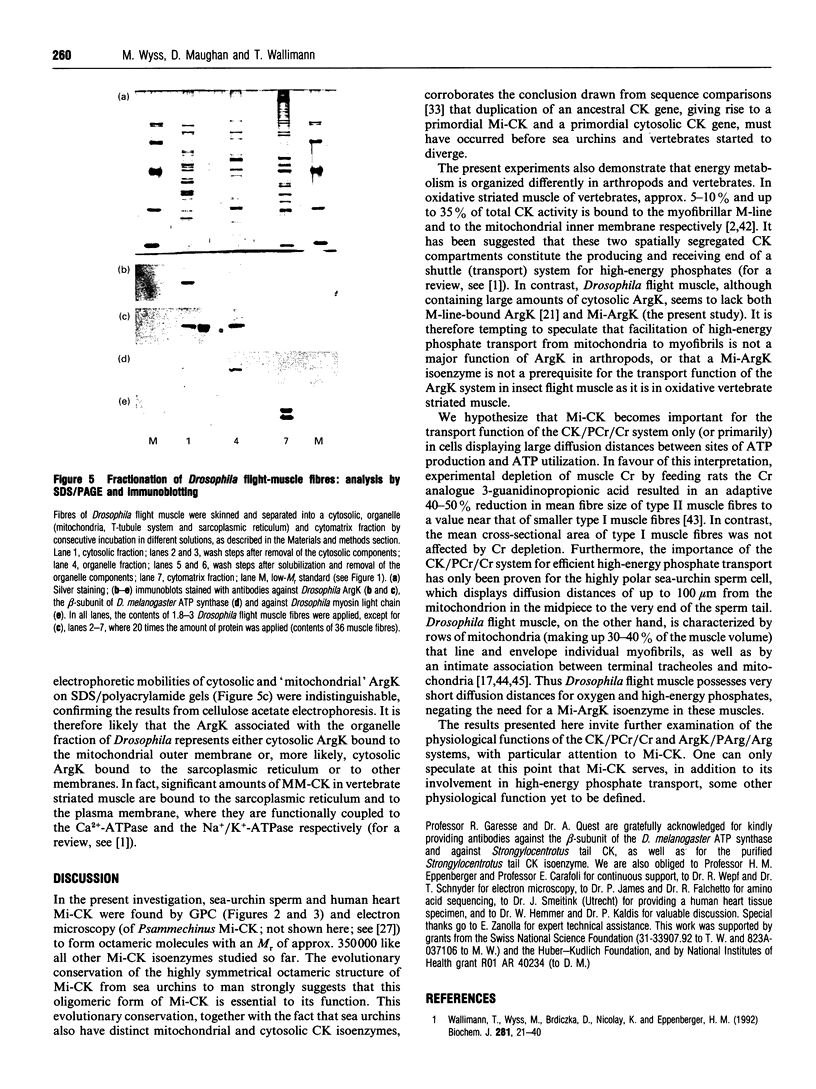
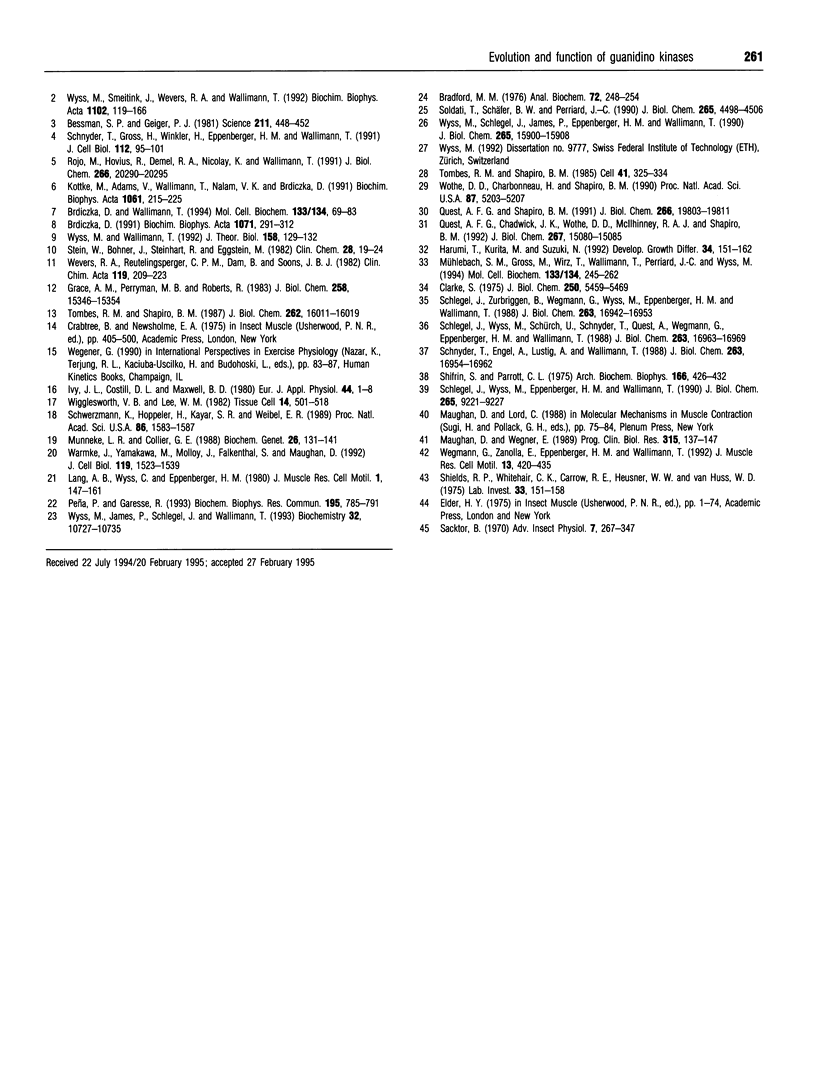
Images in this article
Selected References
These references are in PubMed. This may not be the complete list of references from this article.
- Bessman S. P., Geiger P. J. Transport of energy in muscle: the phosphorylcreatine shuttle. Science. 1981 Jan 30;211(4481):448–452. doi: 10.1126/science.6450446. [DOI] [PubMed] [Google Scholar]
- Bradford M. M. A rapid and sensitive method for the quantitation of microgram quantities of protein utilizing the principle of protein-dye binding. Anal Biochem. 1976 May 7;72:248–254. doi: 10.1006/abio.1976.9999. [DOI] [PubMed] [Google Scholar]
- Brdiczka D. Contact sites between mitochondrial envelope membranes. Structure and function in energy- and protein-transfer. Biochim Biophys Acta. 1991 Nov 13;1071(3):291–312. doi: 10.1016/0304-4157(91)90018-r. [DOI] [PubMed] [Google Scholar]
- Brdiczka D., Wallimann T. The importance of the outer mitochondrial compartment in regulation of energy metabolism. Mol Cell Biochem. 1994 Apr-May;133-134:69–83. doi: 10.1007/BF01267948. [DOI] [PubMed] [Google Scholar]
- Clarke S. The size and detergent binding of membrane proteins. J Biol Chem. 1975 Jul 25;250(14):5459–5469. [PubMed] [Google Scholar]
- Grace A. M., Perryman M. B., Roberts R. Purification and characterization of human mitochondrial creatine kinase. A single enzyme form. J Biol Chem. 1983 Dec 25;258(24):15346–15354. [PubMed] [Google Scholar]
- Ivy J. L., Costill D. L., Maxwell B. D. Skeletal muscle determinants of maximum aerobic power in man. Eur J Appl Physiol Occup Physiol. 1980;44(1):1–8. doi: 10.1007/BF00421757. [DOI] [PubMed] [Google Scholar]
- Kottke M., Adams V., Wallimann T., Nalam V. K., Brdiczka D. Location and regulation of octameric mitochondrial creatine kinase in the contact sites. Biochim Biophys Acta. 1991 Jan 30;1061(2):215–225. doi: 10.1016/0005-2736(91)90287-i. [DOI] [PubMed] [Google Scholar]
- Lang A. B., Wyss C., Eppenberger H. M. Localization of arginine kinase in muscles fibres of Drosophila melanogaster. J Muscle Res Cell Motil. 1980 Jun;1(2):147–161. doi: 10.1007/BF00711796. [DOI] [PubMed] [Google Scholar]
- Maughan D., Lord C. Protein diffusivities in skinned frog skeletal muscle fibers. Adv Exp Med Biol. 1988;226:75–84. [PubMed] [Google Scholar]
- Maughan D., Wegner E. On the organization and diffusion of glycolytic enzymes in skeletal muscle. Prog Clin Biol Res. 1989;315:137–147. [PubMed] [Google Scholar]
