Abstract
Rifamycin SV is an antibiotic anti-bacterial agent used in the treatment of tuberculosis. This drug can autoxidize, especially in the presence of metals, and generate reactive oxygen species. A previous study indicated that rifamycin SV can increase NADH-dependent microsomal production of reactive oxygen species. The current study evaluated the ability of rifamycin SV to interact with iron and increase microsomal production of hydroxyl radical, as detected by conversion of supercoiled plasmid DNA into the relaxed open circular state. The plasmid used was pBluescript II KS(-), and the forms of DNA were separated by agarose-gel electrophoresis. Incubation of rat liver microsomes with plasmid plus NADH plus ferric-ATP caused DNA strand cleavage. The addition of rifamycin SV produced a time- and concentration-dependent increase in DNA-strand cleavage. No stimulation by rifamycin SV occurred in the absence of microsomes, NADH or ferric-ATP. Stimulation occurred with other ferric complexes besides ferric-ATP, e.g. ferric-histidine, ferric-citrate, ferric-EDTA, and ferric-(NH4)2SO4. Rifamycin SV did not significantly increase the high rates of DNA strand cleavage found with NADPH as the microsomal reductant. The stimulation of NADH-dependent microsomal DNA strand cleavage was completely blocked by catalase, superoxide dismutase, GSH and a variety of hydroxyl-radical-scavenging agents, but not by anti-oxidants that prevent microsomal lipid peroxidation. Redox cycling agents, such as menadione and paraquat, in contrast with rifamycin SV, stimulated the NADPH-dependent reaction; menadione and rifamycin SV were superior to paraquat in stimulating the NADH-dependent reaction. These results indicate that rifamycin SV can, in the presence of an iron catalyst, increase microsomal production of reactive oxygen species which can cause DNA-strand cleavage. In contrast with other redox cycling agents, the