Abstract
The possible role of post-translational modifications such as subunit oligomerization, protein glycosylation and oligosaccharide processing on the circulatory life-time of proteins was studied using recombinant human acetylcholinesterase (rHuAChE). Different preparations of rHuAChE containing various amounts of tetramers, dimers and monomers are cleared at similar rates from the circulation, suggesting that oligomerization does not play an important role in determining the rate of clearance. An engineered rHuAChE mutant containing only one N-glycosylation site was cleared from the circulation more rapidly than the wild-type triglycosylated enzyme. On the other hand, hyperglycosylated mutants containing either four or five occupied N-glycosylation sites, analagous to those present on the slowly cleared fetal bovine serum acetylcholinesterase (FBS-AChE), were also cleared more rapidly from the bloodstream than the wild-type species. Furthermore, the two different tetraglycosylated mutants were cleared at different rates while the pentaglycosylated mutant exhibited the most rapid clearance profile. These results imply that though the number of N-glycosylation sites plays a role in the circulatory life-time of the enzyme, the number of N-glycan units in itself does not determine the rate of clearance. When saturating amounts of asialofetuin were administered together with rHuAChE, the circulatory half-life of the enzyme was dramatically increased (from 80 min to 19 h) and was found to be similar to that displayed by plasma-derived cholinesterases while desialylation of these enzymes caused a sharp decrease in the circulatory half-life to