Abstract
Antibodies were raised against synthetic peptides corresponding to the N-terminal (residues 2-17) and C-terminal (residues 691-706) ends of rabbit skeletal muscle triadin, a 95 kDa protein located in the sarcoplasmic reticulum membrane at the triad junction. The specificity of the antibodies generated was tested by ELISA and Western blot analysis. These tests demonstrated the ability of the antibodies to react specifically with the proteins. The anti-N-terminus antibodies bound to sarcoplasmic reticulum vesicles, indicating that the N-terminal end of the membrane-embedded triadin is exposed on the cytoplasmic side of the vesicles. In contrast, the anti-C-terminus antibodies were able to react with sarcoplasmic reticulum vesicles only after permeabilization of the vesicles with a detergent, indicating that the C-terminal end is exposed on the luminal side of the vesicles. These immunological data were complemented by proteolysis experiments using carboxypeptidases and endoproteinase Arg C. A mixture of carboxypeptidases A, B and Y was used to induce degradation of the C-terminal end of triadin in sarcoplasmic reticulum vesicles. This degradation, and a concomitant loss of reactivity of the anti-C-terminus antibodies in Western blots, was observed only when the vesicles were permeabilized, providing further evidence for the luminal localization of the C-terminal end of triadin. Treatment of sarcoplasmic reticulum vesicles with endoproteinase Arg C resulted in the removal of the N-terminal end of triadin, probably due to cleavage after Arg-34. This is a further indication of the cytoplasmic localization of the N-terminal end of triadin (and of its first 34 amino acids). When the proteolysis with endoproteinase Arg C was carried out with permeabilized vesicles, the cleavage occurred after Arg-141 or Arg-157, indicating that at least one of these residues is luminal.
