Abstract
The nucleotide-binding fold of many NAD(+)-dependent dehydrogenases contains a conserved acidic amino acid residue which hydrogen-bonds with the 2'- and 3'-hydroxy groups of the adenine-ribose of the cofactor. This residue is highly conserved as aspartate in malate dehydrogenases, except in the thermophilic enzyme from Thermus aquaticus B (TaqMDH), which has glutamic acid-41 in the equivalent position. The catalytic mechanism was dissected to investigate the functional significance of this difference in TaqMDH with respect to a mutant enzyme where glutamic acid-41 was replaced by aspartic acid. The mutant enzyme was found to retain a high degree of protein structural stability to both thermal and chemical denaturation. When compared with the wild-type enzyme the mutant had a higher Km and Kd for both reduced and oxidized cofactors (NADH and NAD+) and a 2-3-fold increase in steady-state kcat in both assay directions. The rate-determining step for the reduction of oxaloacetate by wild-type TaqMDH was shown to be the rate of NAD+ release, which was about 2.5-fold higher for the mutant enzyme. This correlates well with the 1.8-fold higher steady-state kcat of the mutant enzyme and represents an improvement in the steady-state kcat of a thermophilic enzyme at moderate temperature by a conservative amino acid substitution which increases the rate of product release.
Full text
PDF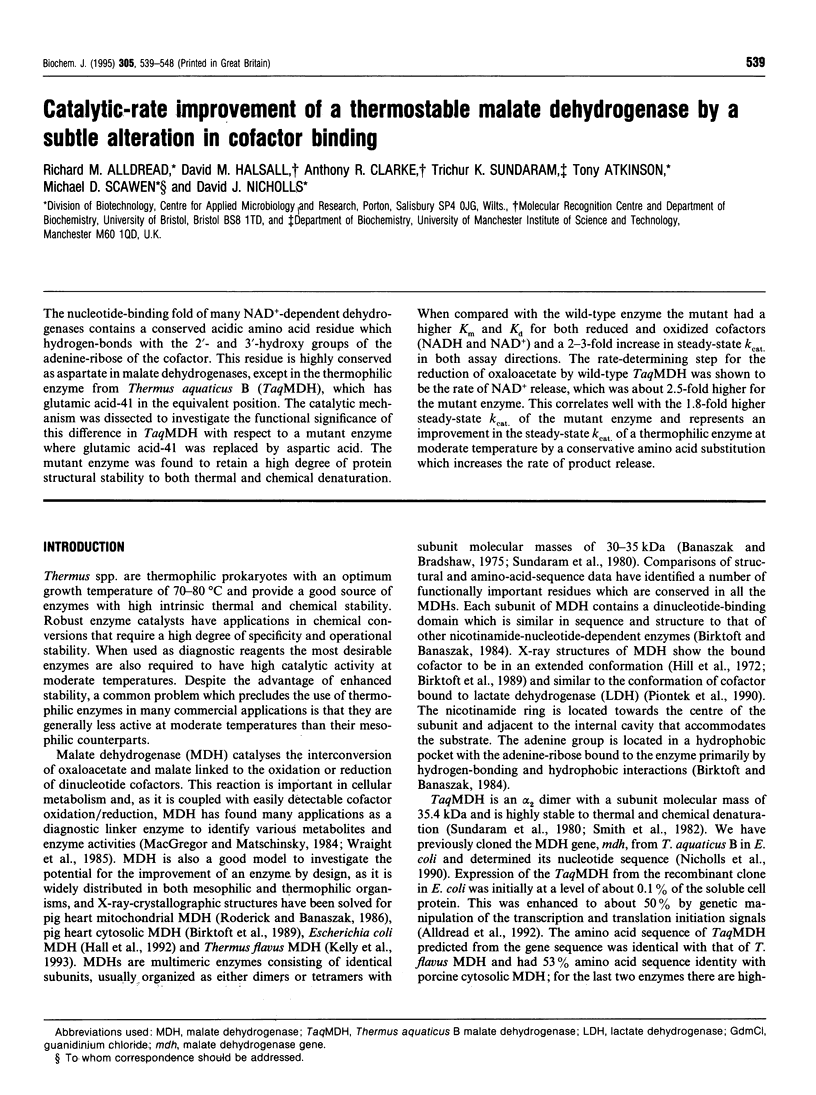
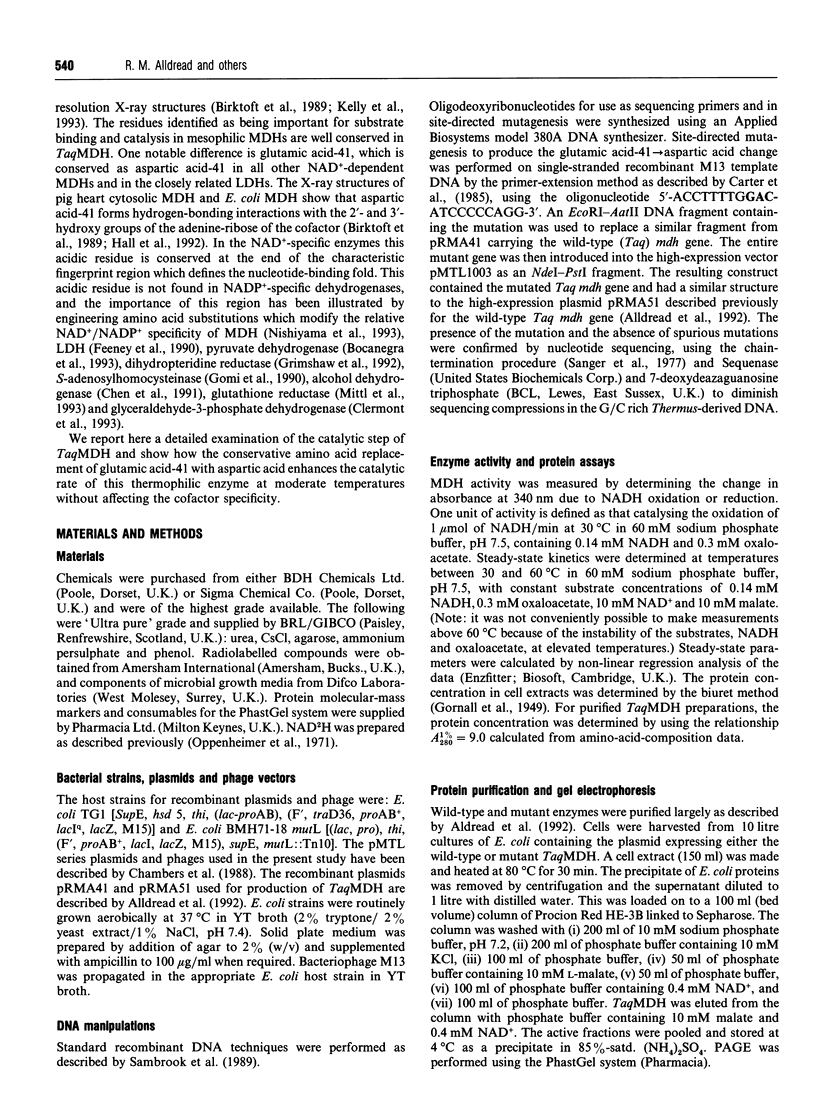
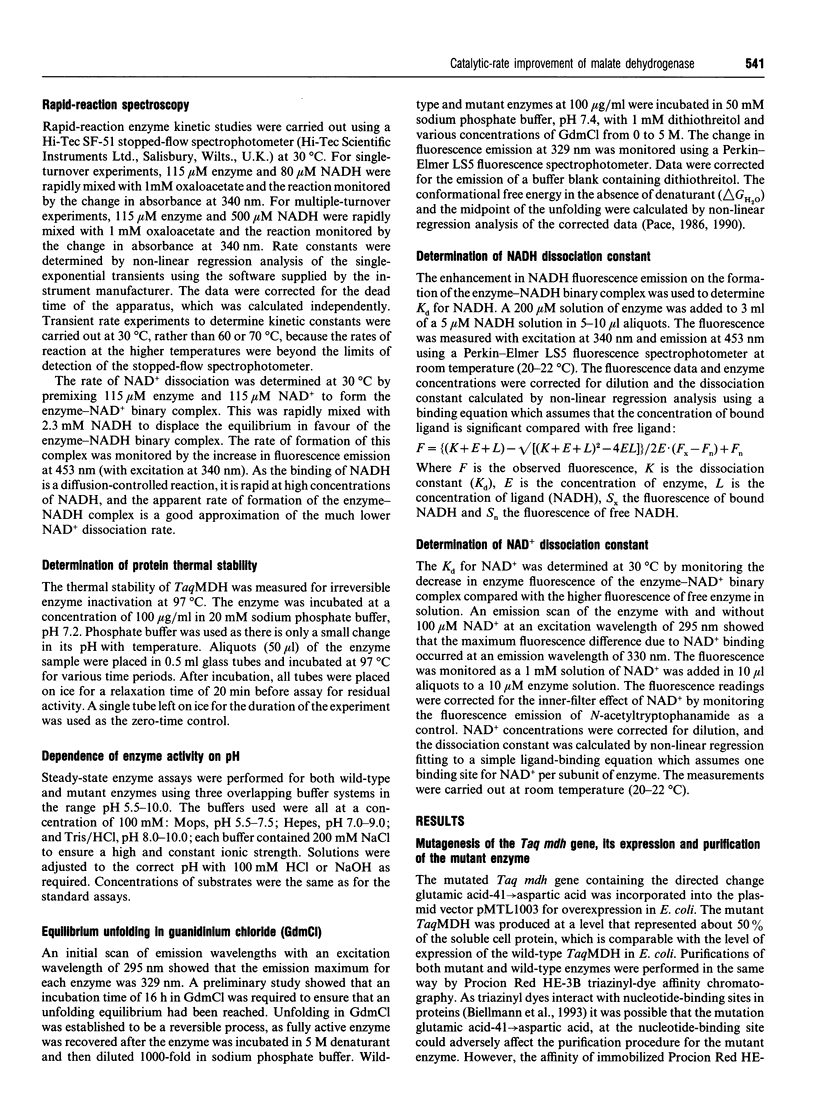
