Abstract
For technical reasons, 86Rb is frequently preferred to 42K as a tracer for K+. Systematic comparisons of the two isotopes, however, are rarely done. In this paper we compare the transport of 42K and 86Rb in rat and mouse soleus muscle and in rat erythrocytes. Ouabain-suppressible K+ uptake in rat soleus was the same whether measured with 42K or 86Rb, both when stimulated by insulin, salbutamol and calcitonin-gene-related peptide (CGRP), and when inhibited by graded concentrations of ouabain. Control experiments with rat erythrocytes, where Na(+)-K(+)-Cl- co-transport has earlier been demonstrated, showed closely similar inhibitory effects of bumetanide on 42K and 86Rb uptake. In contrast, bumetanide produced no significant change in 42K uptake of rat and mouse soleus muscle, but clearly inhibited 86Rb uptake at concentrations down to 10(-7) M (P < 0.001). Whereas the addition of 150 mM NaCl had no effect on 42K uptake in rat soleus, 86Rb uptake, and in particular the bumetanide-suppressible component, was markedly increased by this addition. The inhibitory effect of bumetanide on 86Rb uptake gives rise to the false impression that skeletal muscle contains a NaKCl2 co-transport system. Efflux studies showed that the fractional loss of 42K from rat soleus muscle is 2.3 times larger than that of 86Rb. Salbutamol and CGRP increased 86Rb efflux, but inhibited 42K efflux. This implies that for studies of K+ efflux and bumetanide-sensitive K+ transport, 86Rb is not even an acceptable tracer for the detection of qualitative changes. Control experiments with 42K are essential in any characterization of unknown K+ transport processes.
Full text
PDF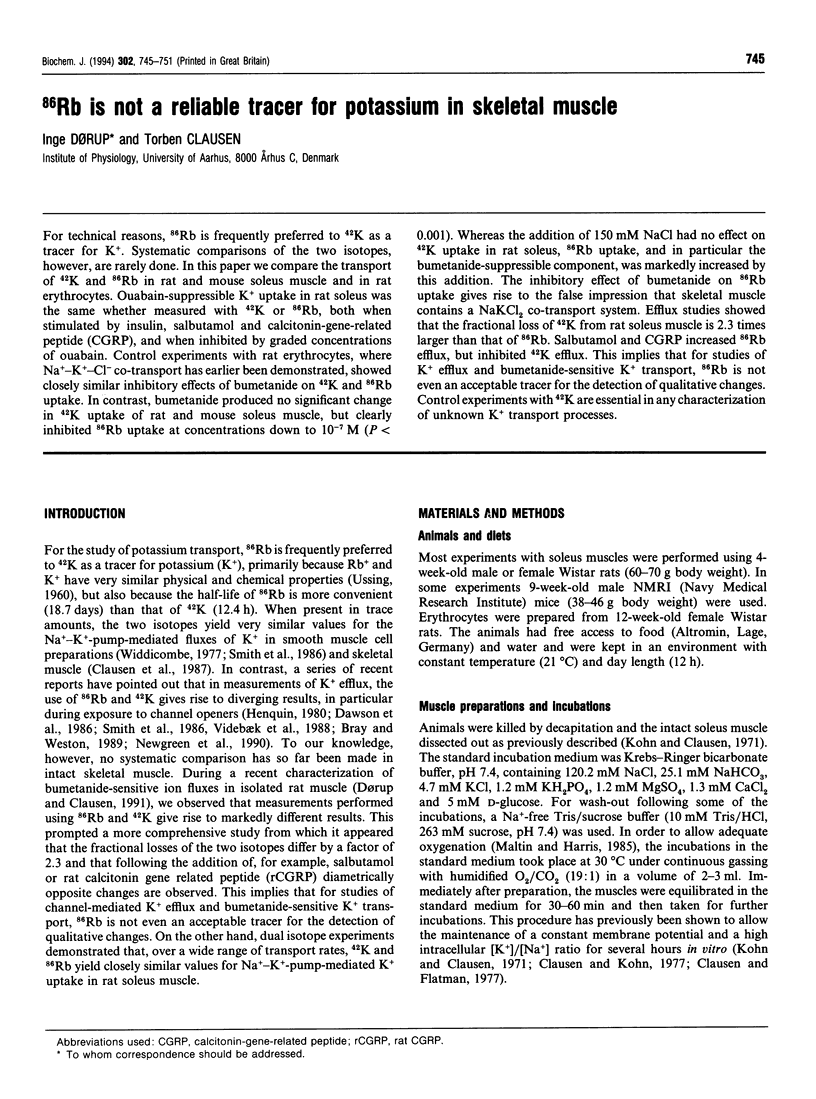
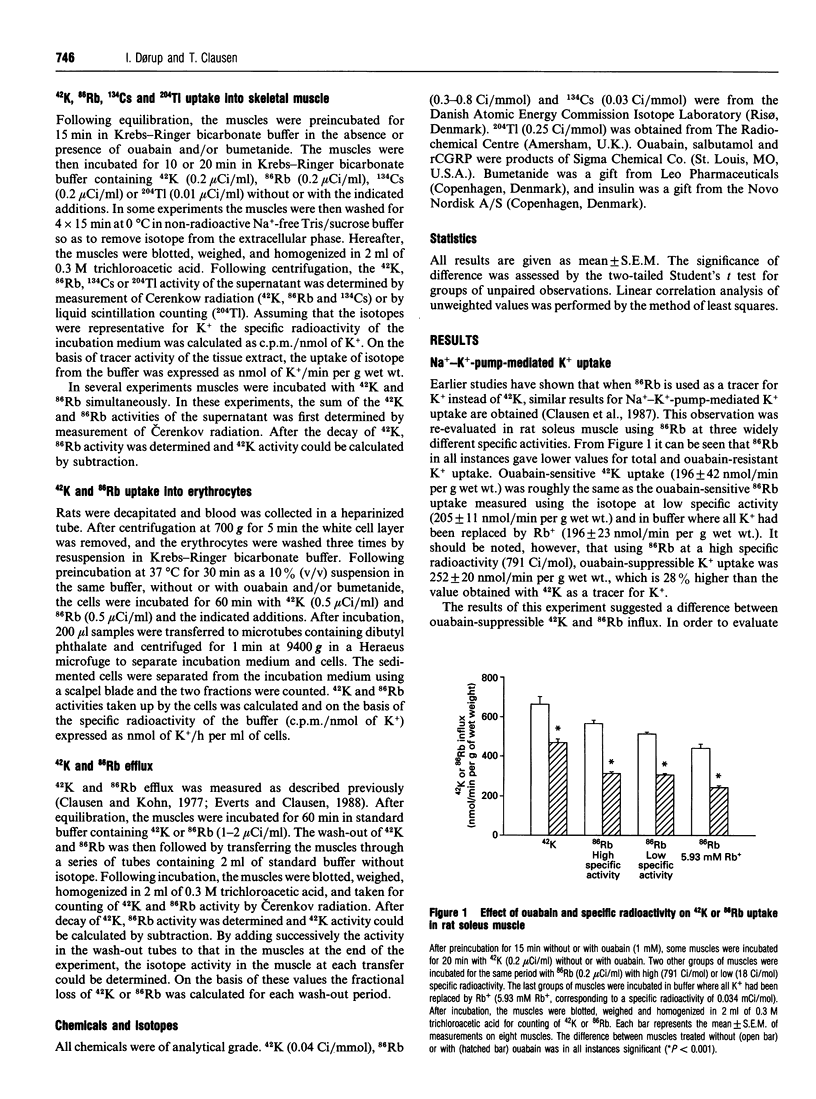
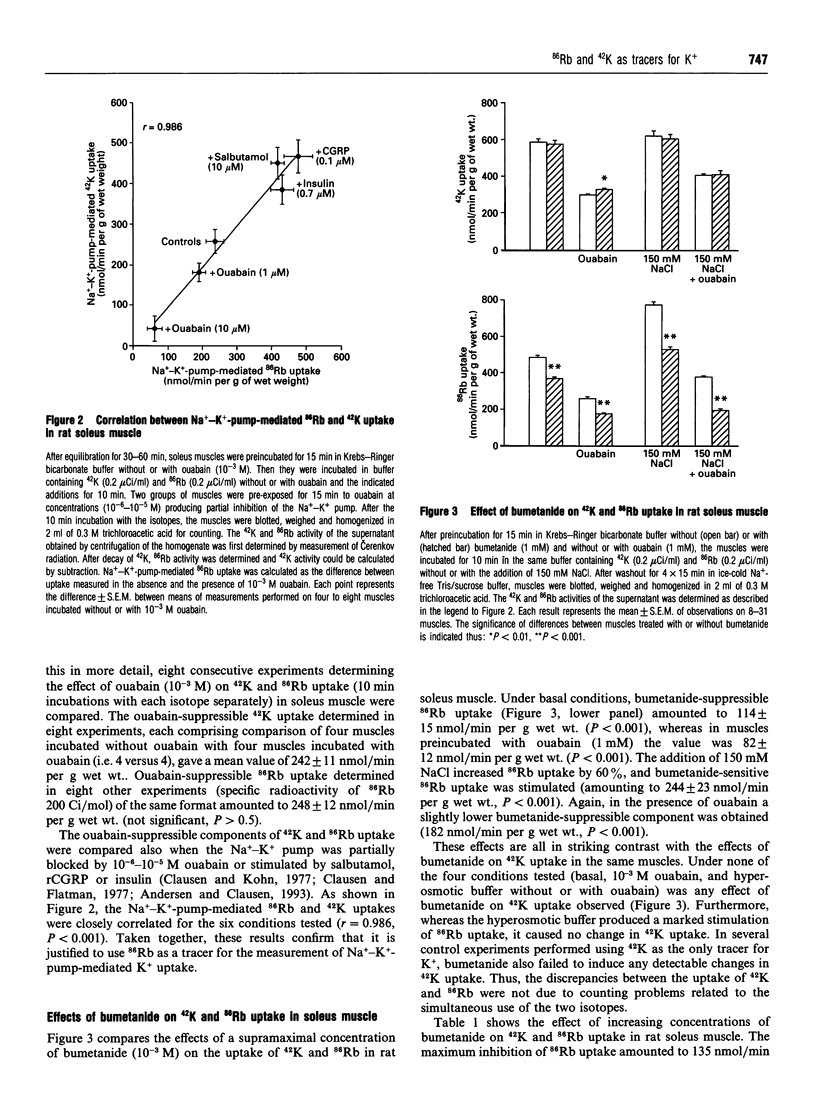
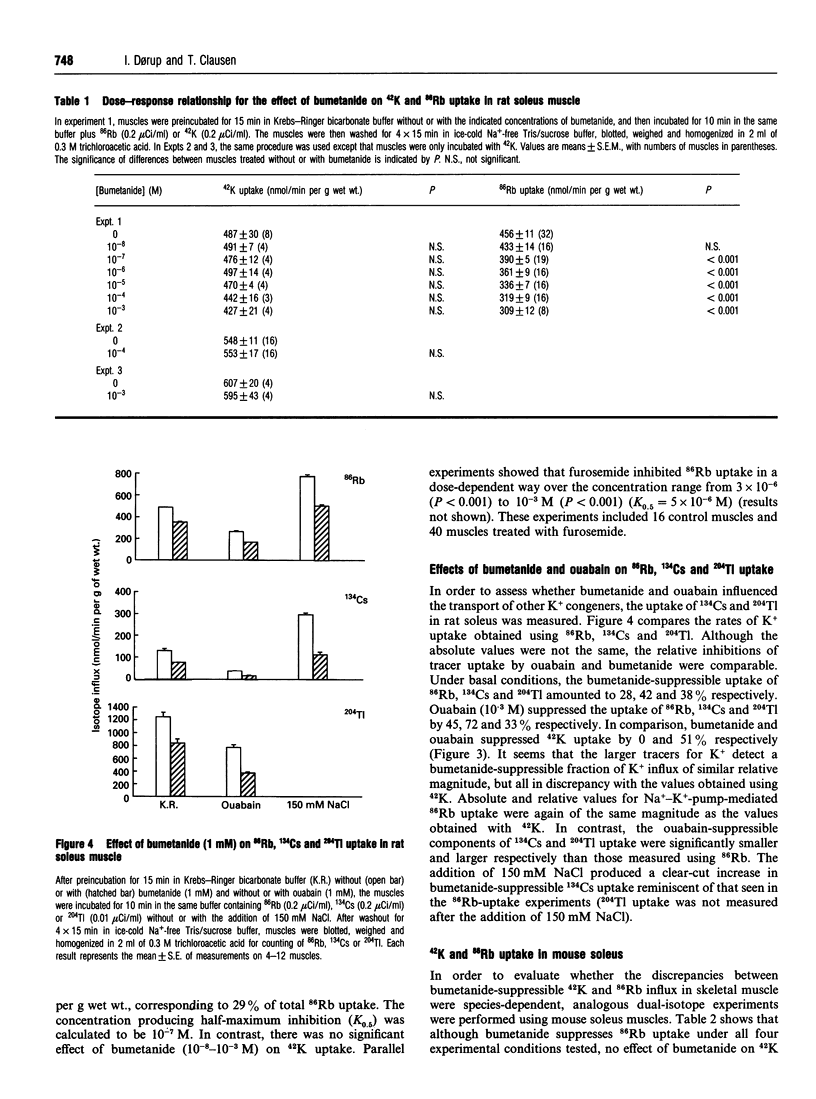
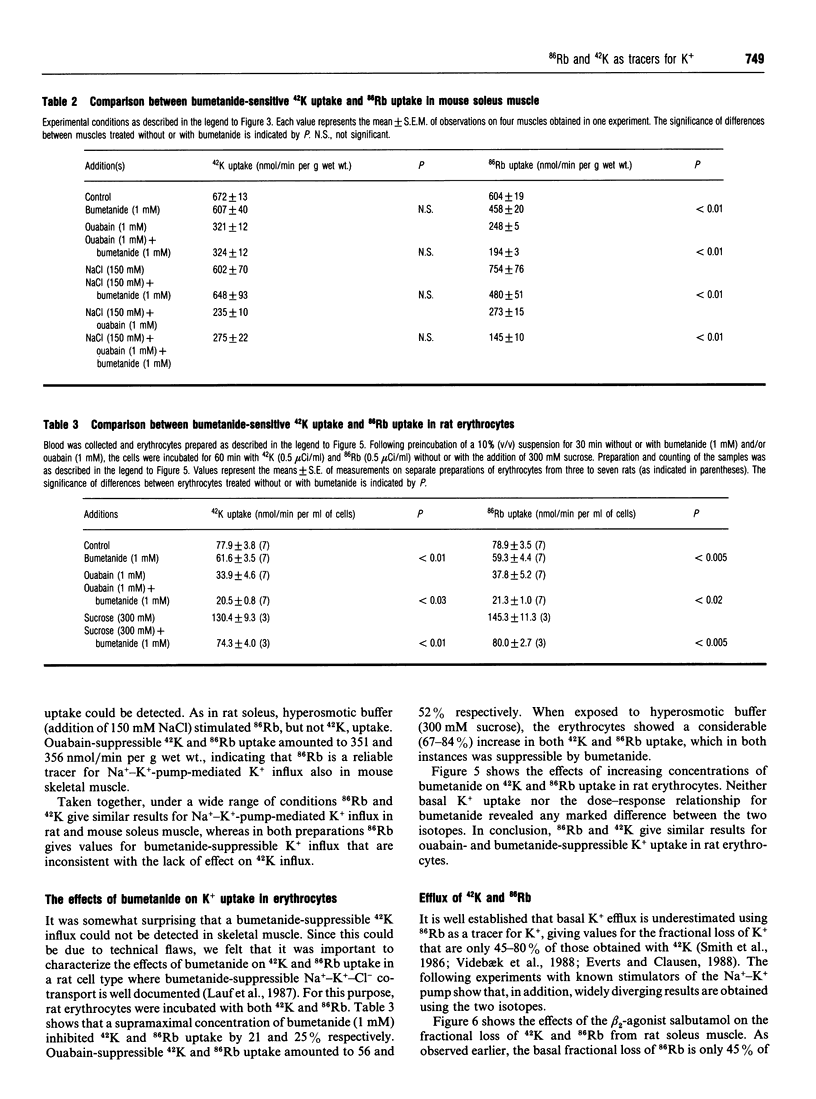
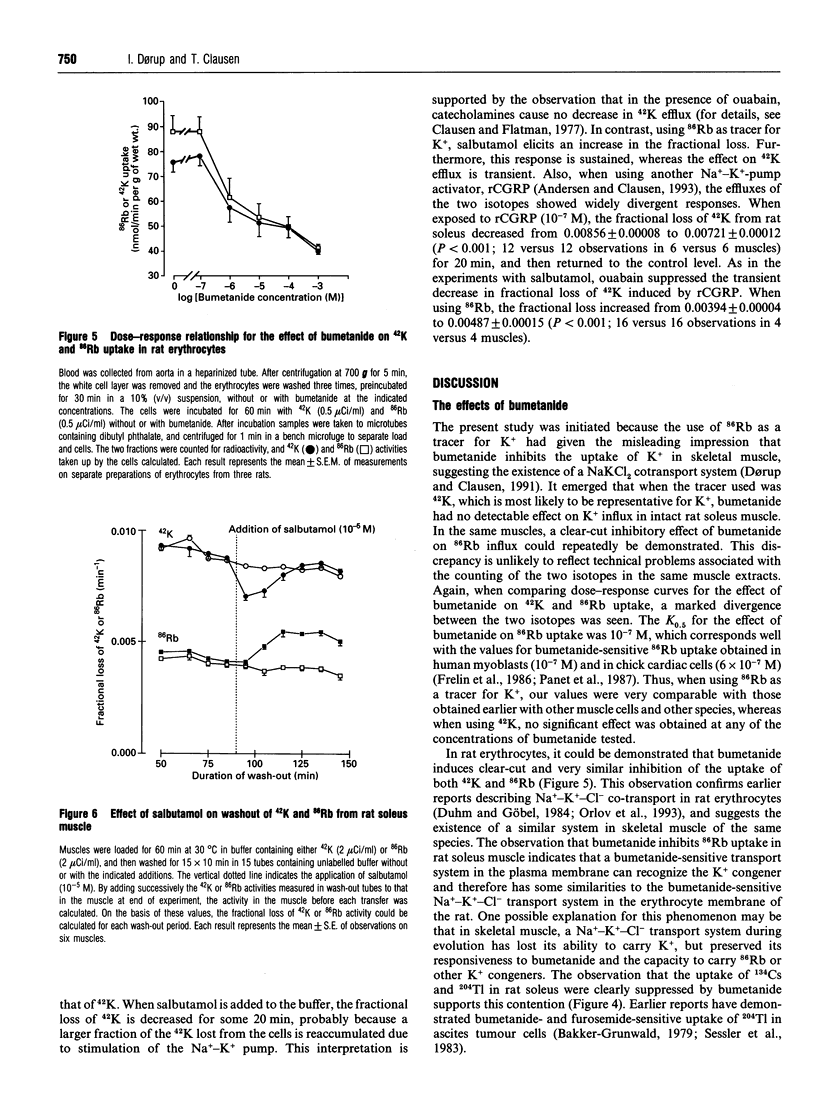
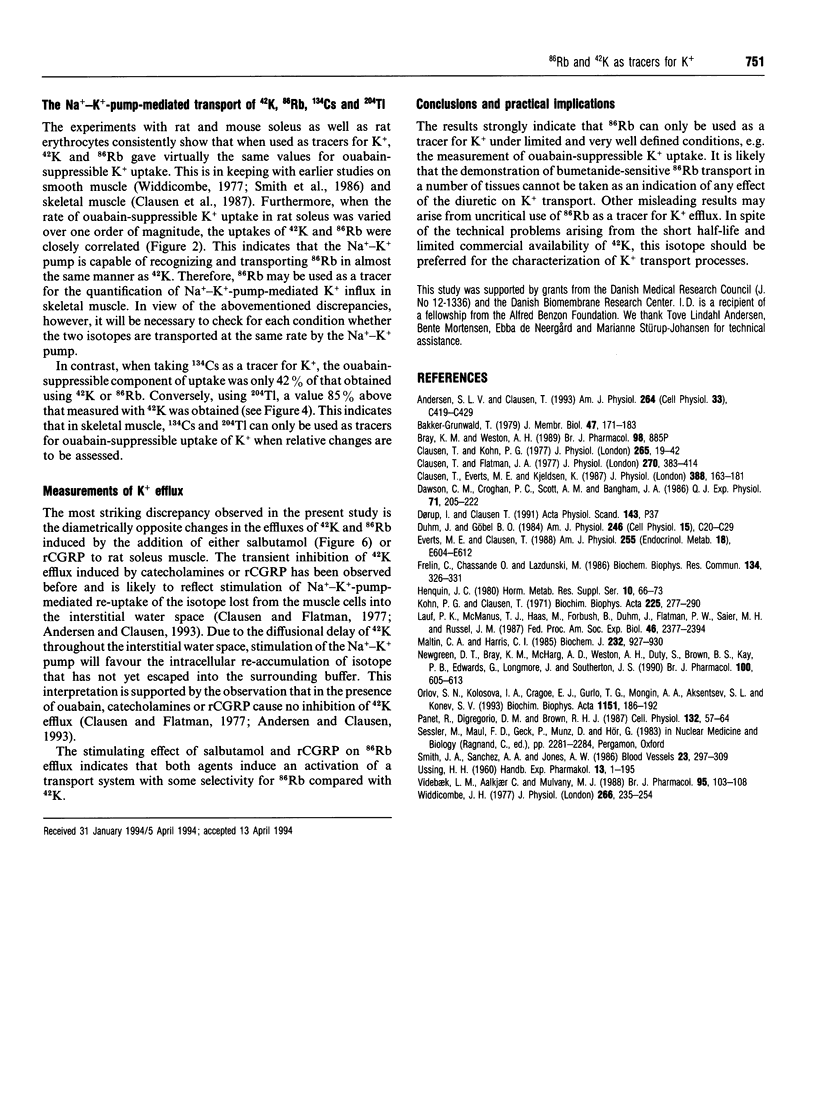
Selected References