Full text
PDF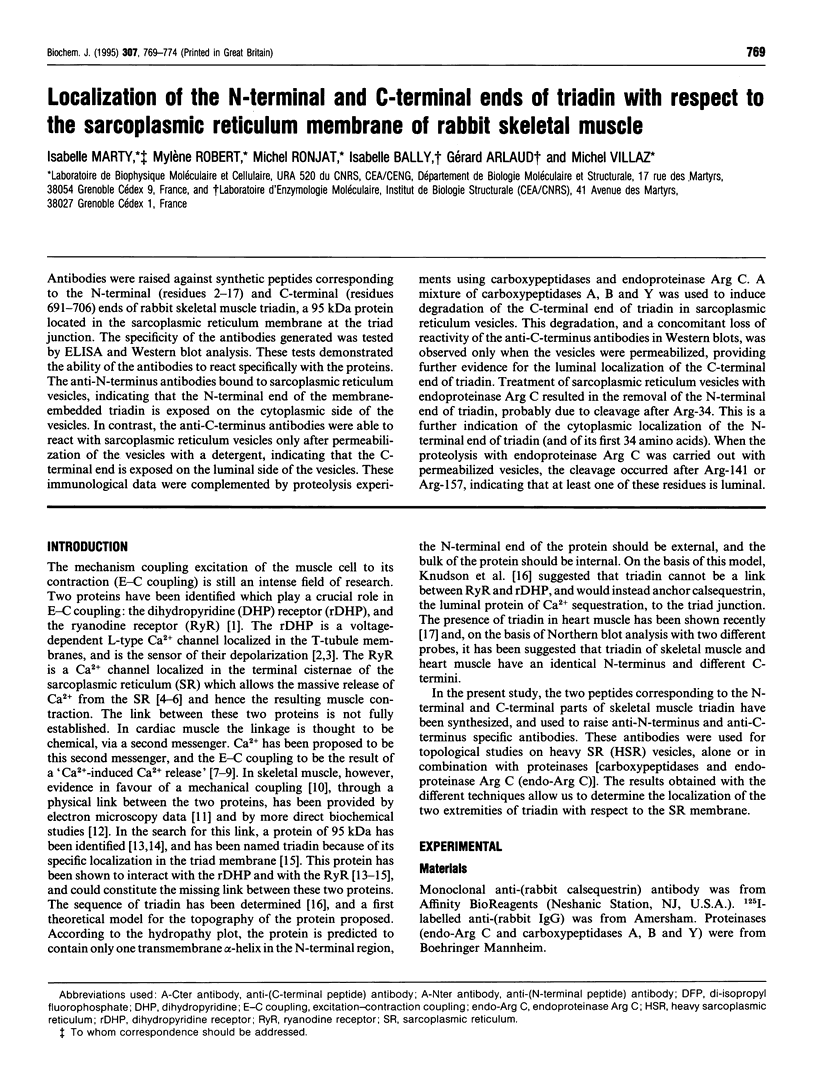
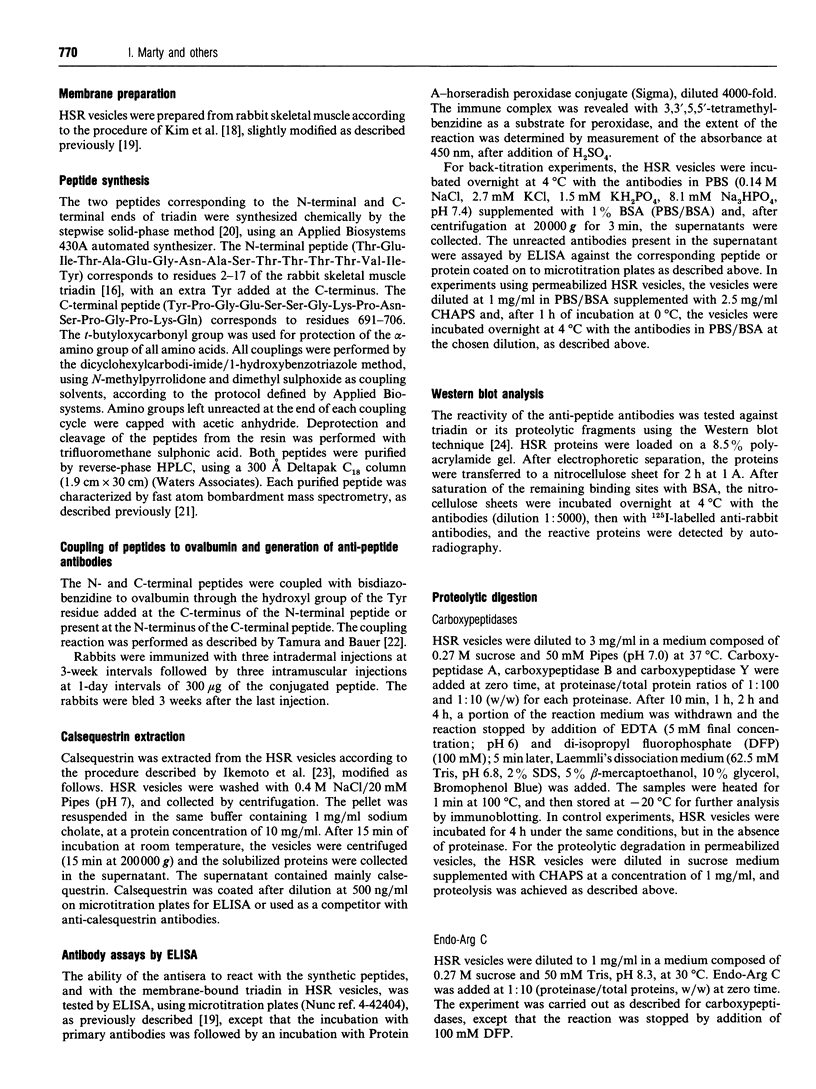
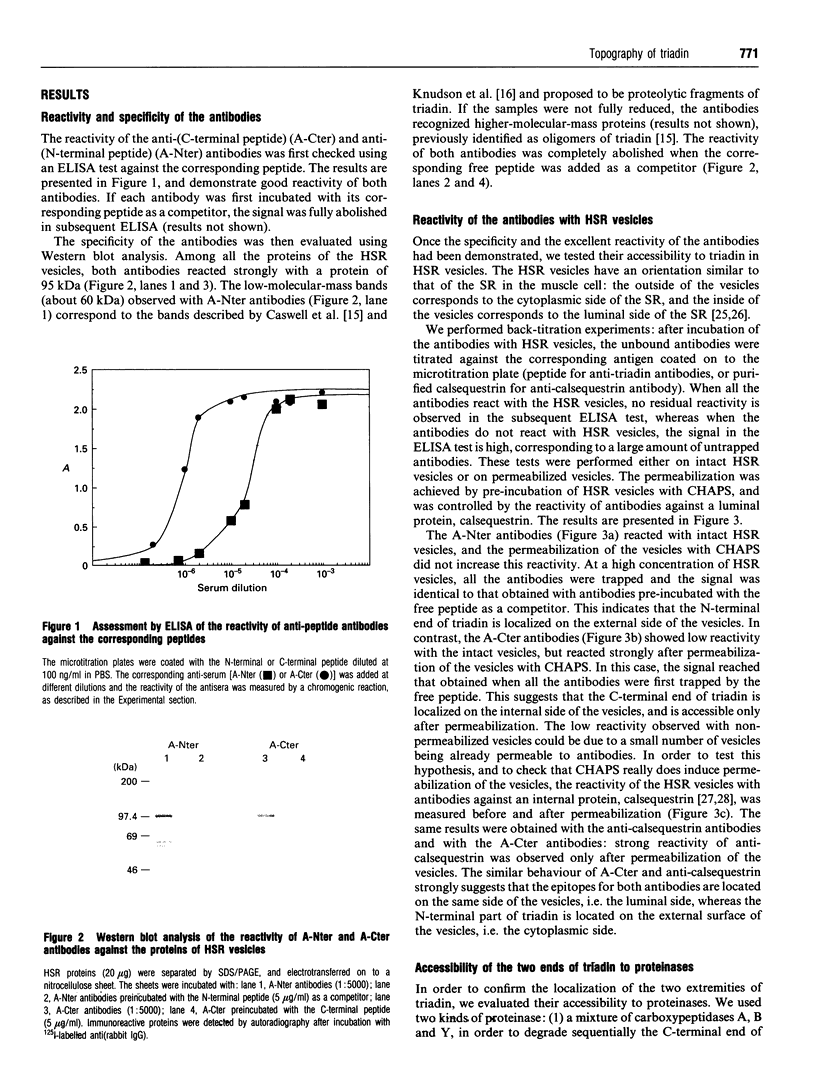
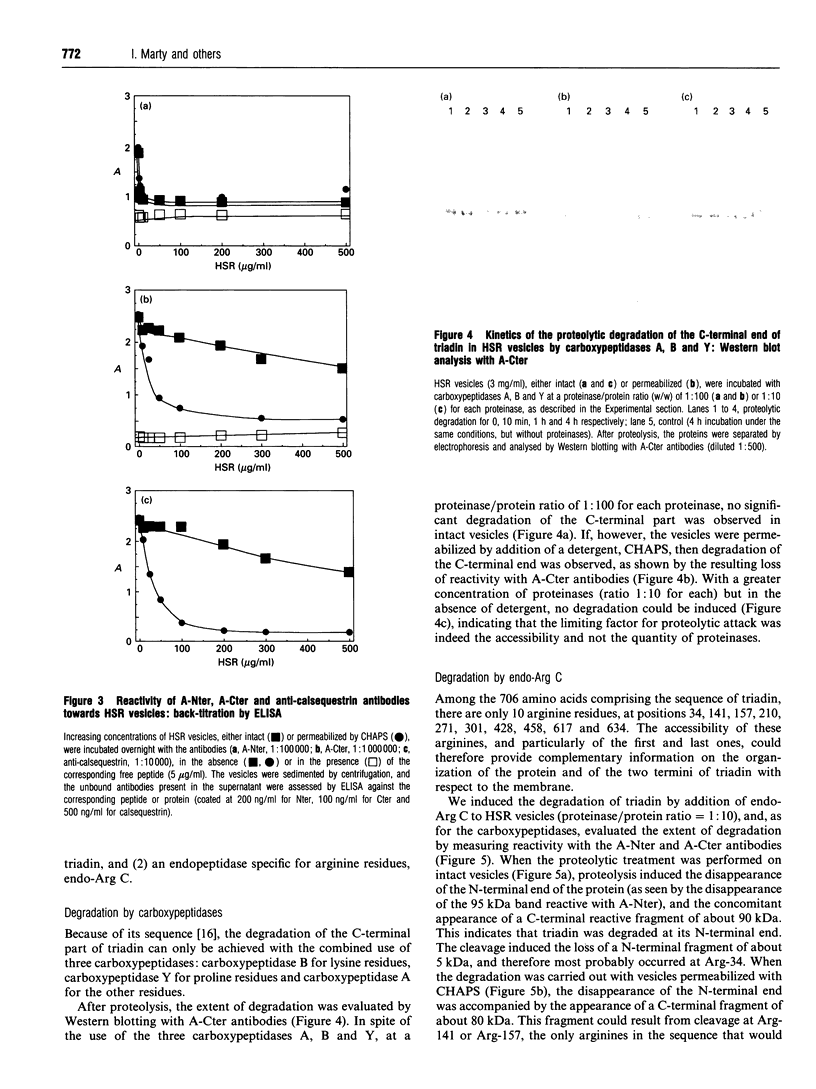
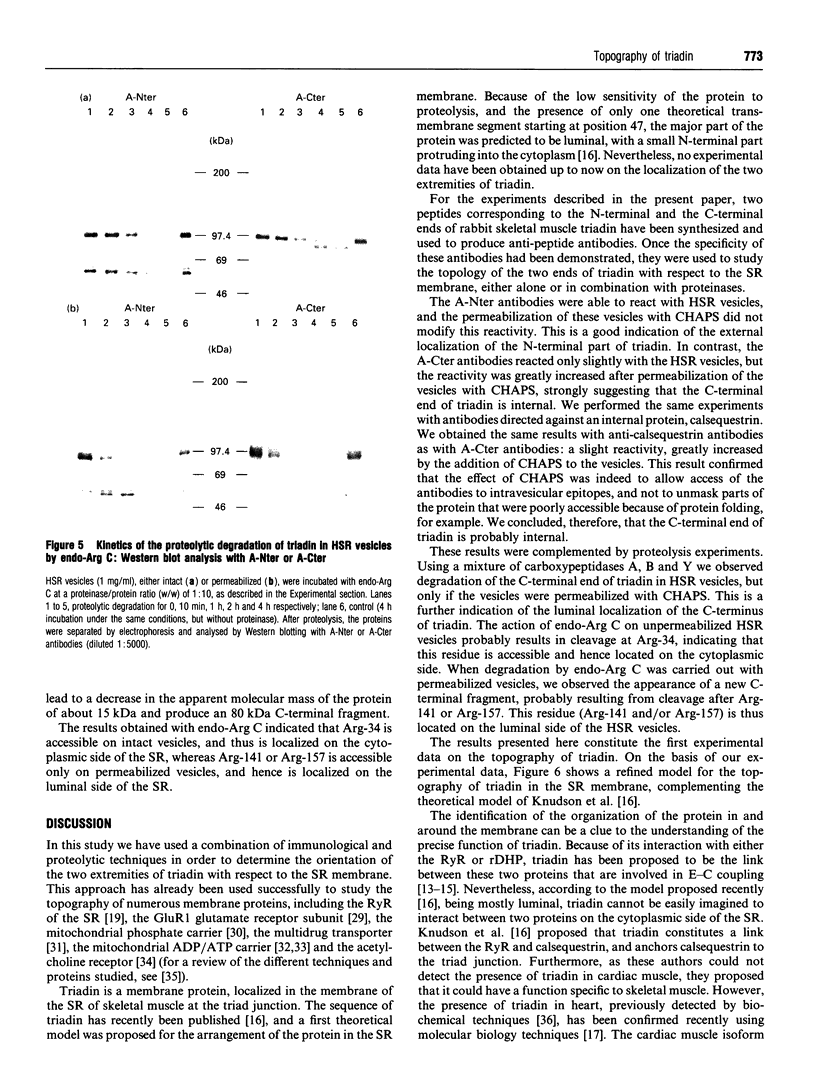
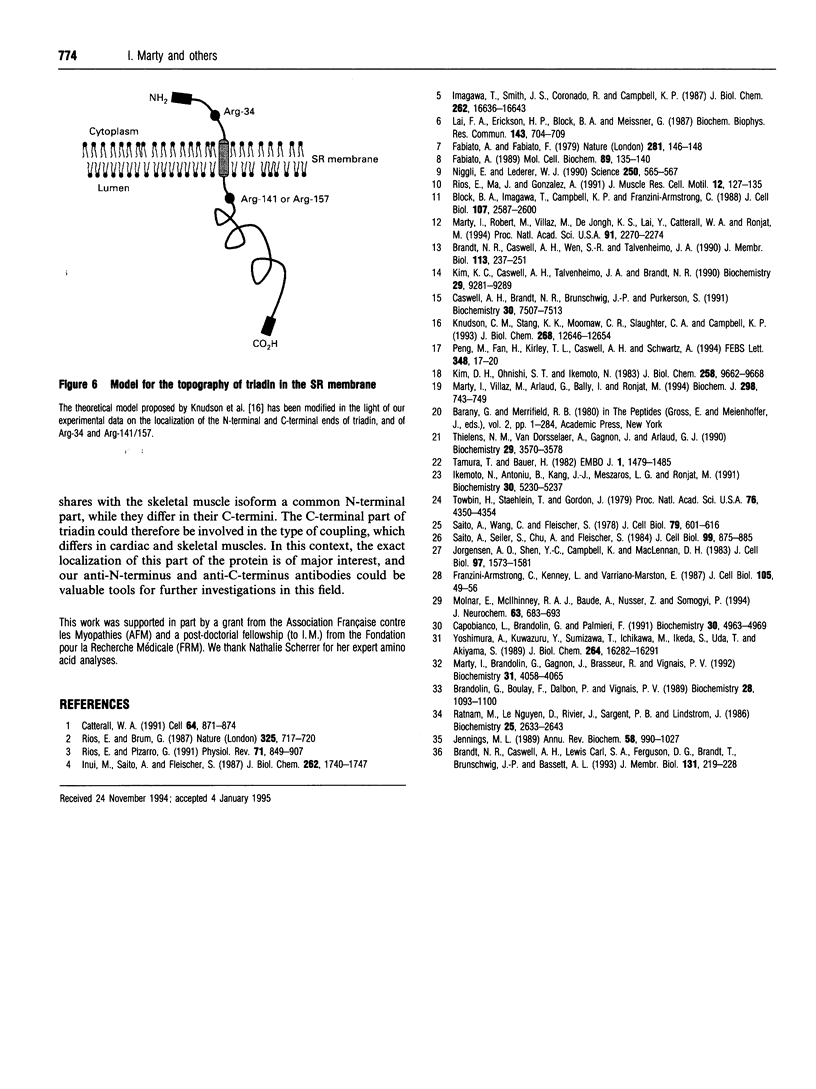
Images in this article
Selected References
These references are in PubMed. This may not be the complete list of references from this article.