approximately 3-5 min. Determination of the average number of sialic acid residues per enzyme subunit of the five different N-glycosylation species generated, revealed that the rate of clearance is not a function of the absolute number of appended sialic acid moieties but rather of the number of unoccupied sialic acid attachment sites per enzyme molecule. Specifically, we demonstrate an inverse-linear relationship between the number of vacant sialic acid attachment sites and the values of the enzyme residence time within the bloodstream.
Full text
PDF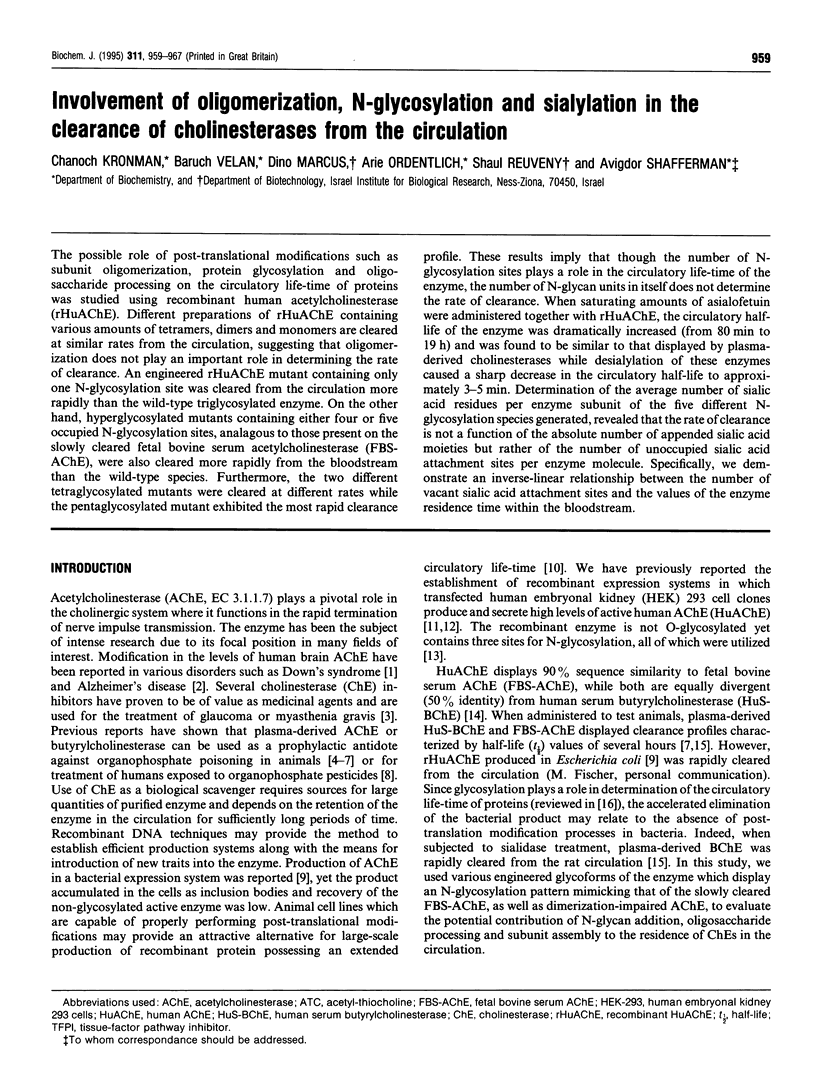
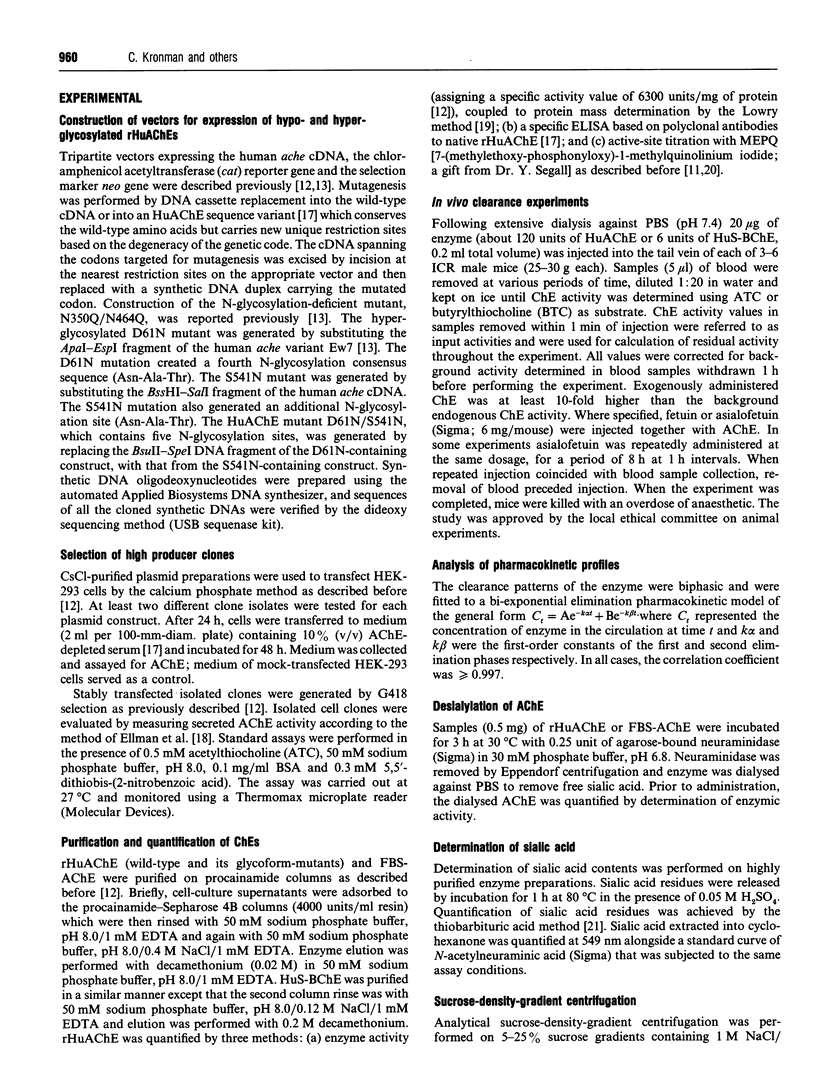
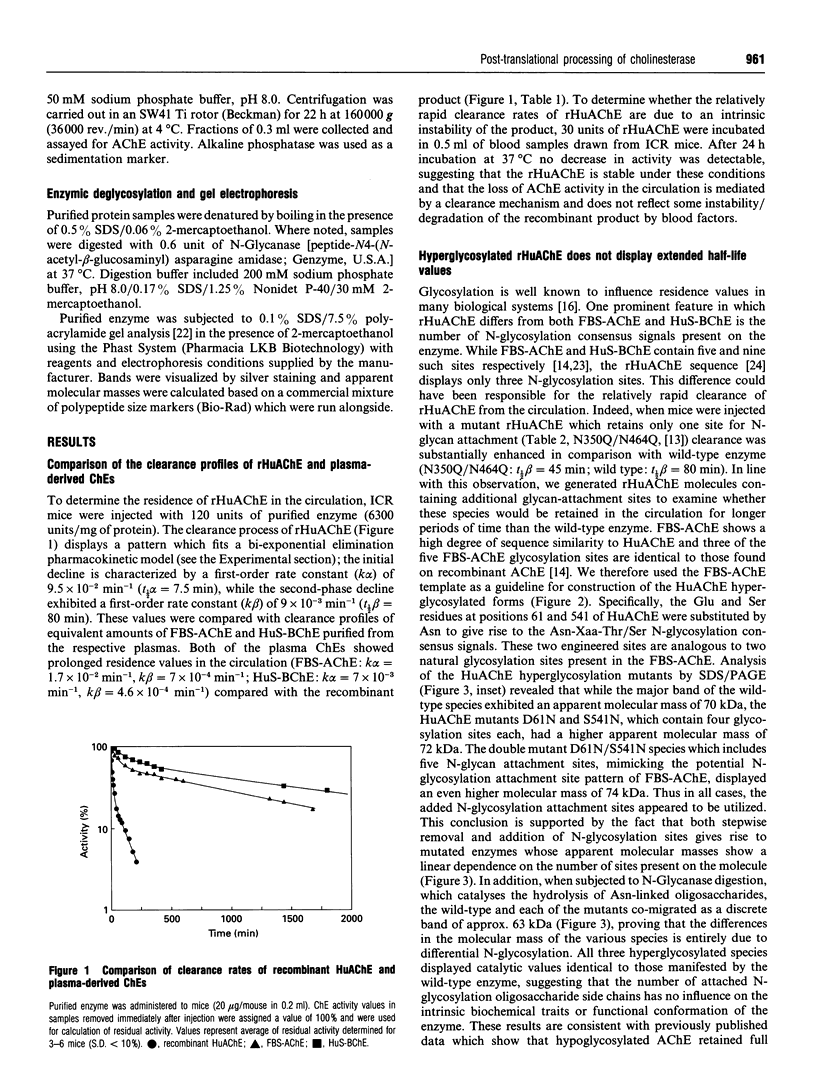
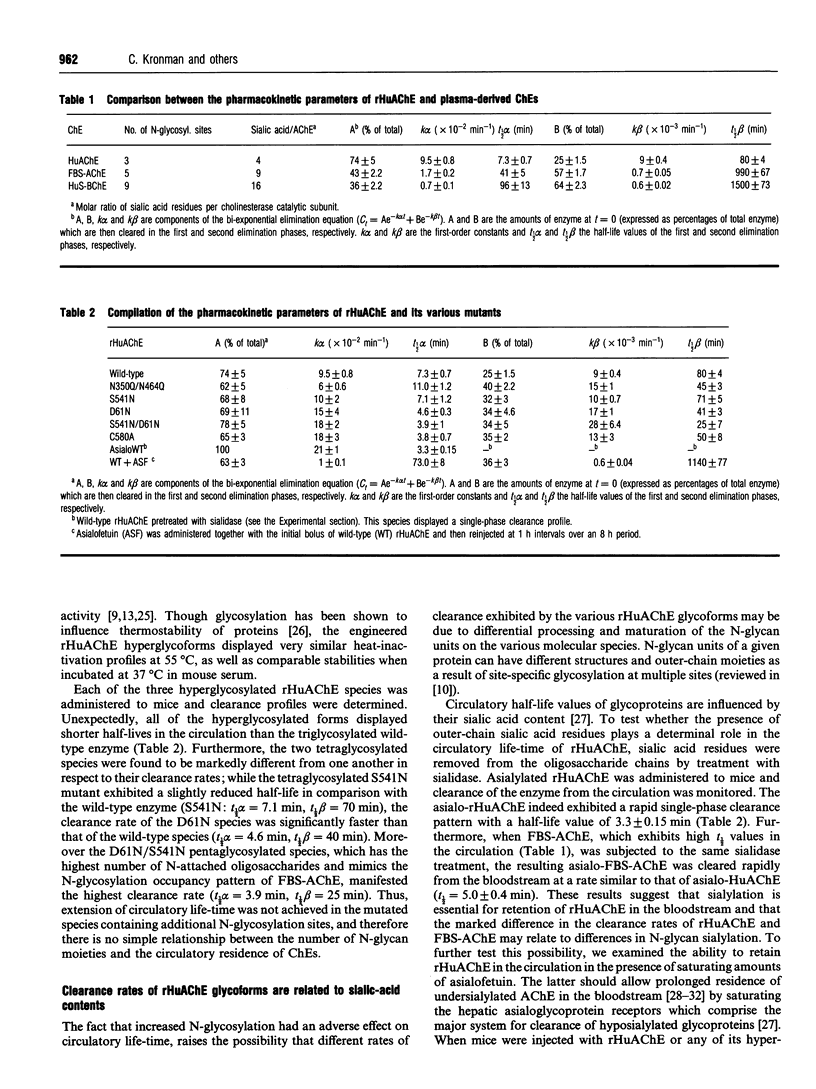
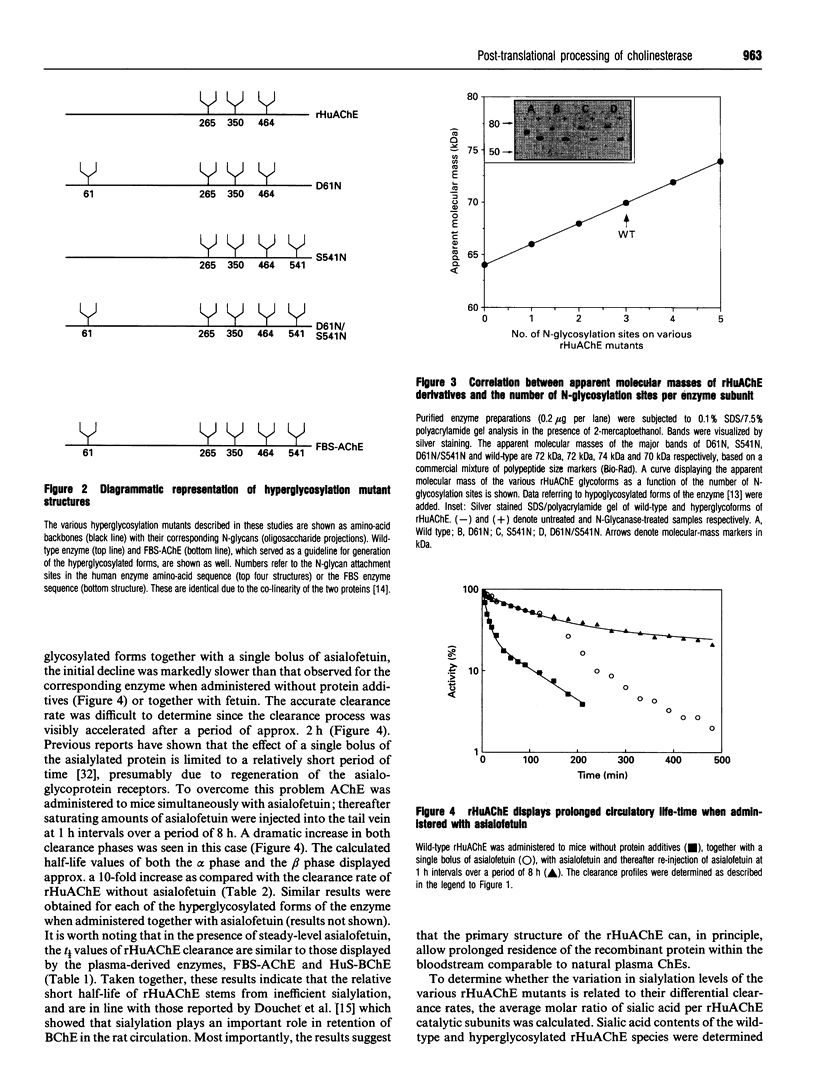
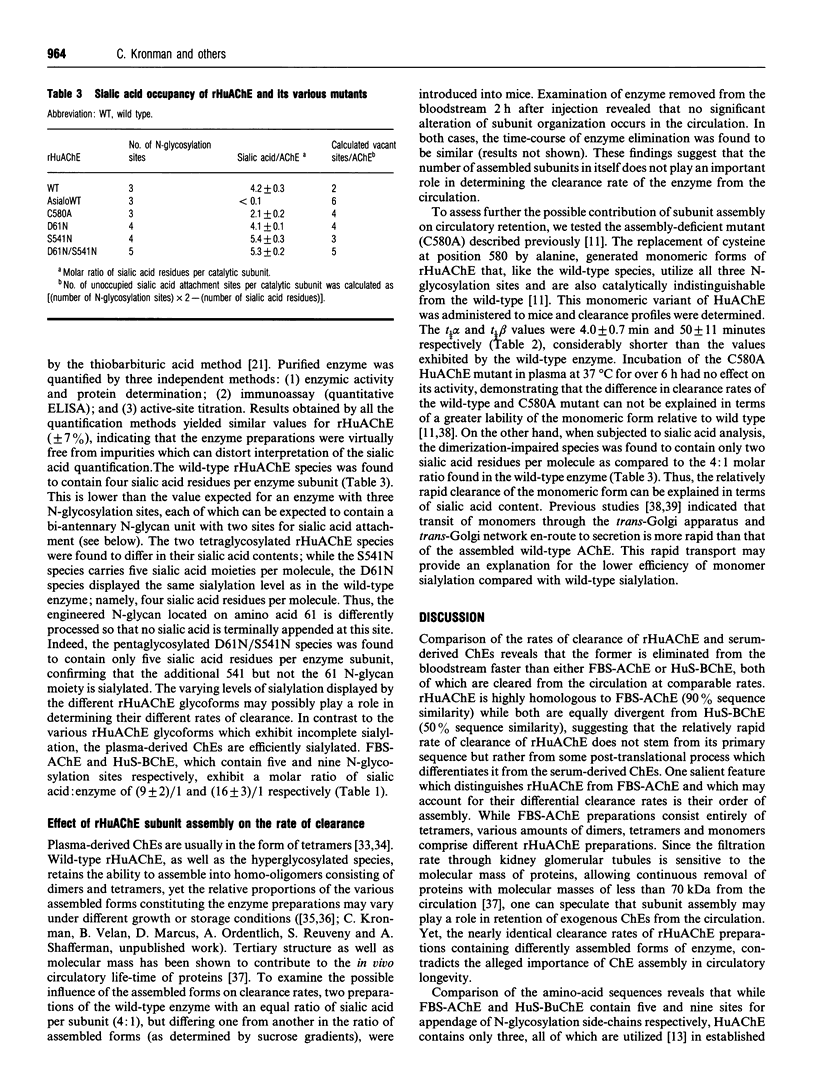
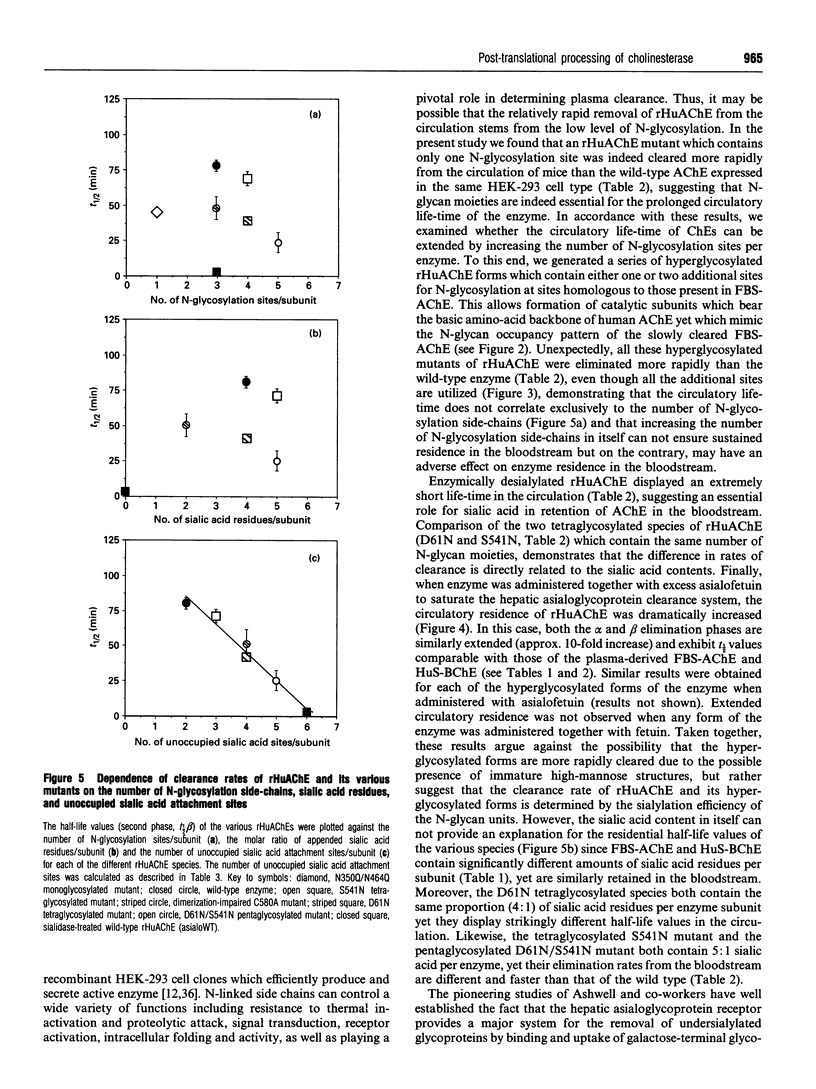
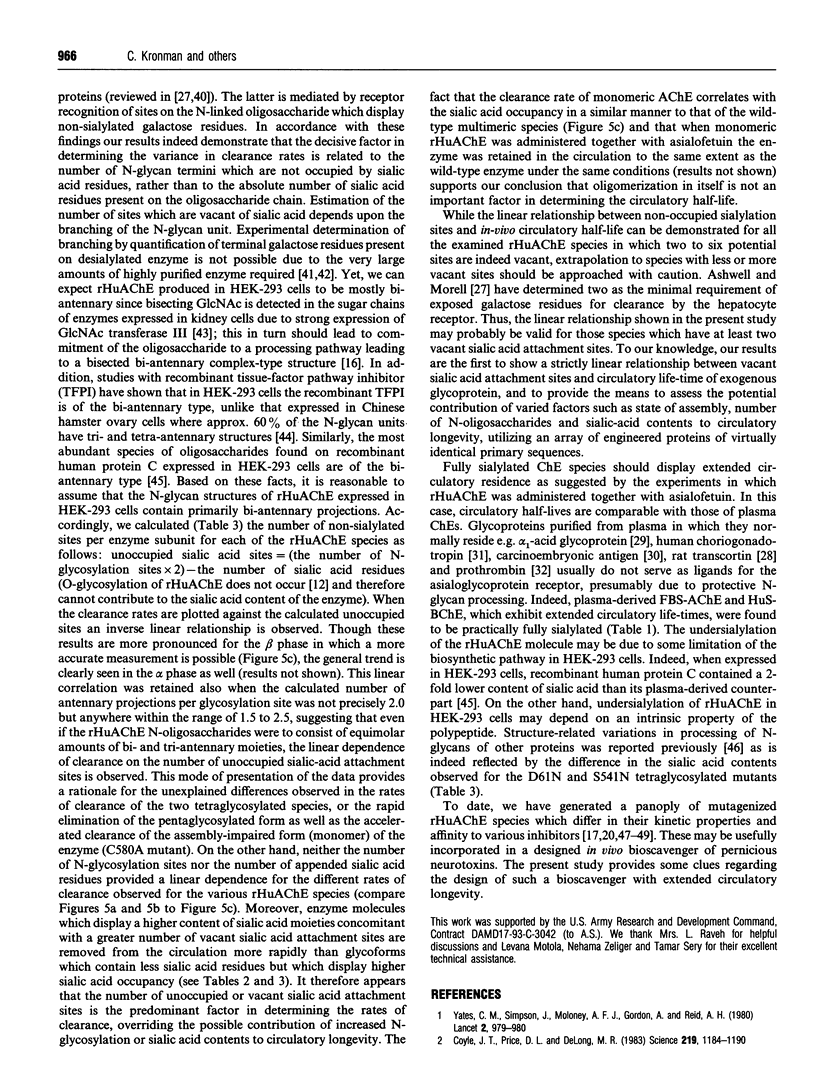
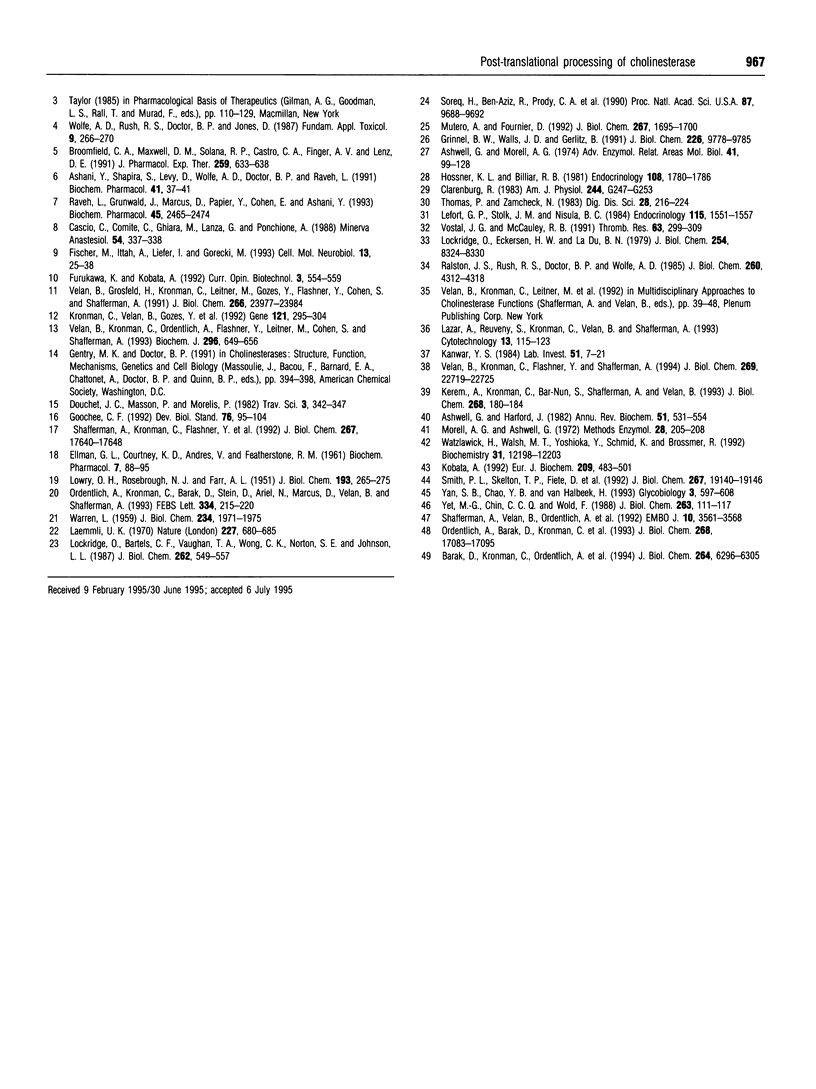
Images in this article
Selected References
These references are in PubMed. This may not be the complete list of references from this article.
- Ashani Y., Shapira S., Levy D., Wolfe A. D., Doctor B. P., Raveh L. Butyrylcholinesterase and acetylcholinesterase prophylaxis against soman poisoning in mice. Biochem Pharmacol. 1991 Jan 1;41(1):37–41. doi: 10.1016/0006-2952(91)90008-s. [DOI] [PubMed] [Google Scholar]
- Ashwell G., Harford J. Carbohydrate-specific receptors of the liver. Annu Rev Biochem. 1982;51:531–554. doi: 10.1146/annurev.bi.51.070182.002531. [DOI] [PubMed] [Google Scholar]
- Barak D., Kronman C., Ordentlich A., Ariel N., Bromberg A., Marcus D., Lazar A., Velan B., Shafferman A. Acetylcholinesterase peripheral anionic site degeneracy conferred by amino acid arrays sharing a common core. J Biol Chem. 1994 Mar 4;269(9):6296–6305. [PubMed] [Google Scholar]