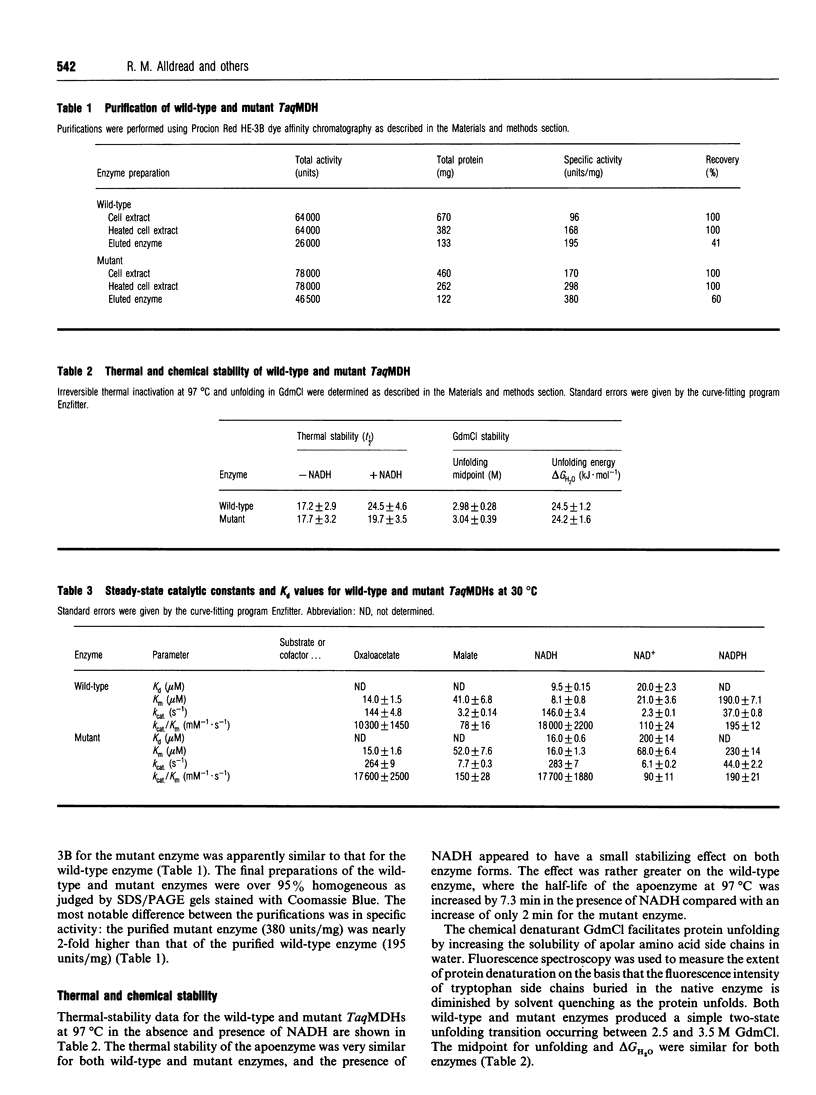
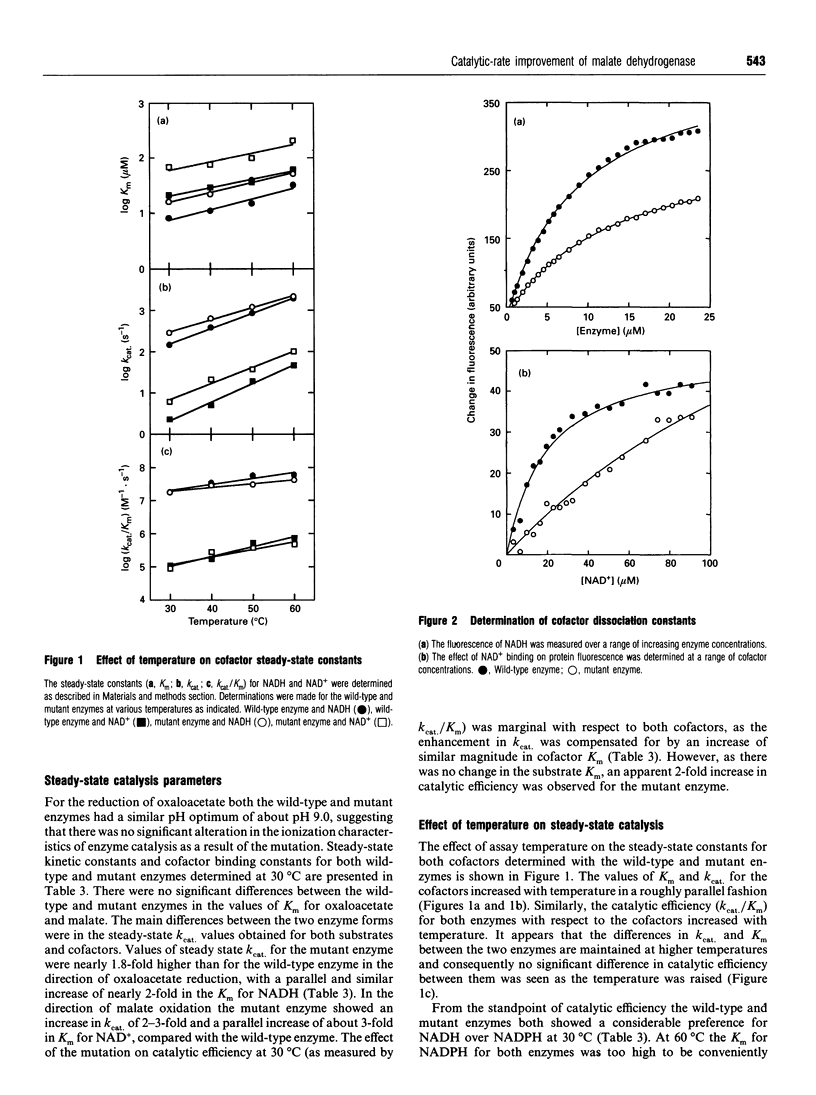
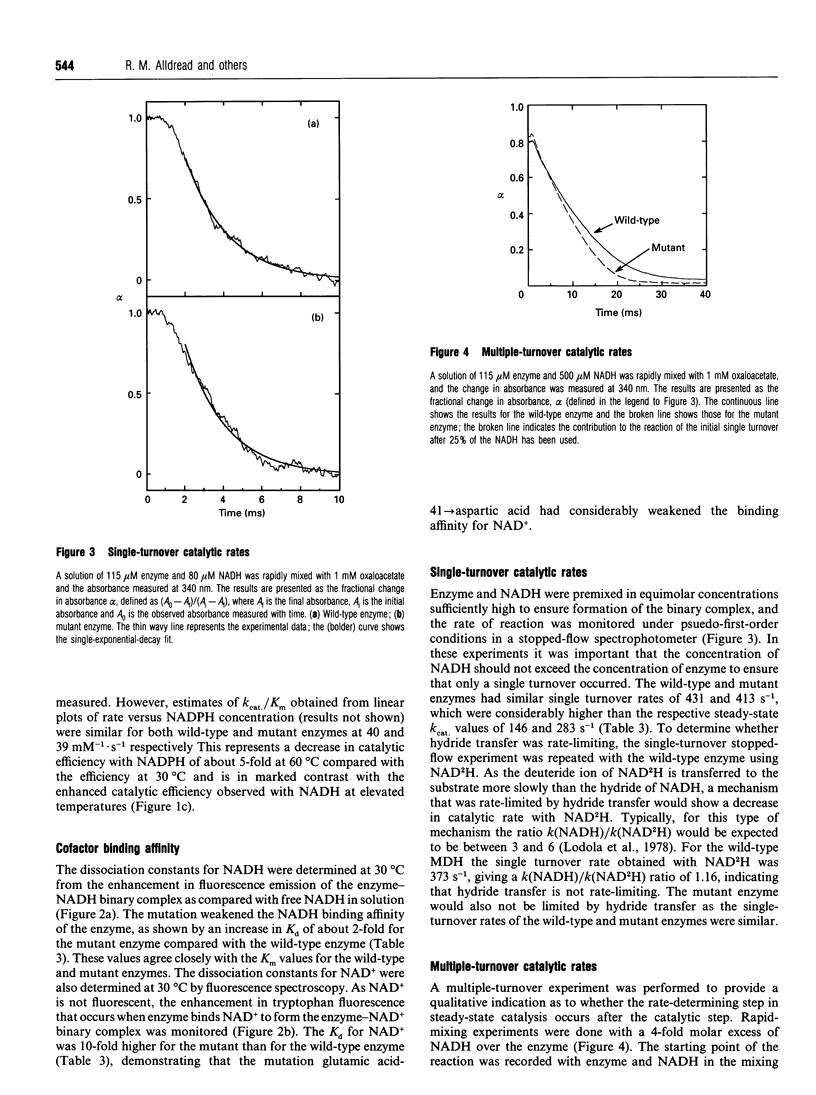
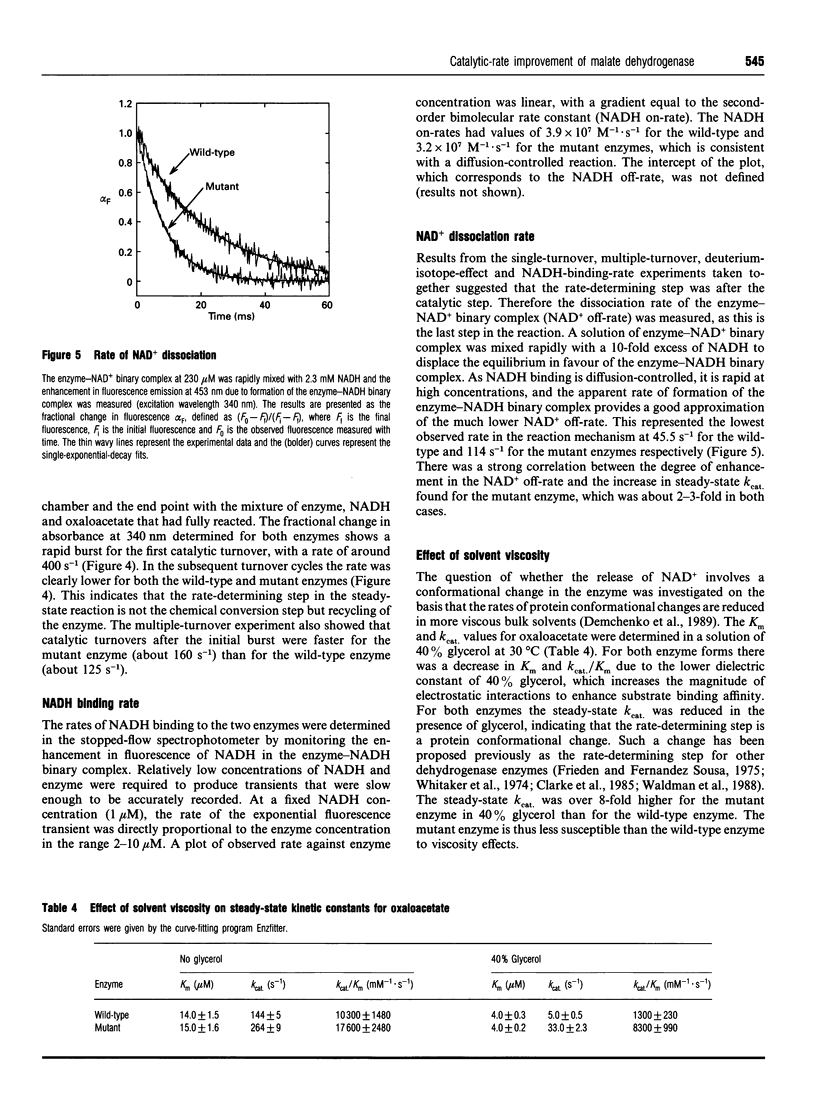
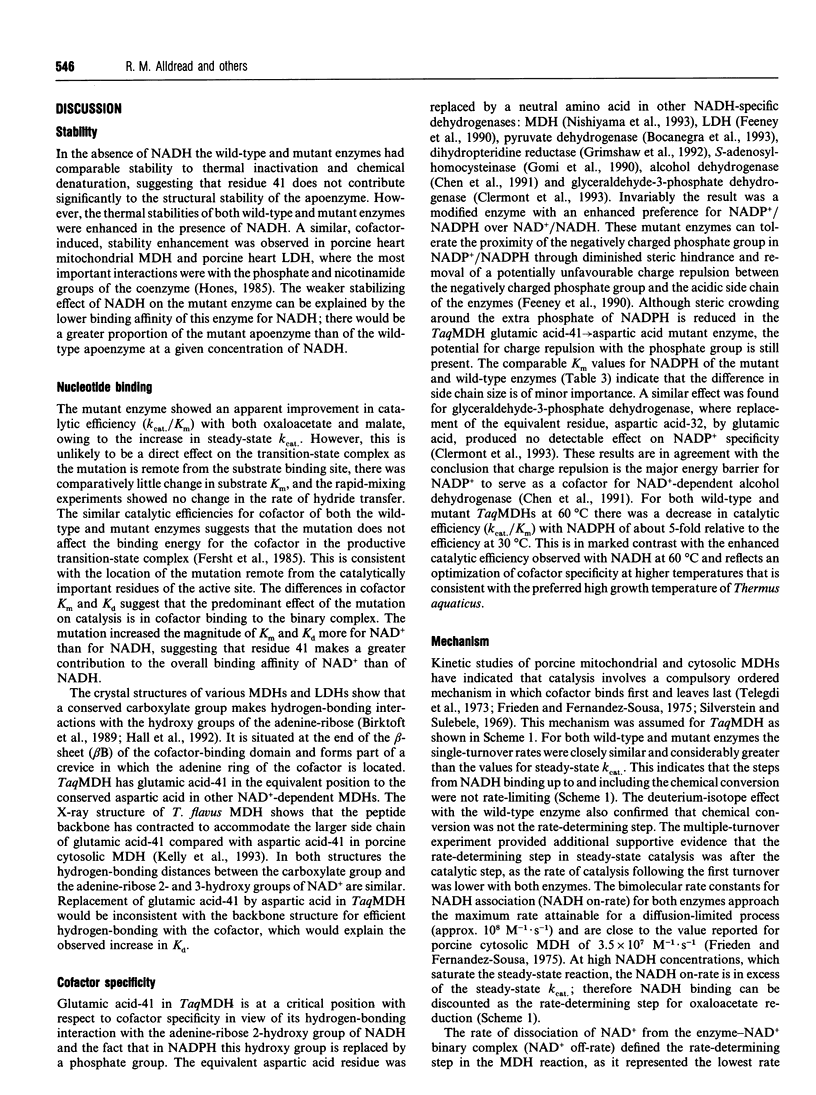
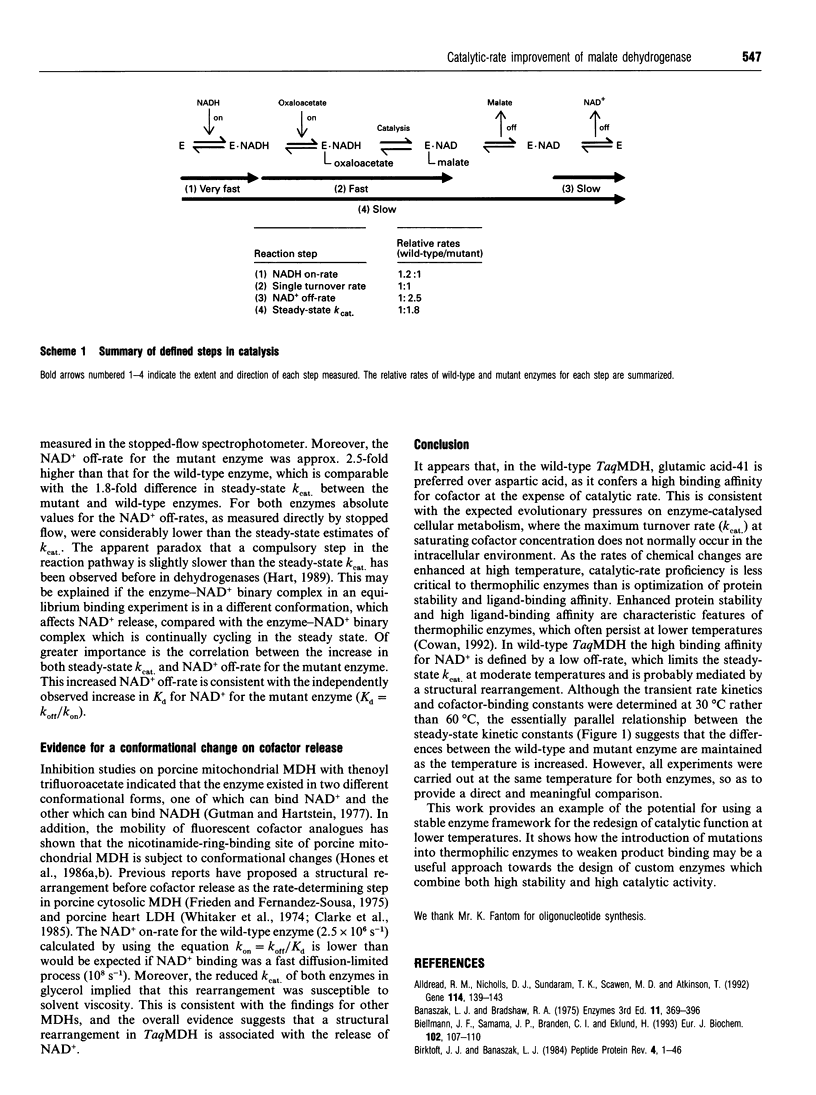
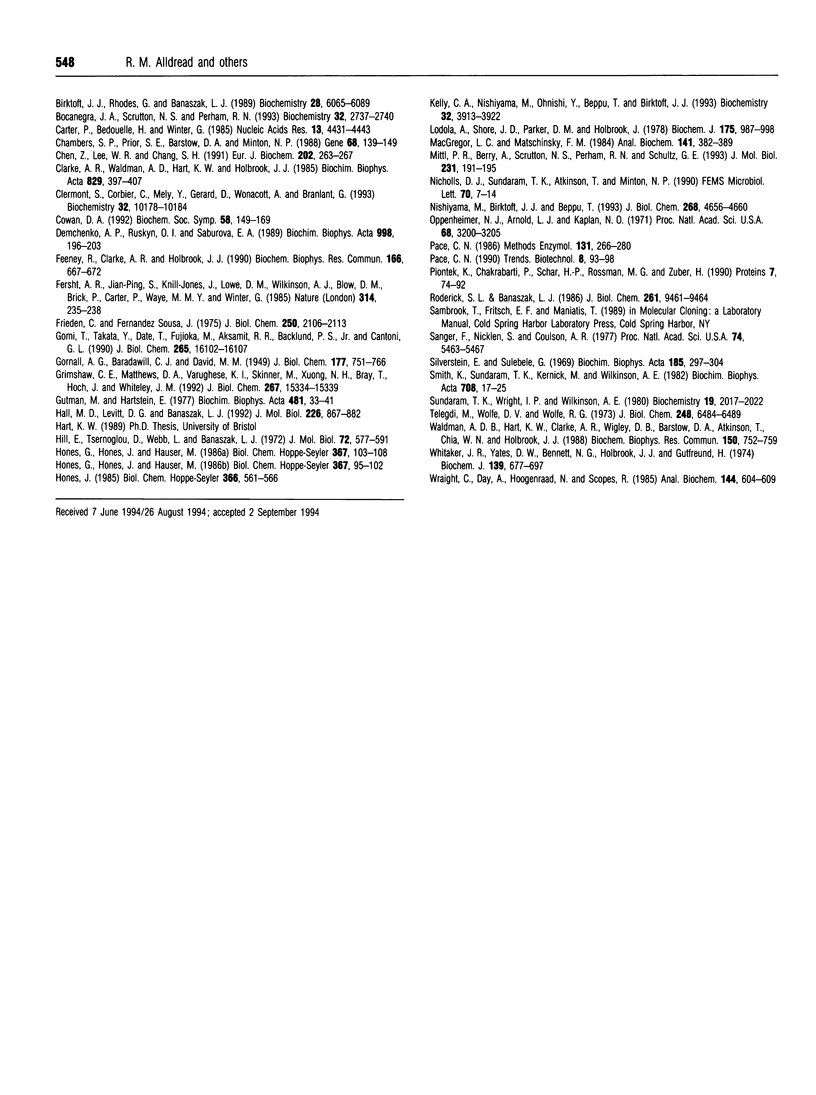
Selected References
These references are in PubMed. This may not be the complete list of references from this article.
- Alldread R. M., Nicholls D. J., Sundaram T. K., Scawen M. D., Atkinson T. Overexpression of the Thermus aquaticus B malate dehydrogenase-encoding gene in Escherichia coli. Gene. 1992 May 1;114(1):139–143. doi: 10.1016/0378-1119(92)90720-a. [DOI] [PubMed] [Google Scholar]
- Biellmann J. F., Samama J. P., Bränden C. I., Eklund H. X-ray studies of the binding of Cibacron blue F3GA to liver alcohol dehydrogenase. Eur J Biochem. 1979 Dec;102(1):107–110. doi: 10.1111/j.1432-1033.1979.tb06268.x. [DOI] [PubMed] [Google Scholar]
- Birktoft J. J., Rhodes G., Banaszak L. J. Refined crystal structure of cytoplasmic malate dehydrogenase at 2.5-A resolution. Biochemistry. 1989 Jul 11;28(14):6065–6081. doi: 10.1021/bi00440a051. [DOI] [PubMed] [Google Scholar]
- Bocanegra J. A., Scrutton N. S., Perham R. N. Creation of an NADP-dependent pyruvate dehydrogenase multienzyme complex by protein engineering. Biochemistry. 1993 Mar 23;32(11):2737–2740. doi: 10.1021/bi00062a001. [DOI] [PubMed] [Google Scholar]
- Carter P., Bedouelle H., Winter G. Improved oligonucleotide site-directed mutagenesis using M13 vectors. Nucleic Acids Res. 1985 Jun 25;13(12):4431–4443. doi: 10.1093/nar/13.12.4431. [DOI] [PMC free article] [PubMed] [Google Scholar]