stimulation by rifamycin SV is more pronounced with NADH than with NADPH as the microsomal reductant. Interactions between rifamycin SV, iron and NADH generating hydroxyl-radical-like species may play a role in some of the hepatotoxic effects associated with the use of this antibacterial antibiotic.
Full text
PDF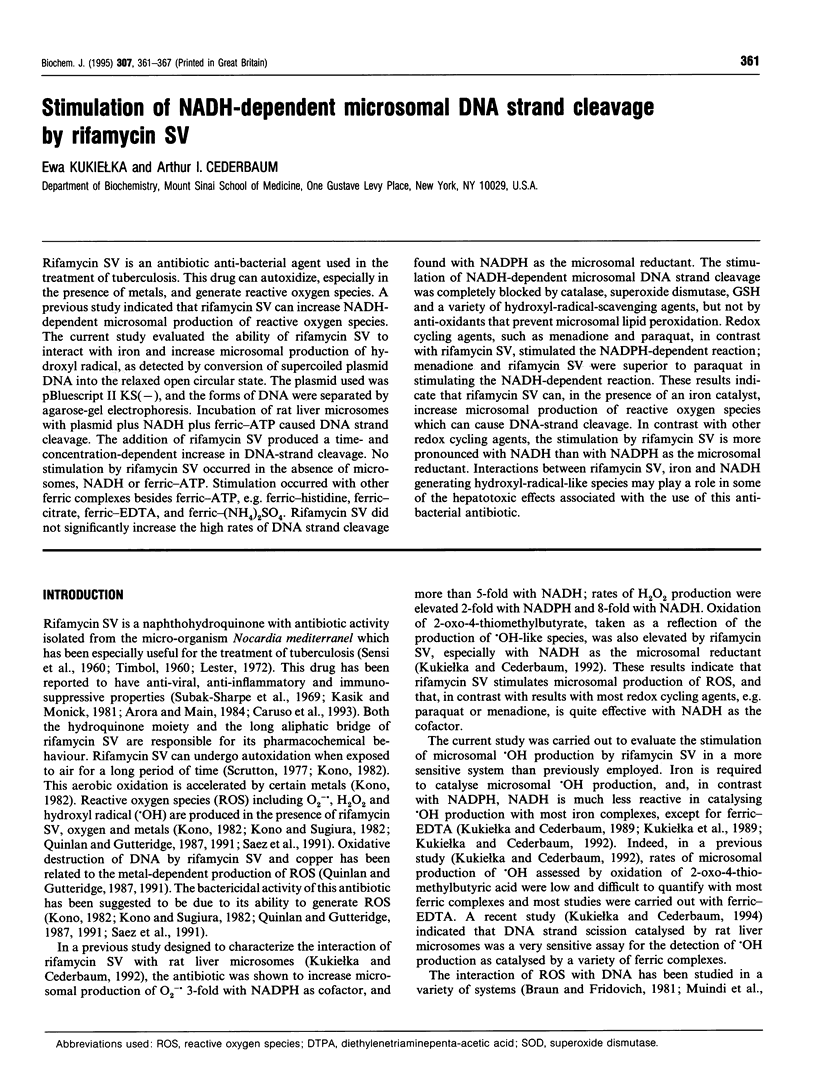
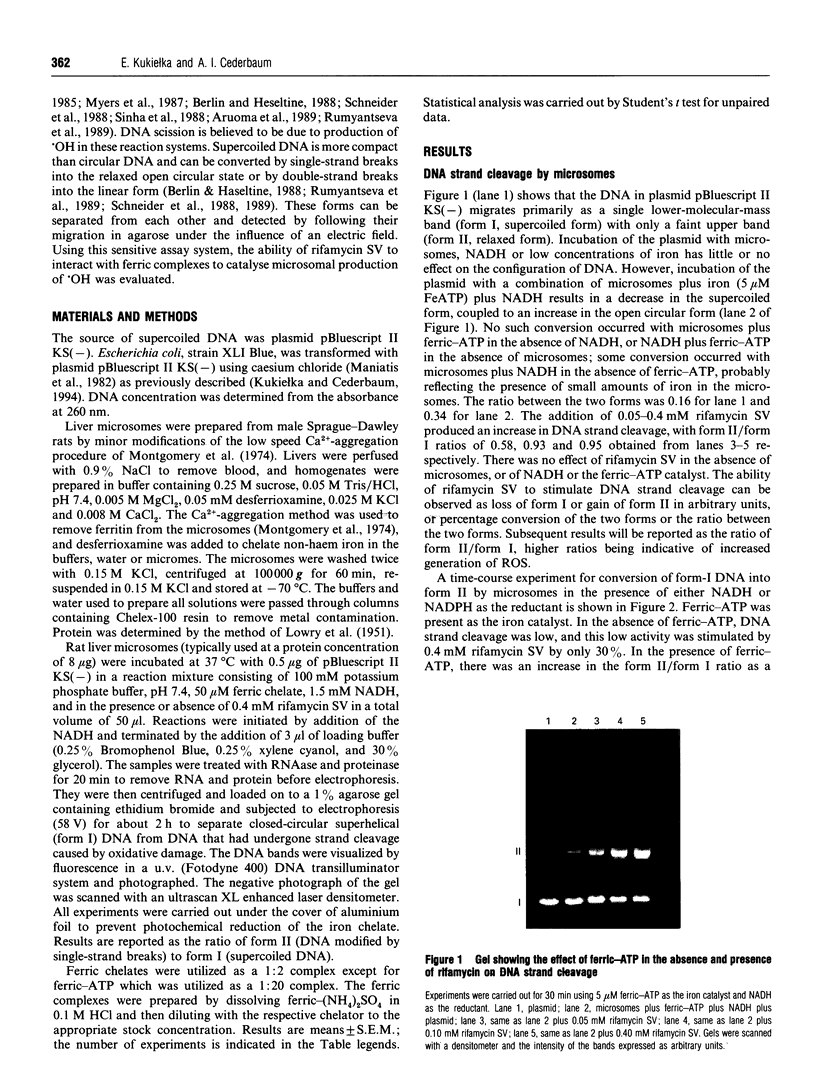
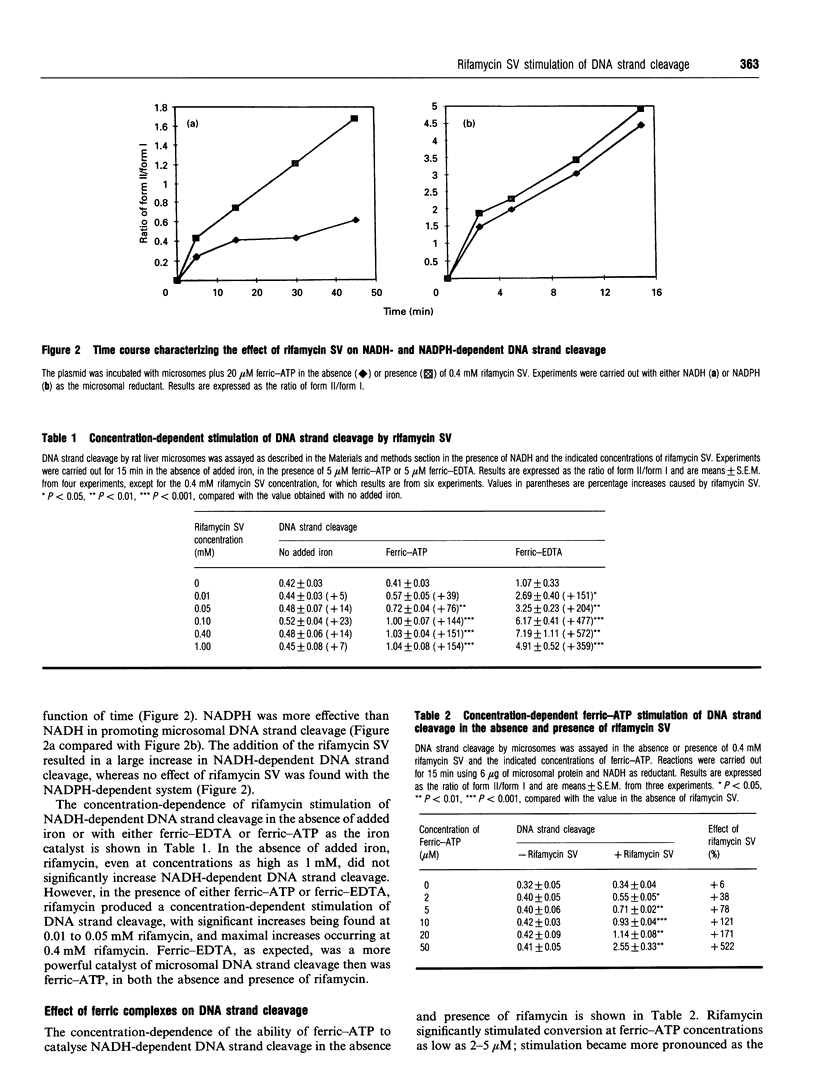
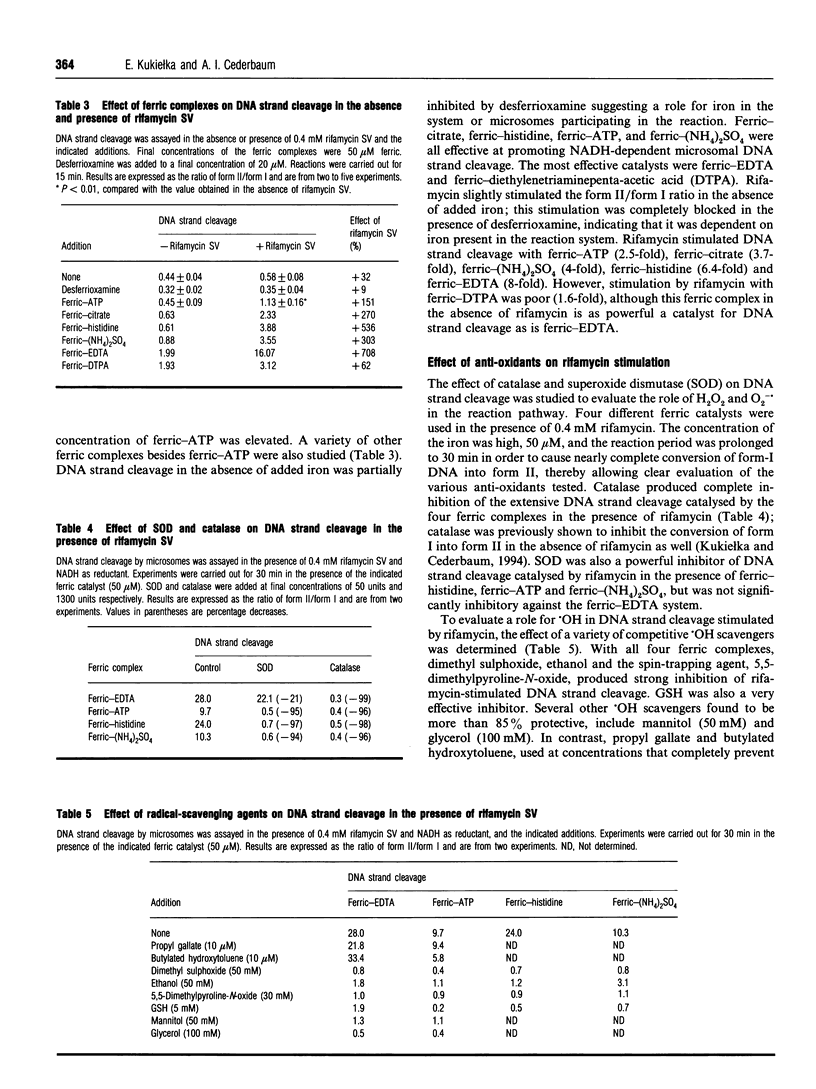
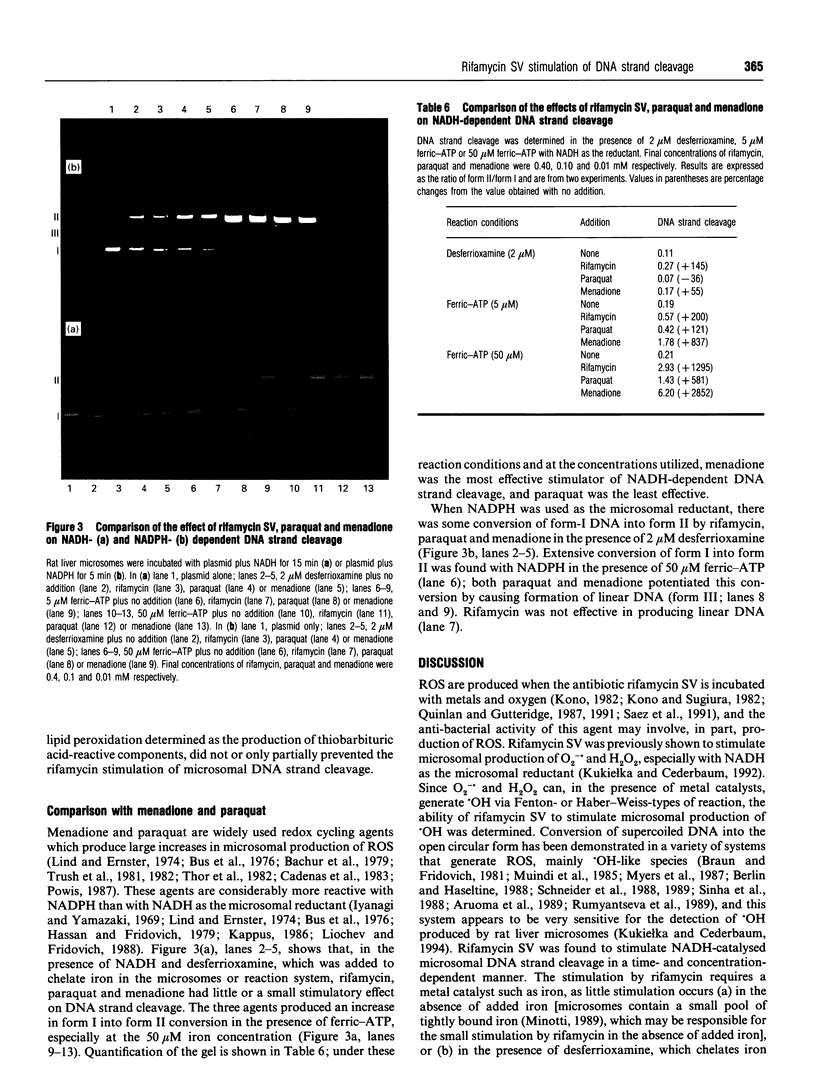
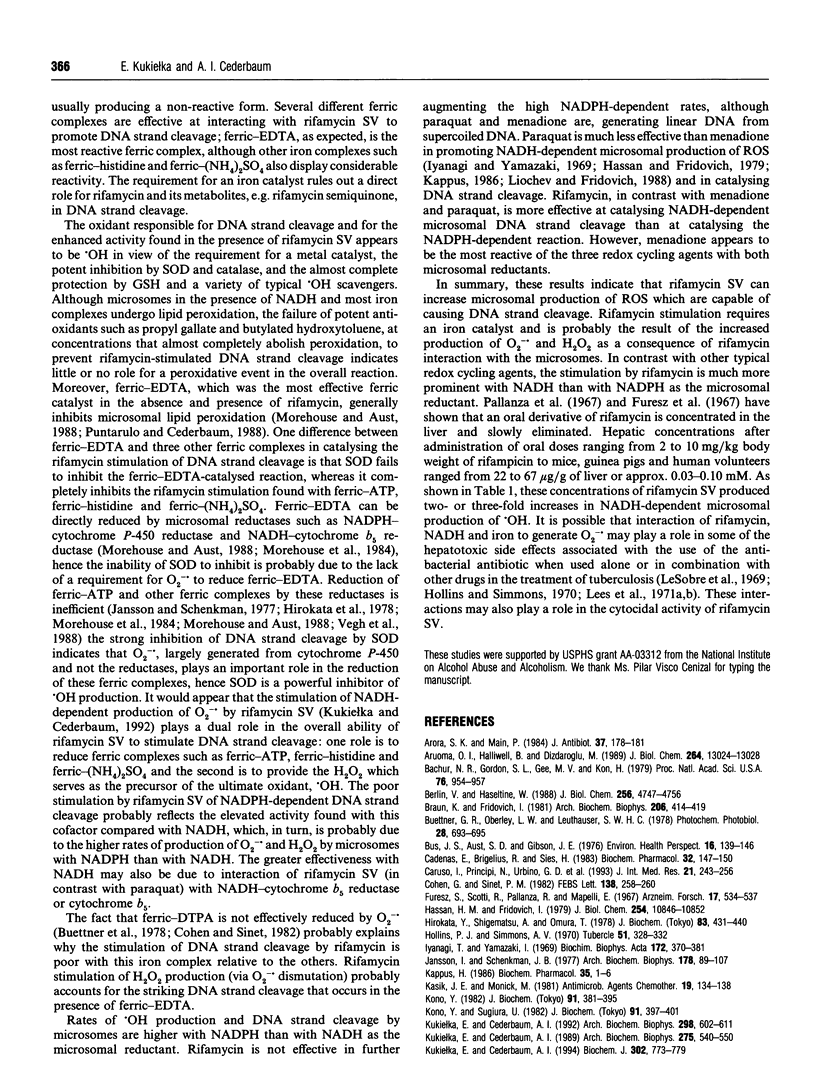
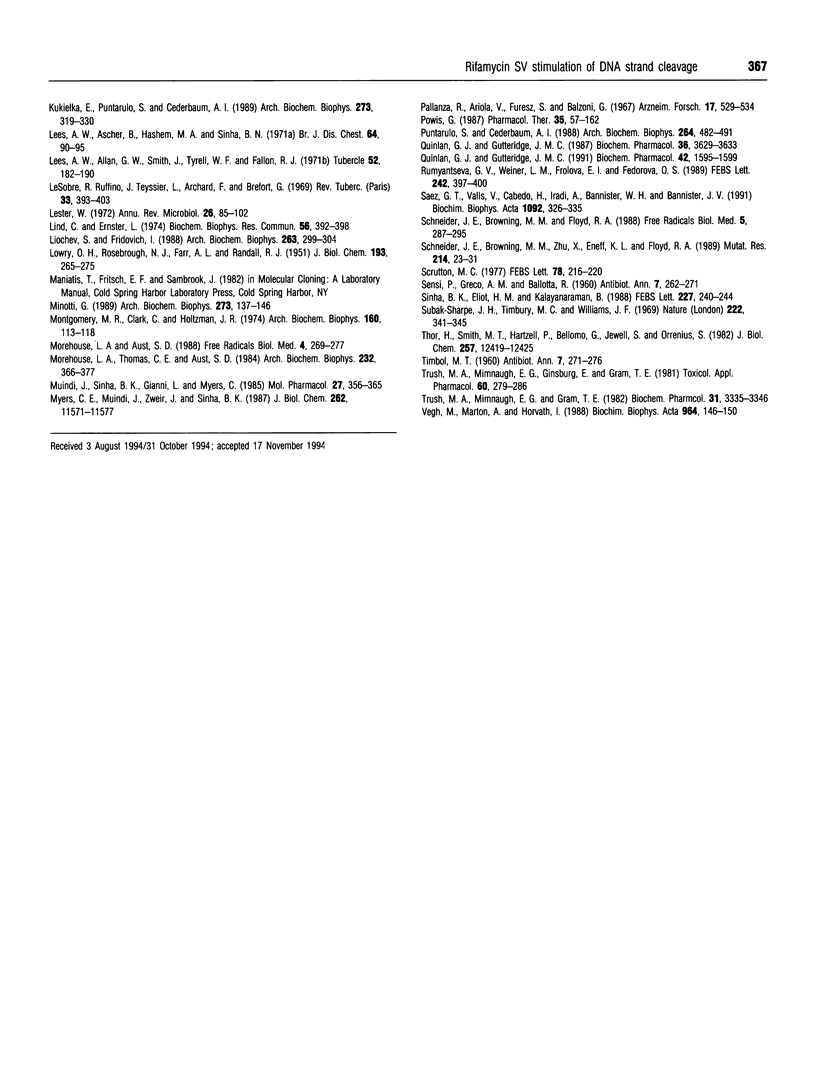
Images in this article
Selected References
These references are in PubMed. This may not be the complete list of references from this article.
- Arora S. K., Main P. Correlation of structure and activity in ansamycins: molecular structure of cyclized rifamycin SV. J Antibiot (Tokyo) 1984 Feb;37(2):178–181. doi: 10.7164/antibiotics.37.178. [DOI] [PubMed] [Google Scholar]
- Aruoma O. I., Halliwell B., Dizdaroglu M. Iron ion-dependent modification of bases in DNA by the superoxide radical-generating system hypoxanthine/xanthine oxidase. J Biol Chem. 1989 Aug 5;264(22):13024–13028. [PubMed] [Google Scholar]
- Bachur N. R., Gordon S. L., Gee M. V., Kon H. NADPH cytochrome P-450 reductase activation of quinone anticancer agents to free radicals. Proc Natl Acad Sci U S A. 1979 Feb;76(2):954–957. doi: 10.1073/pnas.76.2.954. [DOI] [PMC free article] [PubMed] [Google Scholar]
- Berlin V., Haseltine W. A. Reduction of adriamycin to a semiquinone-free radical by NADPH cytochrome P-450 reductase produces DNA cleavage in a reaction mediated by molecular oxygen. J Biol Chem. 1981 May 25;256(10):4747–4756. [PubMed] [Google Scholar]
- Brawn K., Fridovich I. DNA strand scission by enzymically generated oxygen radicals. Arch Biochem Biophys. 1981 Feb;206(2):414–419. doi: 10.1016/0003-9861(81)90108-9. [DOI] [PubMed] [Google Scholar]