- Block B. A., Imagawa T., Campbell K. P., Franzini-Armstrong C. Structural evidence for direct interaction between the molecular components of the transverse tubule/sarcoplasmic reticulum junction in skeletal muscle. J Cell Biol. 1988 Dec;107(6 Pt 2):2587–2600. doi: 10.1083/jcb.107.6.2587. [DOI] [PMC free article] [PubMed] [Google Scholar]
- Brandolin G., Boulay F., Dalbon P., Vignais P. V. Orientation of the N-terminal region of the membrane-bound ADP/ATP carrier protein explored by antipeptide antibodies and an arginine-specific endoprotease. Evidence that the accessibility of the N-terminal residues depends on the conformational state of the carrier. Biochemistry. 1989 Feb 7;28(3):1093–1100. doi: 10.1021/bi00429a025. [DOI] [PubMed] [Google Scholar]
- Brandt N. R., Caswell A. H., Carl S. A., Ferguson D. G., Brandt T., Brunschwig J. P., Bassett A. L. Detection and localization of triadin in rat ventricular muscle. J Membr Biol. 1993 Feb;131(3):219–228. doi: 10.1007/BF02260110. [DOI] [PubMed] [Google Scholar]
- Brandt N. R., Caswell A. H., Wen S. R., Talvenheimo J. A. Molecular interactions of the junctional foot protein and dihydropyridine receptor in skeletal muscle triads. J Membr Biol. 1990 Feb;113(3):237–251. doi: 10.1007/BF01870075. [DOI] [PubMed] [Google Scholar]
- Capobianco L., Brandolin G., Palmieri F. Transmembrane topography of the mitochondrial phosphate carrier explored by peptide-specific antibodies and enzymatic digestion. Biochemistry. 1991 May 21;30(20):4963–4969. doi: 10.1021/bi00234a018. [DOI] [PubMed] [Google Scholar]
- Caswell A. H., Brandt N. R., Brunschwig J. P., Purkerson S. Localization and partial characterization of the oligomeric disulfide-linked molecular weight 95,000 protein (triadin) which binds the ryanodine and dihydropyridine receptors in skeletal muscle triadic vesicles. Biochemistry. 1991 Jul 30;30(30):7507–7513. doi: 10.1021/bi00244a020. [DOI] [PubMed] [Google Scholar]
- Catterall W. A. Excitation-contraction coupling in vertebrate skeletal muscle: a tale of two calcium channels. Cell. 1991 Mar 8;64(5):871–874. doi: 10.1016/0092-8674(91)90309-m. [DOI] [PubMed] [Google Scholar]
- Fabiato A. Appraisal of the physiological relevance of two hypothesis for the mechanism of calcium release from the mammalian cardiac sarcoplasmic reticulum: calcium-induced release versus charge-coupled release. Mol Cell Biochem. 1989 Sep 7;89(2):135–140. doi: 10.1007/BF00220765. [DOI] [PubMed] [Google Scholar]
- Fabiato A., Fabiato F. Use of chlorotetracycline fluorescence to demonstrate Ca2+-induced release of Ca2+ from the sarcoplasmic reticulum of skinned cardiac cells. Nature. 1979 Sep 13;281(5727):146–148. doi: 10.1038/281146a0. [DOI] [PubMed] [Google Scholar]
- Franzini-Armstrong C., Kenney L. J., Varriano-Marston E. The structure of calsequestrin in triads of vertebrate skeletal muscle: a deep-etch study. J Cell Biol. 1987 Jul;105(1):49–56. doi: 10.1083/jcb.105.1.49. [DOI] [PMC free article] [PubMed] [Google Scholar]
- Ikemoto N., Antoniu B., Kang J. J., Mészáros L. G., Ronjat M. Intravesicular calcium transient during calcium release from sarcoplasmic reticulum. Biochemistry. 1991 May 28;30(21):5230–5237. doi: 10.1021/bi00235a017. [DOI] [PubMed] [Google Scholar]