- Chambers S. P., Prior S. E., Barstow D. A., Minton N. P. The pMTL nic- cloning vectors. I. Improved pUC polylinker regions to facilitate the use of sonicated DNA for nucleotide sequencing. Gene. 1988 Aug 15;68(1):139–149. doi: 10.1016/0378-1119(88)90606-3. [DOI] [PubMed] [Google Scholar]
- Chen Z., Lee W. R., Chang S. H. Role of aspartic acid 38 in the cofactor specificity of Drosophila alcohol dehydrogenase. Eur J Biochem. 1991 Dec 5;202(2):263–267. doi: 10.1111/j.1432-1033.1991.tb16371.x. [DOI] [PubMed] [Google Scholar]
- Clarke A. R., Waldman A. D., Hart K. W., Holbrook J. J. The rates of defined changes in protein structure during the catalytic cycle of lactate dehydrogenase. Biochim Biophys Acta. 1985 Jul 1;829(3):397–407. doi: 10.1016/0167-4838(85)90250-x. [DOI] [PubMed] [Google Scholar]
- Clermont S., Corbier C., Mely Y., Gerard D., Wonacott A., Branlant G. Determinants of coenzyme specificity in glyceraldehyde-3-phosphate dehydrogenase: role of the acidic residue in the fingerprint region of the nucleotide binding fold. Biochemistry. 1993 Sep 28;32(38):10178–10184. doi: 10.1021/bi00089a038. [DOI] [PubMed] [Google Scholar]
- Cowan D. A. Enzymes from thermophilic archaebacteria: current and future applications in biotechnology. Biochem Soc Symp. 1992;58:149–169. [PubMed] [Google Scholar]
- Demchenko A. P., Ruskyn O. I., Saburova E. A. Kinetics of the lactate dehydrogenase reaction in high-viscosity media. Biochim Biophys Acta. 1989 Oct 5;998(2):196–203. doi: 10.1016/0167-4838(89)90273-2. [DOI] [PubMed] [Google Scholar]