- Buettner G. R., Oberley L. W., Leuthauser S. W. The effect of iron on the distribution of superoxide and hydroxyl radicals as seen by spin trapping and on the superoxide dismutase assay. Photochem Photobiol. 1978 Oct-Nov;28(4-5):693–695. doi: 10.1111/j.1751-1097.1978.tb07001.x. [DOI] [PubMed] [Google Scholar]
- Bus J. S., Aust S. D., Gibson J. E. Paraquat toxicity: proposed mechanism of action involving lipid peroxidation. Environ Health Perspect. 1976 Aug;16:139–146. doi: 10.1289/ehp.7616139. [DOI] [PMC free article] [PubMed] [Google Scholar]
- Cadenas E., Brigelius R., Sies H. Paraquat-induced chemiluminescence of microsomal fractions. Biochem Pharmacol. 1983 Jan 1;32(1):147–150. doi: 10.1016/0006-2952(83)90667-6. [DOI] [PubMed] [Google Scholar]
- Caruso I., Principi N., D'Urbino G., Santandrea S., Boccassini L., Montrone F., Sarzi Puttini P. C., Bombaci A., Bozzato A., Azzolini V. Rifamycin SV administered by intra-articular infiltrations shows disease modifying activity in patients with pauci- or polyarticular juvenile rheumatoid arthritis. J Int Med Res. 1993 Sep-Oct;21(5):243–256. doi: 10.1177/030006059302100503. [DOI] [PubMed] [Google Scholar]
- Furesz S., Scotti R., Pallanza R., Mapelli E. Rifampicin: a new rifamycin. 3. Absorption, distribution, and elimination in man. Arzneimittelforschung. 1967 May;17(5):534–537. [PubMed] [Google Scholar]
- Hassan H. M., Fridovich I. Paraquat and Escherichia coli. Mechanism of production of extracellular superoxide radical. J Biol Chem. 1979 Nov 10;254(21):10846–10852. [PubMed] [Google Scholar]
- Hirokata Y., Shigematsu A., Omura T. Immunochemical study on the pathway of electron flow in reduced nicotinamide adenine dinucleotide-dependent microsomal lipid peroxidation. J Biochem. 1978 Feb;83(2):431–440. doi: 10.1093/oxfordjournals.jbchem.a131930. [DOI] [PubMed] [Google Scholar]