- Imagawa T., Smith J. S., Coronado R., Campbell K. P. Purified ryanodine receptor from skeletal muscle sarcoplasmic reticulum is the Ca2+-permeable pore of the calcium release channel. J Biol Chem. 1987 Dec 5;262(34):16636–16643. [PubMed] [Google Scholar]
- Inui M., Saito A., Fleischer S. Purification of the ryanodine receptor and identity with feet structures of junctional terminal cisternae of sarcoplasmic reticulum from fast skeletal muscle. J Biol Chem. 1987 Feb 5;262(4):1740–1747. [PubMed] [Google Scholar]
- Jennings M. L. Topography of membrane proteins. Annu Rev Biochem. 1989;58:999–1027. doi: 10.1146/annurev.bi.58.070189.005031. [DOI] [PubMed] [Google Scholar]
- Jorgensen A. O., Shen A. C., Campbell K. P., MacLennan D. H. Ultrastructural localization of calsequestrin in rat skeletal muscle by immunoferritin labeling of ultrathin frozen sections. J Cell Biol. 1983 Nov;97(5 Pt 1):1573–1581. doi: 10.1083/jcb.97.5.1573. [DOI] [PMC free article] [PubMed] [Google Scholar]
- Kim D. H., Ohnishi S. T., Ikemoto N. Kinetic studies of calcium release from sarcoplasmic reticulum in vitro. J Biol Chem. 1983 Aug 25;258(16):9662–9668. [PubMed] [Google Scholar]
- Kim K. C., Caswell A. H., Talvenheimo J. A., Brandt N. R. Isolation of a terminal cisterna protein which may link the dihydropyridine receptor to the junctional foot protein in skeletal muscle. Biochemistry. 1990 Oct 2;29(39):9281–9289. doi: 10.1021/bi00491a025. [DOI] [PubMed] [Google Scholar]
- Knudson C. M., Stang K. K., Moomaw C. R., Slaughter C. A., Campbell K. P. Primary structure and topological analysis of a skeletal muscle-specific junctional sarcoplasmic reticulum glycoprotein (triadin). J Biol Chem. 1993 Jun 15;268(17):12646–12654. [PubMed] [Google Scholar]
- Lai F. A., Erickson H., Block B. A., Meissner G. Evidence for a junctional feet-ryanodine receptor complex from sarcoplasmic reticulum. Biochem Biophys Res Commun. 1987 Mar 13;143(2):704–709. doi: 10.1016/0006-291x(87)91411-2. [DOI] [PubMed] [Google Scholar]
- Marty I., Brandolin G., Gagnon J., Brasseur R., Vignais P. V. Topography of the membrane-bound ADP/ATP carrier assessed by enzymatic proteolysis. Biochemistry. 1992 Apr 28;31(16):4058–4065. doi: 10.1021/bi00131a023. [DOI] [PubMed] [Google Scholar]
- Marty I., Robert M., Villaz M., De Jongh K., Lai Y., Catterall W. A., Ronjat M. Biochemical evidence for a complex involving dihydropyridine receptor and ryanodine receptor in triad junctions of skeletal muscle. Proc Natl Acad Sci U S A. 1994 Mar 15;91(6):2270–2274. doi: 10.1073/pnas.91.6.2270. [DOI] [PMC free article] [PubMed] [Google Scholar]
- Marty I., Villaz M., Arlaud G., Bally I., Ronjat M. Transmembrane orientation of the N-terminal and C-terminal ends of the ryanodine receptor in the sarcoplasmic reticulum of rabbit skeletal muscle. Biochem J. 1994 Mar 15;298(Pt 3):743–749. doi: 10.1042/bj2980743. [DOI] [PMC free article] [PubMed] [Google Scholar]
- Molnár E., McIlhinney R. A., Baude A., Nusser Z., Somogyi P. Membrane topology of the GluR1 glutamate receptor subunit: epitope mapping by site-directed antipeptide antibodies. J Neurochem. 1994 Aug;63(2):683–693. doi: 10.1046/j.1471-4159.1994.63020683.x. [DOI] [PubMed] [Google Scholar]