- Feeney R., Clarke A. R., Holbrook J. J. A single amino acid substitution in lactate dehydrogenase improves the catalytic efficiency with an alternative coenzyme. Biochem Biophys Res Commun. 1990 Jan 30;166(2):667–672. doi: 10.1016/0006-291x(90)90861-g. [DOI] [PubMed] [Google Scholar]
- Fersht A. R., Shi J. P., Knill-Jones J., Lowe D. M., Wilkinson A. J., Blow D. M., Brick P., Carter P., Waye M. M., Winter G. Hydrogen bonding and biological specificity analysed by protein engineering. Nature. 1985 Mar 21;314(6008):235–238. doi: 10.1038/314235a0. [DOI] [PubMed] [Google Scholar]
- Frieden C., Fernandez-Sousa J. Kinetic studies on pig heart cytoplasmic malate dehydrogenase. J Biol Chem. 1975 Mar 25;250(6):2106–2113. [PubMed] [Google Scholar]
- Gomi T., Takata Y., Date T., Fujioka M., Aksamit R. R., Backlund P. S., Jr, Cantoni G. L. Site-directed mutagenesis of rat liver S-adenosylhomocysteinase. Effect of conversion of aspartic acid 244 to glutamic acid on coenzyme binding. J Biol Chem. 1990 Sep 25;265(27):16102–16107. [PubMed] [Google Scholar]
- Grimshaw C. E., Matthews D. A., Varughese K. I., Skinner M., Xuong N. H., Bray T., Hoch J., Whiteley J. M. Characterization and nucleotide binding properties of a mutant dihydropteridine reductase containing an aspartate 37-isoleucine replacement. J Biol Chem. 1992 Aug 5;267(22):15334–15339. [PubMed] [Google Scholar]