- Hollins P. J., Simmons A. V. Jaundice associated with rifampicin. Tubercle. 1970 Sep;51(3):328–332. doi: 10.1016/0041-3879(70)90029-2. [DOI] [PubMed] [Google Scholar]
- Iyanagi T., Yamazaki I. One-electron-transfer reactions in biochemical systems. 3. One-electron reduction of quinones by microsomal flavin enzymes. Biochim Biophys Acta. 1969 Apr 8;172(3):370–381. doi: 10.1016/0005-2728(69)90133-9. [DOI] [PubMed] [Google Scholar]
- Jansson I., Schenkman J. B. Studies on three microsomal electron transfer enzyme systems. Specificity of electron flow pathways. Arch Biochem Biophys. 1977 Jan 15;178(1):89–107. doi: 10.1016/0003-9861(77)90174-6. [DOI] [PubMed] [Google Scholar]
- Kappus H. Overview of enzyme systems involved in bio-reduction of drugs and in redox cycling. Biochem Pharmacol. 1986 Jan 1;35(1):1–6. doi: 10.1016/0006-2952(86)90544-7. [DOI] [PubMed] [Google Scholar]
- Kasik J. E., Monick M. Comparison of antibacterial and antiimmune effects of certain rifamycins. Antimicrob Agents Chemother. 1981 Jan;19(1):134–138. doi: 10.1128/aac.19.1.134. [DOI] [PMC free article] [PubMed] [Google Scholar]
- Kono Y. Oxygen Enhancement of bactericidal activity of rifamycin SV on Escherichia coli and aerobic oxidation of rifamycin SV to rifamycin S catalyzed by manganous ions: the role of superoxide. J Biochem. 1982 Jan;91(1):381–395. doi: 10.1093/oxfordjournals.jbchem.a133698. [DOI] [PubMed] [Google Scholar]
- Kono Y., Sugiura Y. Electron spin resonance studies on the oxidation of rifamycin SV catalyzed by metal ions. J Biochem. 1982 Jan;91(1):397–401. doi: 10.1093/oxfordjournals.jbchem.a133699. [DOI] [PubMed] [Google Scholar]
- Kukiełka E., Cederbaum A. I. NADH-dependent microsomal interaction with ferric complexes and production of reactive oxygen intermediates. Arch Biochem Biophys. 1989 Dec;275(2):540–550. doi: 10.1016/0003-9861(89)90400-1. [DOI] [PubMed] [Google Scholar]