These references are in PubMed. This may not be the complete list of references from this article.
- Andersen S. L., Clausen T. Calcitonin gene-related peptide stimulates active Na(+)-K+ transport in rat soleus muscle. Am J Physiol. 1993 Feb;264(2 Pt 1):C419–C429. doi: 10.1152/ajpcell.1993.264.2.C419. [DOI] [PubMed] [Google Scholar]
- Bakker-Grunwald T. Movement of thallous ion across the ascites cell membrane. J Membr Biol. 1979 May 21;47(2):171–183. doi: 10.1007/BF01876115. [DOI] [PubMed] [Google Scholar]
- Clausen T., Everts M. E., Kjeldsen K. Quantification of the maximum capacity for active sodium-potassium transport in rat skeletal muscle. J Physiol. 1987 Jul;388:163–181. doi: 10.1113/jphysiol.1987.sp016608. [DOI] [PMC free article] [PubMed] [Google Scholar]
- Clausen T., Flatman J. A. The effect of catecholamines on Na-K transport and membrane potential in rat soleus muscle. J Physiol. 1977 Sep;270(2):383–414. doi: 10.1113/jphysiol.1977.sp011958. [DOI] [PMC free article] [PubMed] [Google Scholar]
- Clausen T., Kohn P. G. The effect of insulin on the transport of sodium and potassium in rat soleus muscle. J Physiol. 1977 Feb;265(1):19–42. doi: 10.1113/jphysiol.1977.sp011703. [DOI] [PMC free article] [PubMed] [Google Scholar]
- Dawson C. M., Croghan P. C., Scott A. M., Bangham J. A. Potassium and rubidium permeability and potassium conductance of the beta-cell membrane in mouse islets of Langerhans. Q J Exp Physiol. 1986 Apr;71(2):205–222. doi: 10.1113/expphysiol.1986.sp002979. [DOI] [PubMed] [Google Scholar]
- Duhm J., Göbel B. O. Na+-K+ transport and volume of rat erythrocytes under dietary K+ deficiency. Am J Physiol. 1984 Jan;246(1 Pt 1):C20–C29. doi: 10.1152/ajpcell.1984.246.1.C20. [DOI] [PubMed] [Google Scholar]
- Everts M. E., Clausen T. Effects of thyroid hormone on Na+-K+ transport in resting and stimulated rat skeletal muscle. Am J Physiol. 1988 Nov;255(5 Pt 1):E604–E612. doi: 10.1152/ajpendo.1988.255.5.E604. [DOI] [PubMed] [Google Scholar]