- Gutman M., Hartstein E. Distinction between NAD- and NADH-binding forms of mitochondrial malate dehydrogenase as shown by inhibition with thenoyltrifuoroacetone. Biochim Biophys Acta. 1977 Mar 15;481(1):33–41. doi: 10.1016/0005-2744(77)90134-6. [DOI] [PubMed] [Google Scholar]
- Hall M. D., Levitt D. G., Banaszak L. J. Crystal structure of Escherichia coli malate dehydrogenase. A complex of the apoenzyme and citrate at 1.87 A resolution. J Mol Biol. 1992 Aug 5;226(3):867–882. doi: 10.1016/0022-2836(92)90637-y. [DOI] [PubMed] [Google Scholar]
- Hill E., Tsernoglou D., Webb L., Banaszak L. J. Polypeptide conformation of cytoplasmic malate dehydrogenase from an electron density map at 3.0 angstrom resolution. J Mol Biol. 1972 Dec 30;72(3):577–589. doi: 10.1016/0022-2836(72)90176-3. [DOI] [PubMed] [Google Scholar]
- Hönes G., Hönes J., Hauser M. Studies of enzyme-ligand complexes using dynamic fluorescence anisotropy. I. The substrate-binding site of malate dehydrogenase. Biol Chem Hoppe Seyler. 1986 Feb;367(2):95–102. doi: 10.1515/bchm3.1986.367.1.95. [DOI] [PubMed] [Google Scholar]
- Hönes G., Hönes J., Hauser M. Studies of enzyme-ligand complexes using dynamic fluorescence anisotropy. II. The coenzyme-binding site of malate dehydrogenase. Biol Chem Hoppe Seyler. 1986 Feb;367(2):103–108. doi: 10.1515/bchm3.1986.367.1.103. [DOI] [PubMed] [Google Scholar]
- Hönes J. Ligand binding and stabilization of malate- and lactate dehydrogenase. Biol Chem Hoppe Seyler. 1985 Jun;366(6):561–566. doi: 10.1515/bchm3.1985.366.1.561. [DOI] [PubMed] [Google Scholar]