- Kukiełka E., Cederbaum A. I. Stimulation of microsomal production of reactive oxygen intermediates by rifamycin SV: effect of ferric complexes and comparisons between NADPH and NADH. Arch Biochem Biophys. 1992 Nov 1;298(2):602–611. doi: 10.1016/0003-9861(92)90455-6. [DOI] [PubMed] [Google Scholar]
- Kukiełka E., Puntarulo S., Cederbaum A. I. Interaction of ferric complexes with rat liver nuclei to catalyze NADH-and NADPH-Dependent production of oxygen radicals. Arch Biochem Biophys. 1989 Sep;273(2):319–330. doi: 10.1016/0003-9861(89)90490-6. [DOI] [PubMed] [Google Scholar]
- LOWRY O. H., ROSEBROUGH N. J., FARR A. L., RANDALL R. J. Protein measurement with the Folin phenol reagent. J Biol Chem. 1951 Nov;193(1):265–275. [PubMed] [Google Scholar]
- Lees A. W., Allan G. W., Smith J., Tyrrell W. F., Fallon R. J. Toxicity form rifampicin plus isoniazid and rifampicin plus ethambutol therapy. Tubercle. 1971 Sep;52(3):182–190. doi: 10.1016/0041-3879(71)90041-9. [DOI] [PubMed] [Google Scholar]
- Lees A. W., Asgher B., Hashem M. A., Sinha B. N. Jaundice after rifampicin. Br J Dis Chest. 1970 Apr;64(2):90–95. doi: 10.1016/s0007-0971(70)80034-1. [DOI] [PubMed] [Google Scholar]
- Lesobre R., Ruffino J., Teyssier L., Achard F., Brefort G. Les ictères au cours du traitement par la rifampicine (12 observations) Rev Tuberc Pneumol (Paris) 1969 Apr-May;33(3):393–403. [PubMed] [Google Scholar]
- Lester W. Rifampin: a semisynthetic derivative of rifamycin--a prototype for the future. Annu Rev Microbiol. 1972;26:85–102. doi: 10.1146/annurev.mi.26.100172.000505. [DOI] [PubMed] [Google Scholar]
- Lind C., Ernster L. A possible relationship between DT diaphorase and the aryl hydrocarbon hydroxylase system. Biochem Biophys Res Commun. 1974 Jan 23;56(2):392–400. doi: 10.1016/0006-291x(74)90855-9. [DOI] [PubMed] [Google Scholar]
- Liochev S., Fridovich I. Superoxide is responsible for the vanadate stimulation of NAD(P)H oxidation by biological membranes. Arch Biochem Biophys. 1988 Jun;263(2):299–304. doi: 10.1016/0003-9861(88)90639-x. [DOI] [PubMed] [Google Scholar]