- Broomfield C. A., Maxwell D. M., Solana R. P., Castro C. A., Finger A. V., Lenz D. E. Protection by butyrylcholinesterase against organophosphorus poisoning in nonhuman primates. J Pharmacol Exp Ther. 1991 Nov;259(2):633–638. [PubMed] [Google Scholar]
- Cascio C., Comite C., Ghiara M., Lanza G., Ponchione A. L'uso delle colinesterasi sieriche nella intossicazione grave da organofosforici. Nostra esperienza. Minerva Anestesiol. 1988 Jul-Aug;54(7-8):337–338. [PubMed] [Google Scholar]
- Clarenburg R. Asialoglycoprotein receptor is uninvolved in clearing intact glycoproteins from rat blood. Am J Physiol. 1983 Mar;244(3):G247–G253. doi: 10.1152/ajpgi.1983.244.3.G247. [DOI] [PubMed] [Google Scholar]
- Coyle J. T., Price D. L., DeLong M. R. Alzheimer's disease: a disorder of cortical cholinergic innervation. Science. 1983 Mar 11;219(4589):1184–1190. doi: 10.1126/science.6338589. [DOI] [PubMed] [Google Scholar]
- ELLMAN G. L., COURTNEY K. D., ANDRES V., Jr, FEATHER-STONE R. M. A new and rapid colorimetric determination of acetylcholinesterase activity. Biochem Pharmacol. 1961 Jul;7:88–95. doi: 10.1016/0006-2952(61)90145-9. [DOI] [PubMed] [Google Scholar]
- Fischer M., Ittah A., Liefer I., Gorecki M. Expression and reconstitution of biologically active human acetylcholinesterase from Escherichia coli. Cell Mol Neurobiol. 1993 Feb;13(1):25–38. doi: 10.1007/BF00712987. [DOI] [PubMed] [Google Scholar]
- Furukawa K., Kobata A. Protein glycosylation. Curr Opin Biotechnol. 1992 Oct;3(5):554–559. doi: 10.1016/0958-1669(92)90085-w. [DOI] [PubMed] [Google Scholar]