- Kelly C. A., Nishiyama M., Ohnishi Y., Beppu T., Birktoft J. J. Determinants of protein thermostability observed in the 1.9-A crystal structure of malate dehydrogenase from the thermophilic bacterium Thermus flavus. Biochemistry. 1993 Apr 20;32(15):3913–3922. doi: 10.1021/bi00066a010. [DOI] [PubMed] [Google Scholar]
- Lodola A., Shore J. D., Parker D. M., Holbrook J. Malate dehydrogenase of the cytosol. A kinetic investigation of the reaction mechanism and a comparison with lactate dehydrogenase. Biochem J. 1978 Dec 1;175(3):987–998. doi: 10.1042/bj1750987. [DOI] [PMC free article] [PubMed] [Google Scholar]
- MacGregor L. C., Matschinsky F. M. An enzymatic fluorimetric assay for myo-inositol. Anal Biochem. 1984 Sep;141(2):382–389. doi: 10.1016/0003-2697(84)90058-7. [DOI] [PubMed] [Google Scholar]
- Mittl P. R., Berry A., Scrutton N. S., Perham R. N., Schulz G. E. Structural differences between wild-type NADP-dependent glutathione reductase from Escherichia coli and a redesigned NAD-dependent mutant. J Mol Biol. 1993 May 20;231(2):191–195. doi: 10.1006/jmbi.1993.1274. [DOI] [PubMed] [Google Scholar]
- Nicholls D. J., Sundaram T. K., Atkinson T., Minton N. P. Cloning and nucleotide sequences of the mdh and sucD genes from Thermus aquaticus B. FEMS Microbiol Lett. 1990 Jun 15;58(1):7–14. doi: 10.1016/0378-1097(90)90093-6. [DOI] [PubMed] [Google Scholar]
- Nishiyama M., Birktoft J. J., Beppu T. Alteration of coenzyme specificity of malate dehydrogenase from Thermus flavus by site-directed mutagenesis. J Biol Chem. 1993 Mar 5;268(7):4656–4660. [PubMed] [Google Scholar]