- Minotti G. tert-butyl hydroperoxide-dependent microsomal release of iron and lipid peroxidation. I. Evidence for the reductive release of nonheme, nonferritin iron. Arch Biochem Biophys. 1989 Aug 15;273(1):137–143. doi: 10.1016/0003-9861(89)90171-9. [DOI] [PubMed] [Google Scholar]
- Montgomery M. R., Clark C., Holtzman J. L. Iron species of hepatic microsomes from control and phenobarbital-treated rats. Arch Biochem Biophys. 1974 Jan;160(1):113–118. doi: 10.1016/s0003-9861(74)80015-9. [DOI] [PubMed] [Google Scholar]
- Morehouse L. A., Aust S. D. Reconstituted microsomal lipid peroxidation: ADP-Fe3+-dependent peroxidation of phospholipid vesicles containing NADPH-cytochrome P450 reductase and cytochrome P450. Free Radic Biol Med. 1988;4(5):269–277. doi: 10.1016/0891-5849(88)90047-0. [DOI] [PubMed] [Google Scholar]
- Morehouse L. A., Thomas C. E., Aust S. D. Superoxide generation by NADPH-cytochrome P-450 reductase: the effect of iron chelators and the role of superoxide in microsomal lipid peroxidation. Arch Biochem Biophys. 1984 Jul;232(1):366–377. doi: 10.1016/0003-9861(84)90552-6. [DOI] [PubMed] [Google Scholar]
- Muindi J., Sinha B. K., Gianni L., Myers C. Thiol-dependent DNA damage produced by anthracycline-iron complexes. The structure-activity relationships and molecular mechanisms. Mol Pharmacol. 1985 Mar;27(3):356–365. [PubMed] [Google Scholar]
- Myers C. E., Muindi J. R., Zweier J., Sinha B. K. 5-Iminodaunomycin. An anthracycline with unique properties. J Biol Chem. 1987 Aug 25;262(24):11571–11577. [PubMed] [Google Scholar]
- Pallanza R., Arioli V., Furesz S., Bolzoni G. Rifampicin: a new rifamycin. II. Laboratory studies on the antituberculous activity and preliminary clinical observations. Arzneimittelforschung. 1967 May;17(5):529–534. [PubMed] [Google Scholar]