- Frelin C., Chassande O., Lazdunski M. Biochemical characterization of the Na+/K+/Cl- co-transport in chick cardiac cells. Biochem Biophys Res Commun. 1986 Jan 14;134(1):326–331. doi: 10.1016/0006-291x(86)90566-8. [DOI] [PubMed] [Google Scholar]
- Henquin J. C. The potassium permeability of pancreatic islet cells: mechanisms of control and influence on insulin release. Horm Metab Res Suppl. 1980;Suppl 10:66–73. [PubMed] [Google Scholar]
- Kohn P. G., Clausen T. The relationship between the transport of glucose and cations across cell membranes in isolated tissues. VI. The effect of insulin, ouabain, and metabolic inhibitors on the transport of 3-O-methylglucose and glucose in rat soleus muscles. Biochim Biophys Acta. 1971 Feb 2;225(2):277–290. doi: 10.1016/0005-2736(71)90221-5. [DOI] [PubMed] [Google Scholar]
- Lauf P. K., McManus T. J., Haas M., Forbush B., 3rd, Duhm J., Flatman P. W., Saier M. H., Jr, Russell J. M. Physiology and biophysics of chloride and cation cotransport across cell membranes. Fed Proc. 1987 May 15;46(7):2377–2394. [PubMed] [Google Scholar]