- Goochee C. F. Bioprocess factors affecting glycoprotein oligosaccharide structure. Dev Biol Stand. 1992;76:95–104. [PubMed] [Google Scholar]
- Grinnell B. W., Walls J. D., Gerlitz B. Glycosylation of human protein C affects its secretion, processing, functional activities, and activation by thrombin. J Biol Chem. 1991 May 25;266(15):9778–9785. [PubMed] [Google Scholar]
- Hossner K. L., Billiar R. B. Plasma clearance and organ distribution of native and desialylated rat and human transcortin: species specificity. Endocrinology. 1981 May;108(5):1780–1786. doi: 10.1210/endo-108-5-1780. [DOI] [PubMed] [Google Scholar]
- Kanwar Y. S. Biophysiology of glomerular filtration and proteinuria. Lab Invest. 1984 Jul;51(1):7–21. [PubMed] [Google Scholar]
- Kerem A., Kronman C., Bar-Nun S., Shafferman A., Velan B. Interrelations between assembly and secretion of recombinant human acetylcholinesterase. J Biol Chem. 1993 Jan 5;268(1):180–184. [PubMed] [Google Scholar]
- Kobata A. Structures and functions of the sugar chains of glycoproteins. Eur J Biochem. 1992 Oct 15;209(2):483–501. doi: 10.1111/j.1432-1033.1992.tb17313.x. [DOI] [PubMed] [Google Scholar]
- Kronman C., Velan B., Gozes Y., Leitner M., Flashner Y., Lazar A., Marcus D., Sery T., Papier Y., Grosfeld H. Production and secretion of high levels of recombinant human acetylcholinesterase in cultured cell lines: microheterogeneity of the catalytic subunit. Gene. 1992 Nov 16;121(2):295–304. doi: 10.1016/0378-1119(92)90134-b. [DOI] [PubMed] [Google Scholar]
- LOWRY O. H., ROSEBROUGH N. J., FARR A. L., RANDALL R. J. Protein measurement with the Folin phenol reagent. J Biol Chem. 1951 Nov;193(1):265–275. [PubMed] [Google Scholar]