- Powis G. Metabolism and reactions of quinoid anticancer agents. Pharmacol Ther. 1987;35(1-2):57–162. doi: 10.1016/0163-7258(87)90105-7. [DOI] [PubMed] [Google Scholar]
- Puntarulo S., Cederbaum A. I. Comparison of the ability of ferric complexes to catalyze microsomal chemiluminescence, lipid peroxidation, and hydroxyl radical generation. Arch Biochem Biophys. 1988 Aug 1;264(2):482–491. doi: 10.1016/0003-9861(88)90313-x. [DOI] [PubMed] [Google Scholar]
- Quinlan G. J., Gutteridge J. M. DNA base damage by beta-lactam, tetracycline, bacitracin and rifamycin antibacterial antibiotics. Biochem Pharmacol. 1991 Sep 27;42(8):1595–1599. doi: 10.1016/0006-2952(91)90429-9. [DOI] [PubMed] [Google Scholar]
- Quinlan G. J., Gutteridge J. M. Oxygen radical damage to DNA by rifamycin SV and copper ions. Biochem Pharmacol. 1987 Nov 1;36(21):3629–3633. doi: 10.1016/0006-2952(87)90012-8. [DOI] [PubMed] [Google Scholar]
- Rumyantseva G. V., Weiner L. M., Frolova E. I., Fedorova O. S. Hydroxyl radical generation and DNA strand scission mediated by natural anticancer and synthetic quinones. FEBS Lett. 1989 Jan 2;242(2):397–400. doi: 10.1016/0014-5793(89)80509-5. [DOI] [PubMed] [Google Scholar]
- SENSI P., GRECO A. M., BALLOTTA R. Rifomycin. I. Isolation and properties of rifomycin B and rifomycin complex. Antibiot Annu. 1959;7:262–270. [PubMed] [Google Scholar]
- Schneider J. E., Browning M. M., Floyd R. A. Ascorbate/iron mediation of hydroxyl free radical damage to PBR322 plasmid DNA. Free Radic Biol Med. 1988;5(5-6):287–295. doi: 10.1016/0891-5849(88)90099-8. [DOI] [PubMed] [Google Scholar]
- Schneider J. E., Browning M. M., Zhu X., Eneff K. L., Floyd R. A. Characterization of hydroxyl free radical mediated damage to plasmid pBR322 DNA. Mutat Res. 1989 Sep;214(1):23–31. doi: 10.1016/0027-5107(89)90194-2. [DOI] [PubMed] [Google Scholar]
- Scrutton M. C. Divalent metal ion catalysis of the oxidation of rifamycin SV to rifamycin S. FEBS Lett. 1977 Jun 15;78(2):216–220. doi: 10.1016/0014-5793(77)80309-8. [DOI] [PubMed] [Google Scholar]