- Maltin C. A., Harris C. I. Morphological observations and rates of protein synthesis in rat muscles incubated in vitro. Biochem J. 1985 Dec 15;232(3):927–930. doi: 10.1042/bj2320927. [DOI] [PMC free article] [PubMed] [Google Scholar]
- Newgreen D. T., Bray K. M., McHarg A. D., Weston A. H., Duty S., Brown B. S., Kay P. B., Edwards G., Longmore J., Southerton J. S. The action of diazoxide and minoxidil sulphate on rat blood vessels: a comparison with cromakalim. Br J Pharmacol. 1990 Jul;100(3):605–613. doi: 10.1111/j.1476-5381.1990.tb15854.x. [DOI] [PMC free article] [PubMed] [Google Scholar]
- Orlov S. N., Kolosova I. A., Cragoe E. J., Gurlo T. G., Mongin A. A., Aksentsev S. L., Konev S. V. Kinetics and peculiarities of thermal inactivation of volume-induced Na+/H+ exchange, Na+,K+,2Cl- cotransport and K+,Cl- cotransport in rat erythrocytes. Biochim Biophys Acta. 1993 Sep 19;1151(2):186–192. doi: 10.1016/0005-2736(93)90103-7. [DOI] [PubMed] [Google Scholar]
- Panet R., Digregorio D. M., Brown R. H., Jr Irreversible reduction in potassium fluxes accompanies terminal differentiation of human myoblasts to myotubes. J Cell Physiol. 1987 Jul;132(1):57–64. doi: 10.1002/jcp.1041320108. [DOI] [PubMed] [Google Scholar]
- Smith J. M., Sanchez A. A., Jones A. W. Comparison of rubidium-86 and potassium-42 fluxes in rat aorta. Blood Vessels. 1986;23(6):297–309. doi: 10.1159/000158657. [DOI] [PubMed] [Google Scholar]
- Videbaek L. M., Aalkjaer C., Mulvany M. J. Pinacidil opens K+-selective channels causing hyperpolarization and relaxation of noradrenaline contractions in rat mesenteric resistance vessels. Br J Pharmacol. 1988 Sep;95(1):103–108. doi: 10.1111/j.1476-5381.1988.tb16553.x. [DOI] [PMC free article] [PubMed] [Google Scholar]
- Widdicombe J. H. Ouabain-sensitive ion fluxes in the smooth muscle of the guinea-pig's taenia coli. J Physiol. 1977 Apr;266(2):235–254. doi: 10.1113/jphysiol.1977.sp011766. [DOI] [PMC free article] [PubMed] [Google Scholar]