- Laemmli U. K. Cleavage of structural proteins during the assembly of the head of bacteriophage T4. Nature. 1970 Aug 15;227(5259):680–685. doi: 10.1038/227680a0. [DOI] [PubMed] [Google Scholar]
- Lazar A., Reuveny S., Kronman C., Velan B., Shafferman A. Evaluation of anchorage-dependent cell propagation systems for production of human acetylcholinesterase by recombinant 293 cells. Cytotechnology. 1993;13(2):115–123. doi: 10.1007/BF00749938. [DOI] [PubMed] [Google Scholar]
- Lefort G. P., Stolk J. M., Nisula B. C. Evidence that desialylation and uptake by hepatic receptors for galactose-terminated glycoproteins are immaterial to the metabolism of human choriogonadotropin in the rat. Endocrinology. 1984 Oct;115(4):1551–1557. doi: 10.1210/endo-115-4-1551. [DOI] [PubMed] [Google Scholar]
- Lockridge O., Bartels C. F., Vaughan T. A., Wong C. K., Norton S. E., Johnson L. L. Complete amino acid sequence of human serum cholinesterase. J Biol Chem. 1987 Jan 15;262(2):549–557. [PubMed] [Google Scholar]
- Lockridge O., Eckerson H. W., La Du B. N. Interchain disulfide bonds and subunit organization in human serum cholinesterase. J Biol Chem. 1979 Sep 10;254(17):8324–8330. [PubMed] [Google Scholar]
- Mutero A., Fournier D. Post-translational modifications of Drosophila acetylcholinesterase. In vitro mutagenesis and expression in Xenopus oocytes. J Biol Chem. 1992 Jan 25;267(3):1695–1700. [PubMed] [Google Scholar]