- Sinha B. K., Eliot H. M., Kalayanaraman B. Iron-dependent hydroxyl radical formation and DNA damage from a novel metabolite of the clinically active antitumor drug VP-16. FEBS Lett. 1988 Jan 25;227(2):240–244. doi: 10.1016/0014-5793(88)80906-2. [DOI] [PubMed] [Google Scholar]
- Subak-Sharpe J. H., Timbury M. C., Williams J. F. Rifampicin inhibits the growth of some mammalian viruses. Nature. 1969 Apr 26;222(5191):341–345. doi: 10.1038/222341a0. [DOI] [PubMed] [Google Scholar]
- Sáez G. T., Valls V., Cabedo H., Iradi A., Bannister W. H., Bannister J. V. Effect of metal ion catalyzed oxidation of rifamycin SV on cell viability and metabolic performance of isolated rat hepatocytes. Biochim Biophys Acta. 1991 May 17;1092(3):326–335. doi: 10.1016/s0167-4889(97)90008-2. [DOI] [PubMed] [Google Scholar]
- Thor H., Smith M. T., Hartzell P., Bellomo G., Jewell S. A., Orrenius S. The metabolism of menadione (2-methyl-1,4-naphthoquinone) by isolated hepatocytes. A study of the implications of oxidative stress in intact cells. J Biol Chem. 1982 Oct 25;257(20):12419–12425. [PubMed] [Google Scholar]
- Trush M. A., Mimnaugh E. G., Ginsburg E., Gram T. E. In vitro stimulation by paraquat of reactive oxygen-mediated lipid peroxidation in rat lung microsomes. Toxicol Appl Pharmacol. 1981 Sep 15;60(2):279–286. doi: 10.1016/0041-008x(91)90231-3. [DOI] [PubMed] [Google Scholar]
- Trush M. A., Mimnaugh E. G., Gram T. E. Activation of pharmacologic agents to radical intermediates. Implications for the role of free radicals in drug action and toxicity. Biochem Pharmacol. 1982 Nov 1;31(21):3335–3346. doi: 10.1016/0006-2952(82)90609-8. [DOI] [PubMed] [Google Scholar]
- Végh M., Marton A., Horváth I. Reduction of Fe(III)ADP complex by liver microsomes. Biochim Biophys Acta. 1988 Feb 17;964(2):146–150. doi: 10.1016/0304-4165(88)90160-2. [DOI] [PubMed] [Google Scholar]