- Niggli E., Lederer W. J. Voltage-independent calcium release in heart muscle. Science. 1990 Oct 26;250(4980):565–568. doi: 10.1126/science.2173135. [DOI] [PubMed] [Google Scholar]
- Peng M., Fan H., Kirley T. L., Caswell A. H., Schwartz A. Structural diversity of triadin in skeletal muscle and evidence of its existence in heart. FEBS Lett. 1994 Jul 4;348(1):17–20. doi: 10.1016/0014-5793(94)00556-7. [DOI] [PubMed] [Google Scholar]
- Ratnam M., Nguyen D. L., Rivier J., Sargent P. B., Lindstrom J. Transmembrane topography of nicotinic acetylcholine receptor: immunochemical tests contradict theoretical predictions based on hydrophobicity profiles. Biochemistry. 1986 May 6;25(9):2633–2643. doi: 10.1021/bi00357a052. [DOI] [PubMed] [Google Scholar]
- Rios E., Brum G. Involvement of dihydropyridine receptors in excitation-contraction coupling in skeletal muscle. Nature. 1987 Feb 19;325(6106):717–720. doi: 10.1038/325717a0. [DOI] [PubMed] [Google Scholar]
- Ríos E., Ma J. J., González A. The mechanical hypothesis of excitation-contraction (EC) coupling in skeletal muscle. J Muscle Res Cell Motil. 1991 Apr;12(2):127–135. doi: 10.1007/BF01774031. [DOI] [PubMed] [Google Scholar]
- Ríos E., Pizarro G. Voltage sensor of excitation-contraction coupling in skeletal muscle. Physiol Rev. 1991 Jul;71(3):849–908. doi: 10.1152/physrev.1991.71.3.849. [DOI] [PubMed] [Google Scholar]
- Saito A., Seiler S., Chu A., Fleischer S. Preparation and morphology of sarcoplasmic reticulum terminal cisternae from rabbit skeletal muscle. J Cell Biol. 1984 Sep;99(3):875–885. doi: 10.1083/jcb.99.3.875. [DOI] [PMC free article] [PubMed] [Google Scholar]
- Saito A., Wang C. T., Fleischer S. Membrane asymmetry and enhanced ultrastructural detail of sarcoplasmic reticulum revealed with use of tannic acid. J Cell Biol. 1978 Dec;79(3):601–616. doi: 10.1083/jcb.79.3.601. [DOI] [PMC free article] [PubMed] [Google Scholar]
- Tamura T., Bauer H. Monoclonal antibody against the carboxy terminal peptide of pp60src of Rous sarcoma virus reacts with native pp60src. EMBO J. 1982;1(12):1479–1485. doi: 10.1002/j.1460-2075.1982.tb01343.x. [DOI] [PMC free article] [PubMed] [Google Scholar]
- Thielens N. M., Van Dorsselaer A., Gagnon J., Arlaud G. J. Chemical and functional characterization of a fragment of C1-s containing the epidermal growth factor homology region. Biochemistry. 1990 Apr 10;29(14):3570–3578. doi: 10.1021/bi00466a021. [DOI] [PubMed] [Google Scholar]
- Towbin H., Staehelin T., Gordon J. Electrophoretic transfer of proteins from polyacrylamide gels to nitrocellulose sheets: procedure and some applications. Proc Natl Acad Sci U S A. 1979 Sep;76(9):4350–4354. doi: 10.1073/pnas.76.9.4350. [DOI] [PMC free article] [PubMed] [Google Scholar]
- Yoshimura A., Kuwazuru Y., Sumizawa T., Ichikawa M., Ikeda S., Uda T., Akiyama S. Cytoplasmic orientation and two-domain structure of the multidrug transporter, P-glycoprotein, demonstrated with sequence-specific antibodies. J Biol Chem. 1989 Sep 25;264(27):16282–16291. [PubMed] [Google Scholar]