- Ordentlich A., Barak D., Kronman C., Flashner Y., Leitner M., Segall Y., Ariel N., Cohen S., Velan B., Shafferman A. Dissection of the human acetylcholinesterase active center determinants of substrate specificity. Identification of residues constituting the anionic site, the hydrophobic site, and the acyl pocket. J Biol Chem. 1993 Aug 15;268(23):17083–17095. [PubMed] [Google Scholar]
- Ordentlich A., Kronman C., Barak D., Stein D., Ariel N., Marcus D., Velan B., Shafferman A. Engineering resistance to 'aging' of phosphylated human acetylcholinesterase. Role of hydrogen bond network in the active center. FEBS Lett. 1993 Nov 15;334(2):215–220. doi: 10.1016/0014-5793(93)81714-b. [DOI] [PubMed] [Google Scholar]
- Ralston J. S., Rush R. S., Doctor B. P., Wolfe A. D. Acetylcholinesterase from fetal bovine serum. Purification and characterization of soluble G4 enzyme. J Biol Chem. 1985 Apr 10;260(7):4312–4318. [PubMed] [Google Scholar]
- Raveh L., Grunwald J., Marcus D., Papier Y., Cohen E., Ashani Y. Human butyrylcholinesterase as a general prophylactic antidote for nerve agent toxicity. In vitro and in vivo quantitative characterization. Biochem Pharmacol. 1993 Jun 22;45(12):2465–2474. doi: 10.1016/0006-2952(93)90228-o. [DOI] [PubMed] [Google Scholar]
- Shafferman A., Velan B., Ordentlich A., Kronman C., Grosfeld H., Leitner M., Flashner Y., Cohen S., Barak D., Ariel N. Substrate inhibition of acetylcholinesterase: residues affecting signal transduction from the surface to the catalytic center. EMBO J. 1992 Oct;11(10):3561–3568. doi: 10.1002/j.1460-2075.1992.tb05439.x. [DOI] [PMC free article] [PubMed] [Google Scholar]
- Smith P. L., Skelton T. P., Fiete D., Dharmesh S. M., Beranek M. C., MacPhail L., Broze G. J., Jr, Baenziger J. U. The asparagine-linked oligosaccharides on tissue factor pathway inhibitor terminate with SO4-4GalNAc beta 1, 4GlcNAc beta 1,2 Mana alpha. J Biol Chem. 1992 Sep 25;267(27):19140–19146. [PubMed] [Google Scholar]