- Oppenheimer N. J., Arnold L. J., Kaplan N. O. A structure of pyridine nucleotides in solution. Proc Natl Acad Sci U S A. 1971 Dec;68(12):3200–3205. doi: 10.1073/pnas.68.12.3200. [DOI] [PMC free article] [PubMed] [Google Scholar]
- Pace C. N. Determination and analysis of urea and guanidine hydrochloride denaturation curves. Methods Enzymol. 1986;131:266–280. doi: 10.1016/0076-6879(86)31045-0. [DOI] [PubMed] [Google Scholar]
- Pace C. N. Measuring and increasing protein stability. Trends Biotechnol. 1990 Apr;8(4):93–98. doi: 10.1016/0167-7799(90)90146-o. [DOI] [PubMed] [Google Scholar]
- Piontek K., Chakrabarti P., Schär H. P., Rossmann M. G., Zuber H. Structure determination and refinement of Bacillus stearothermophilus lactate dehydrogenase. Proteins. 1990;7(1):74–92. doi: 10.1002/prot.340070108. [DOI] [PubMed] [Google Scholar]
- Roderick S. L., Banaszak L. J. The three-dimensional structure of porcine heart mitochondrial malate dehydrogenase at 3.0-A resolution. J Biol Chem. 1986 Jul 15;261(20):9461–9464. [PubMed] [Google Scholar]
- Sanger F., Nicklen S., Coulson A. R. DNA sequencing with chain-terminating inhibitors. Proc Natl Acad Sci U S A. 1977 Dec;74(12):5463–5467. doi: 10.1073/pnas.74.12.5463. [DOI] [PMC free article] [PubMed] [Google Scholar]
- Silverstein E., Sulebele G. Equilibrium kinetic study of the mechanism of mitochondrial and supernatant malate dehydrogenases of bovine heart. Biochim Biophys Acta. 1969;185(2):297–304. doi: 10.1016/0005-2744(69)90422-7. [DOI] [PubMed] [Google Scholar]