- Munneke L. R., Collier G. E. Cytoplasmic and mitochondrial arginine kinases in Drosophila: evidence for a single gene. Biochem Genet. 1988 Feb;26(1-2):131–141. doi: 10.1007/BF00555494. [DOI] [PubMed] [Google Scholar]
- Mühlebach S. M., Gross M., Wirz T., Wallimann T., Perriard J. C., Wyss M. Sequence homology and structure predictions of the creatine kinase isoenzymes. Mol Cell Biochem. 1994 Apr-May;133-134:245–262. doi: 10.1007/BF01267958. [DOI] [PubMed] [Google Scholar]
- Peña P., Garesse R. The beta subunit of the Drosophila melanogaster ATP synthase: cDNA cloning, amino acid analysis and identification of the protein in adult flies. Biochem Biophys Res Commun. 1993 Sep 15;195(2):785–791. doi: 10.1006/bbrc.1993.2114. [DOI] [PubMed] [Google Scholar]
- Quest A. F., Chadwick J. K., Wothe D. D., McIlhinney R. A., Shapiro B. M. Myristoylation of flagellar creatine kinase in the sperm phosphocreatine shuttle is linked to its membrane association properties. J Biol Chem. 1992 Jul 25;267(21):15080–15085. [PubMed] [Google Scholar]
- Quest A. F., Shapiro B. M. Membrane association of flagellar creatine kinase in the sperm phosphocreatine shuttle. J Biol Chem. 1991 Oct 15;266(29):19803–19811. [PubMed] [Google Scholar]
- Rojo M., Hovius R., Demel R. A., Nicolay K., Wallimann T. Mitochondrial creatine kinase mediates contact formation between mitochondrial membranes. J Biol Chem. 1991 Oct 25;266(30):20290–20295. [PubMed] [Google Scholar]
- Schlegel J., Wyss M., Eppenberger H. M., Wallimann T. Functional studies with the octameric and dimeric form of mitochondrial creatine kinase. Differential pH-dependent association of the two oligomeric forms with the inner mitochondrial membrane. J Biol Chem. 1990 Jun 5;265(16):9221–9227. [PubMed] [Google Scholar]
- Schlegel J., Wyss M., Schürch U., Schnyder T., Quest A., Wegmann G., Eppenberger H. M., Wallimann T. Mitochondrial creatine kinase from cardiac muscle and brain are two distinct isoenzymes but both form octameric molecules. J Biol Chem. 1988 Nov 15;263(32):16963–16969. [PubMed] [Google Scholar]
- Schlegel J., Zurbriggen B., Wegmann G., Wyss M., Eppenberger H. M., Wallimann T. Native mitochondrial creatine kinase forms octameric structures. I. Isolation of two interconvertible mitochondrial creatine kinase forms, dimeric and octameric mitochondrial creatine kinase: characterization, localization, and structure-function relationships. J Biol Chem. 1988 Nov 15;263(32):16942–16953. [PubMed] [Google Scholar]