- Soreq H., Ben-Aziz R., Prody C. A., Seidman S., Gnatt A., Neville L., Lieman-Hurwitz J., Lev-Lehman E., Ginzberg D., Lipidot-Lifson Y. Molecular cloning and construction of the coding region for human acetylcholinesterase reveals a G + C-rich attenuating structure. Proc Natl Acad Sci U S A. 1990 Dec;87(24):9688–9692. doi: 10.1073/pnas.87.24.9688. [DOI] [PMC free article] [PubMed] [Google Scholar]
- Thomas P., Zamcheck N. Role of the liver in clearance and excretion of circulating carcinoembryonic antigen (CEA). Dig Dis Sci. 1983 Mar;28(3):216–224. doi: 10.1007/BF01295116. [DOI] [PubMed] [Google Scholar]
- Velan B., Grosfeld H., Kronman C., Leitner M., Gozes Y., Lazar A., Flashner Y., Marcus D., Cohen S., Shafferman A. The effect of elimination of intersubunit disulfide bonds on the activity, assembly, and secretion of recombinant human acetylcholinesterase. Expression of acetylcholinesterase Cys-580----Ala mutant. J Biol Chem. 1991 Dec 15;266(35):23977–23984. [PubMed] [Google Scholar]
- Velan B., Kronman C., Flashner Y., Shafferman A. Reversal of signal-mediated cellular retention by subunit assembly of human acetylcholinesterase. J Biol Chem. 1994 Sep 9;269(36):22719–22725. [PubMed] [Google Scholar]
- Velan B., Kronman C., Ordentlich A., Flashner Y., Leitner M., Cohen S., Shafferman A. N-glycosylation of human acetylcholinesterase: effects on activity, stability and biosynthesis. Biochem J. 1993 Dec 15;296(Pt 3):649–656. doi: 10.1042/bj2960649. [DOI] [PMC free article] [PubMed] [Google Scholar]