- Sundaram T. K., Wright I. P., Wilkinson A. E. Malate dehydrogenase from thermophilic and mesophilic bacteria. Molecular size, subunit structure, amino acid composition, immunochemical homology, and catalytic activity. Biochemistry. 1980 May 13;19(10):2017–2022. doi: 10.1021/bi00551a002. [DOI] [PubMed] [Google Scholar]
- Telegdi M., Wolfe D. V., Wolfe R. G. Malate dehydrogenase. XII. Initial rate kinetic studies of substrate activation of porcine mitochondrial enzyme by malate. J Biol Chem. 1973 Sep 25;248(18):6484–6489. [PubMed] [Google Scholar]
- Waldman A. D., Hart K. W., Clarke A. R., Wigley D. B., Barstow D. A., Atkinson T., Chia W. N., Holbrook J. J. The use of genetically engineered tryptophan to identify the movement of a domain of B. stearothermophilus lactate dehydrogenase with the process which limits the steady-state turnover of the enzyme. Biochem Biophys Res Commun. 1988 Jan 29;150(2):752–759. doi: 10.1016/0006-291x(88)90455-x. [DOI] [PubMed] [Google Scholar]
- Whitaker J. R., Yates D. W., Bennett N. G., Holbrook J. J., Gutfreund H. The identification of intermediates in the reaction of pig heart lactate dehydrogenase with its substrates. Biochem J. 1974 Jun;139(3):677–697. doi: 10.1042/bj1390677. [DOI] [PMC free article] [PubMed] [Google Scholar]
- Wraight C., Day A., Hoogenraad N., Scopes R. A two-step purification of ATP-citrate lyase from rat liver and its use in a fluorometric assay for N-acetylglutamate synthetase. Anal Biochem. 1985 Feb 1;144(2):604–609. doi: 10.1016/0003-2697(85)90159-9. [DOI] [PubMed] [Google Scholar]