- Schnyder T., Engel A., Lustig A., Wallimann T. Native mitochondrial creatine kinase forms octameric structures. II. Characterization of dimers and octamers by ultracentrifugation, direct mass measurements by scanning transmission electron microscopy, and image analysis of single mitochondrial creatine kinase octamers. J Biol Chem. 1988 Nov 15;263(32):16954–16962. [PubMed] [Google Scholar]
- Schnyder T., Gross H., Winkler H., Eppenberger H. M., Wallimann T. Structure of the mitochondrial creatine kinase octamer: high-resolution shadowing and image averaging of single molecules and formation of linear filaments under specific staining conditions. J Cell Biol. 1991 Jan;112(1):95–101. doi: 10.1083/jcb.112.1.95. [DOI] [PMC free article] [PubMed] [Google Scholar]
- Schwerzmann K., Hoppeler H., Kayar S. R., Weibel E. R. Oxidative capacity of muscle and mitochondria: correlation of physiological, biochemical, and morphometric characteristics. Proc Natl Acad Sci U S A. 1989 Mar;86(5):1583–1587. doi: 10.1073/pnas.86.5.1583. [DOI] [PMC free article] [PubMed] [Google Scholar]
- Shields R. P., Whitehair C. K., Carrow R. E., Heusner W. W., Van Huss W. D. Skeletal muscle function and structure after depletion of creatine. Lab Invest. 1975 Aug;33(2):151–158. [PubMed] [Google Scholar]
- Shifrin S., Parrott C. L. Influence of glycerol and other polyhydric alcohol on the quaternary structure of an oligometric protein. Arch Biochem Biophys. 1975 Feb;166(2):426–432. doi: 10.1016/0003-9861(75)90405-1. [DOI] [PubMed] [Google Scholar]
- Soldati T., Schäfer B. W., Perriard J. C. Alternative ribosomal initiation gives rise to chicken brain-type creatine kinase isoproteins with heterogeneous amino termini. J Biol Chem. 1990 Mar 15;265(8):4498–4506. [PubMed] [Google Scholar]
- Stein W., Bohner J., Steinhart R., Eggstein M. Macro creatine kinase: determination and differentiation of two types by their activation energies. Clin Chem. 1982 Jan;28(1):19–24. [PubMed] [Google Scholar]
- Tombes R. M., Shapiro B. M. Enzyme termini of a phosphocreatine shuttle. Purification and characterization of two creatine kinase isozymes from sea urchin sperm. J Biol Chem. 1987 Nov 25;262(33):16011–16019. [PubMed] [Google Scholar]
- Tombes R. M., Shapiro B. M. Metabolite channeling: a phosphorylcreatine shuttle to mediate high energy phosphate transport between sperm mitochondrion and tail. Cell. 1985 May;41(1):325–334. doi: 10.1016/0092-8674(85)90085-6. [DOI] [PubMed] [Google Scholar]