- Vostal J. G., McCauley R. B. Prothrombin plasma clearance is not mediated by hepatic asialoglycoprotein receptors. Thromb Res. 1991 Aug 1;63(3):299–309. doi: 10.1016/0049-3848(91)90133-h. [DOI] [PubMed] [Google Scholar]
- WARREN L. The thiobarbituric acid assay of sialic acids. J Biol Chem. 1959 Aug;234(8):1971–1975. [PubMed] [Google Scholar]
- Watzlawick H., Walsh M. T., Yoshioka Y., Schmid K., Brossmer R. Structure of the N- and O-glycans of the A-chain of human plasma alpha 2HS-glycoprotein as deduced from the chemical compositions of the derivatives prepared by stepwise degradation with exoglycosidases. Biochemistry. 1992 Dec 8;31(48):12198–12203. doi: 10.1021/bi00163a032. [DOI] [PubMed] [Google Scholar]
- Wolfe A. D., Rush R. S., Doctor B. P., Koplovitz I., Jones D. Acetylcholinesterase prophylaxis against organophosphate toxicity. Fundam Appl Toxicol. 1987 Aug;9(2):266–270. doi: 10.1016/0272-0590(87)90048-0. [DOI] [PubMed] [Google Scholar]
- Yan S. B., Chao Y. B., van Halbeek H. Novel Asn-linked oligosaccharides terminating in GalNAc beta (1-->4)[Fuc alpha (1-->3)]GlcNAc beta (1-->.) are present in recombinant human protein C expressed in human kidney 293 cells. Glycobiology. 1993 Dec;3(6):597–608. doi: 10.1093/glycob/3.6.597. [DOI] [PubMed] [Google Scholar]
- Yates C. M., Simpson J., Maloney A. F., Gordon A., Reid A. H. Alzheimer-like cholinergic deficiency in Down syndrome. Lancet. 1980 Nov 1;2(8201):979–979. doi: 10.1016/s0140-6736(80)92137-6. [DOI] [PubMed] [Google Scholar]
- Yet M. G., Chin C. C., Wold F. The covalent structure of individual N-linked glycopeptides from ovomucoid and asialofetuin. J Biol Chem. 1988 Jan 5;263(1):111–117. [PubMed] [Google Scholar]