- Wallimann T., Wyss M., Brdiczka D., Nicolay K., Eppenberger H. M. Intracellular compartmentation, structure and function of creatine kinase isoenzymes in tissues with high and fluctuating energy demands: the 'phosphocreatine circuit' for cellular energy homeostasis. Biochem J. 1992 Jan 1;281(Pt 1):21–40. doi: 10.1042/bj2810021. [DOI] [PMC free article] [PubMed] [Google Scholar]
- Warmke J., Yamakawa M., Molloy J., Falkenthal S., Maughan D. Myosin light chain-2 mutation affects flight, wing beat frequency, and indirect flight muscle contraction kinetics in Drosophila. J Cell Biol. 1992 Dec;119(6):1523–1539. doi: 10.1083/jcb.119.6.1523. [DOI] [PMC free article] [PubMed] [Google Scholar]
- Wegmann G., Zanolla E., Eppenberger H. M., Wallimann T. In situ compartmentation of creatine kinase in intact sarcomeric muscle: the acto-myosin overlap zone as a molecular sieve. J Muscle Res Cell Motil. 1992 Aug;13(4):420–435. doi: 10.1007/BF01738037. [DOI] [PubMed] [Google Scholar]
- Wevers R. A., Reutelingsperger C. P., Dam B., Soons J. B. Mitochondrial creatine kinase (EC 2.7.3.2) in the brain. Clin Chim Acta. 1982 Mar 12;119(3):209–223. doi: 10.1016/0009-8981(82)90333-3. [DOI] [PubMed] [Google Scholar]
- Wigglesworth V. B., Lee W. M. The supply of oxygen to the flight muscles of insects: a theory of tracheole physiology. Tissue Cell. 1982;14(3):501–518. doi: 10.1016/0040-8166(82)90043-x. [DOI] [PubMed] [Google Scholar]
- Wothe D. D., Charbonneau H., Shapiro B. M. The phosphocreatine shuttle of sea urchin sperm: flagellar creatine kinase resulted from a gene triplication. Proc Natl Acad Sci U S A. 1990 Jul;87(13):5203–5207. doi: 10.1073/pnas.87.13.5203. [DOI] [PMC free article] [PubMed] [Google Scholar]
- Wyss M., Schlegel J., James P., Eppenberger H. M., Wallimann T. Mitochondrial creatine kinase from chicken brain. Purification, biophysical characterization, and generation of heterodimeric and heterooctameric molecules with subunits of other creatine kinase isoenzymes. J Biol Chem. 1990 Sep 15;265(26):15900–15908. [PubMed] [Google Scholar]
- Wyss M., Smeitink J., Wevers R. A., Wallimann T. Mitochondrial creatine kinase: a key enzyme of aerobic energy metabolism. Biochim Biophys Acta. 1992 Sep 25;1102(2):119–166. doi: 10.1016/0005-2728(92)90096-k. [DOI] [PubMed] [Google Scholar]
- Wyss M., Wallimann T. Metabolite channelling in aerobic energy metabolism. J Theor Biol. 1992 Sep 7;158(1):129–132. doi: 10.1016/s0022-5193(05)80650-2. [DOI] [PubMed] [Google Scholar]