Abstract
In newborn-pig hepatocytes, the rate of oleate oxidation is extremely low, despite a very low malonyl-CoA concentration. By contrast, the sensitivity of carnitine palmitoyltransferase (CPT) I to malonyl-CoA inhibition is high, as suggested by the very low concentration of malonyl-CoA required for 50% inhibition of CPT I (IC50). The rates of oleate oxidation and ketogenesis are respectively 70 and 80% lower in mitochondria isolated from newborn-pig liver than from starved-adult-rat liver mitochondria. Using polarographic measurements, we showed that the oxidation of oleoyl-CoA and palmitoyl-L-carnitine is very low when the acetyl-CoA produced is channelled into the hydroxymethylglutaryl-CoA (HMG-CoA) pathway by addition of malonate. In contrast, the oxidation of the same substrates is high when the acetyl-CoA produced is directed towards the citric acid cycle by addition of malate. We demonstrate that the limitation of ketogenesis in newborn-pig liver is due to a very low amount and activity of mitochondrial HMG-CoA synthase as compared with rat liver mitochondria, and suggest that this could promote the accumulation of acetyl-CoA and/or beta-oxidation products that in turn would decrease the overall rate of fatty acid oxidation in newborn- and adult-pig livers.
Full text
PDF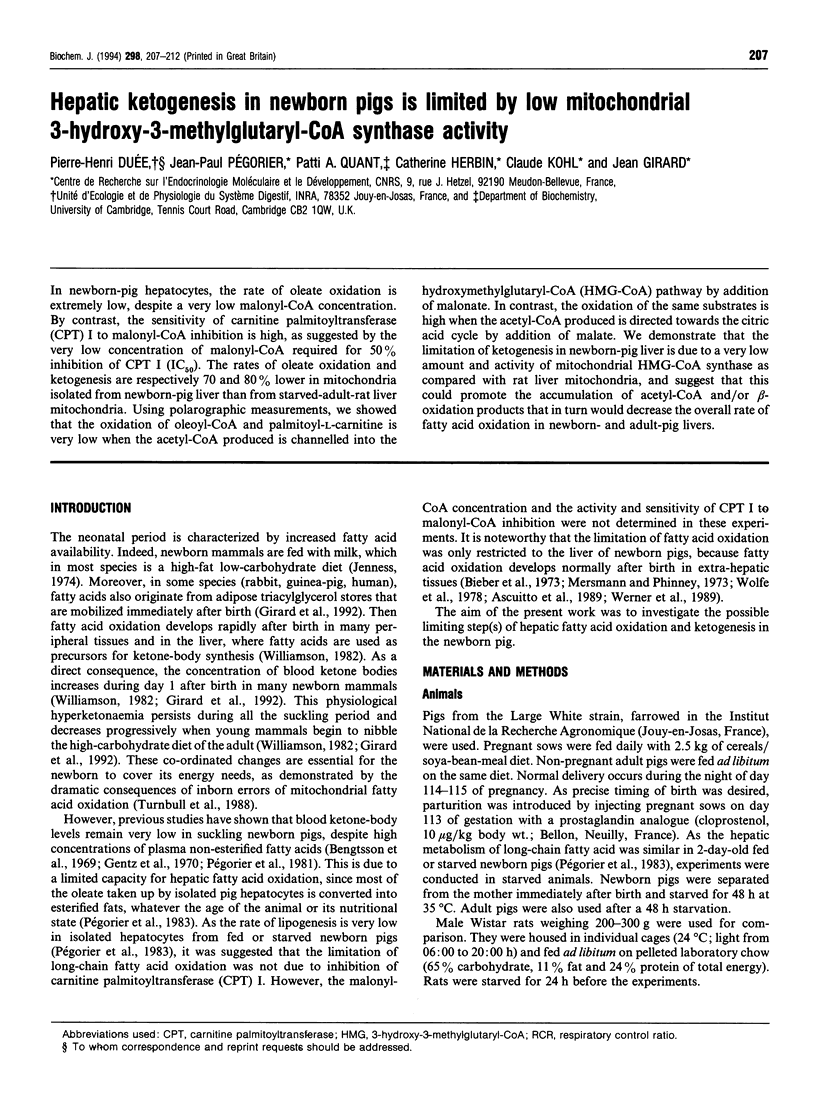
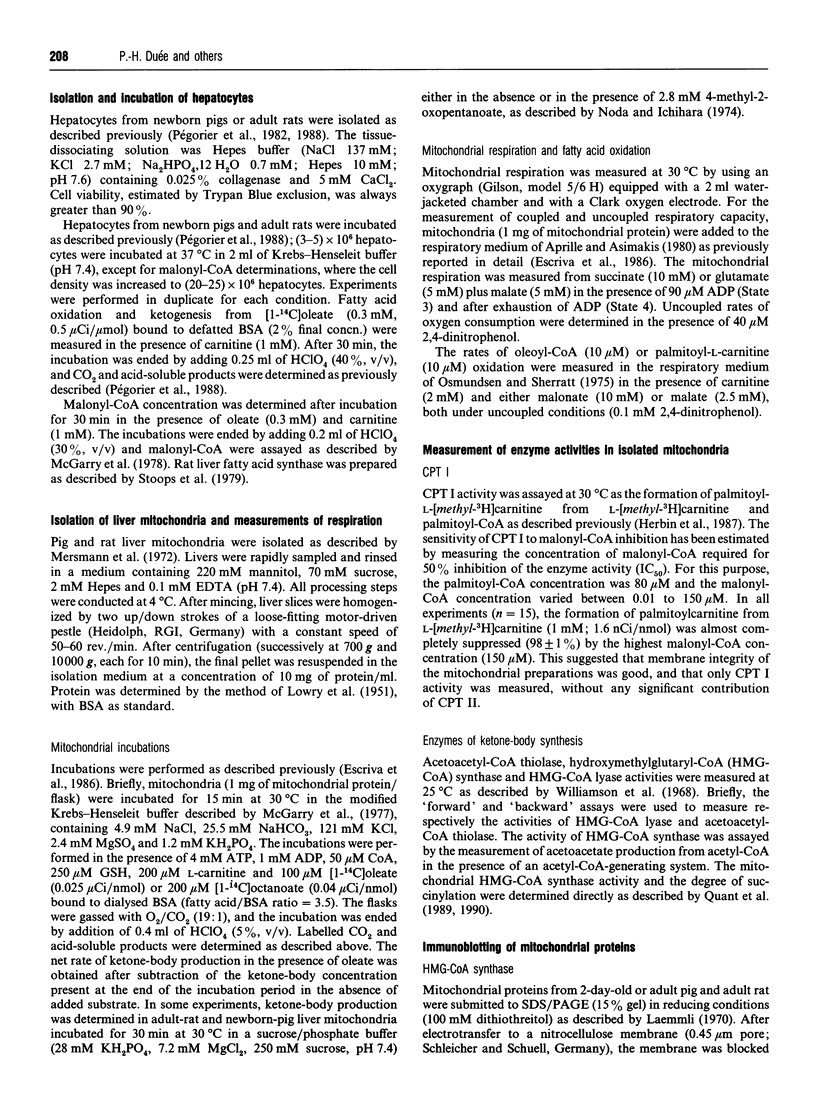
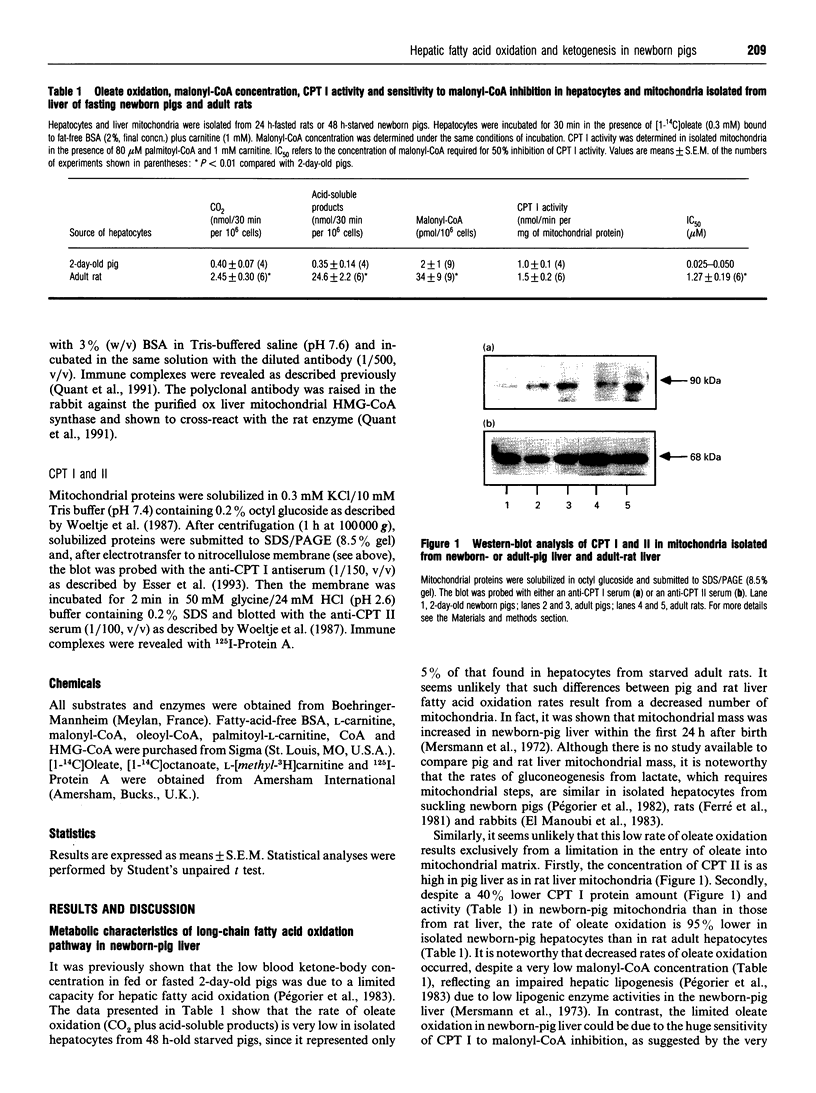
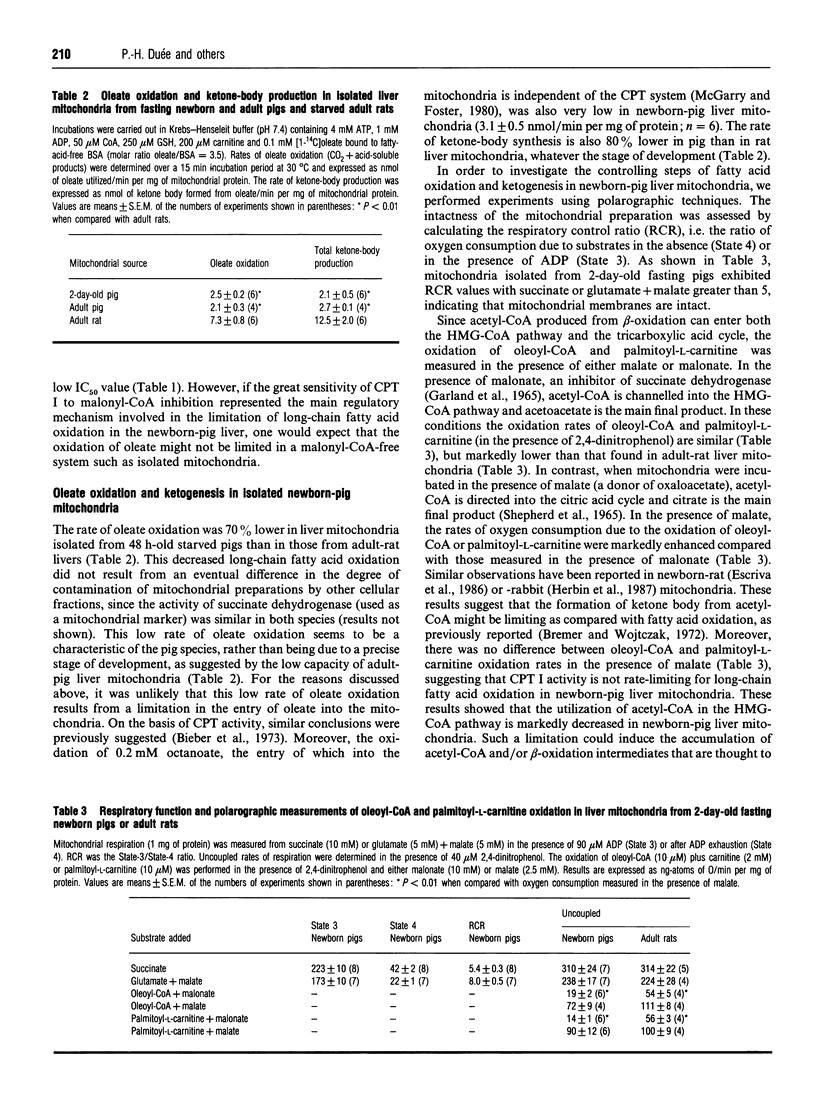
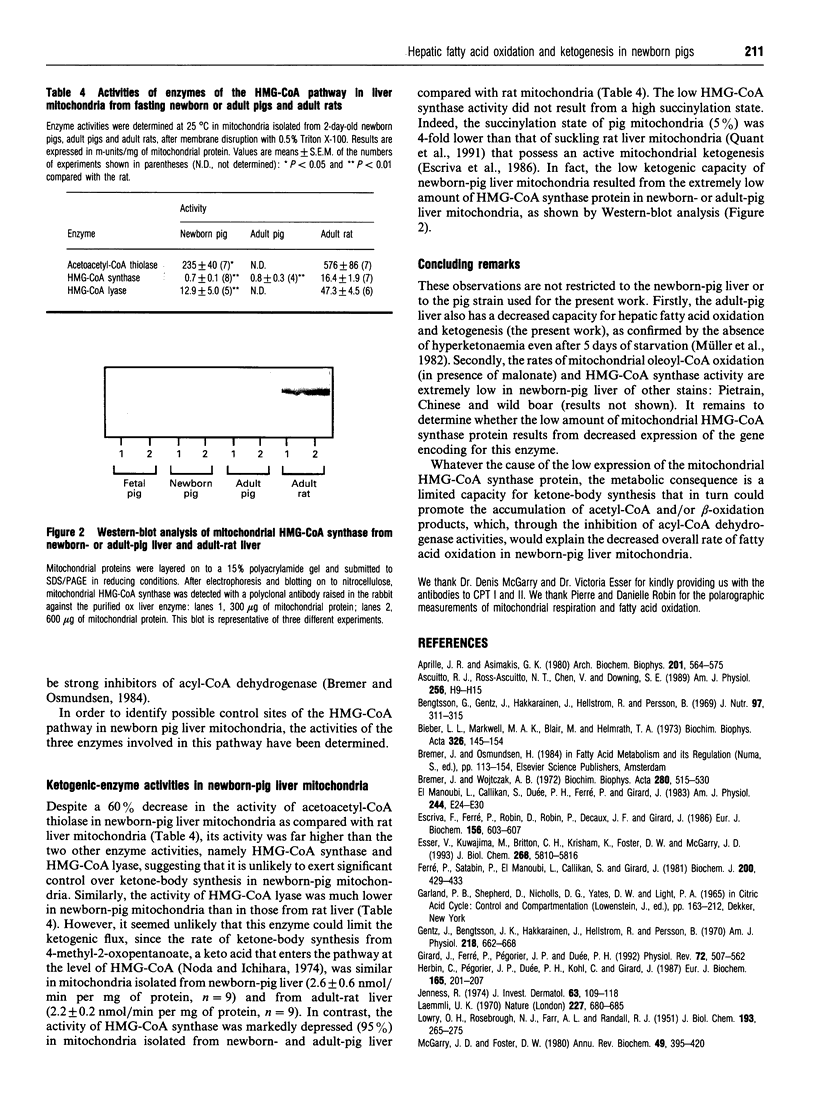
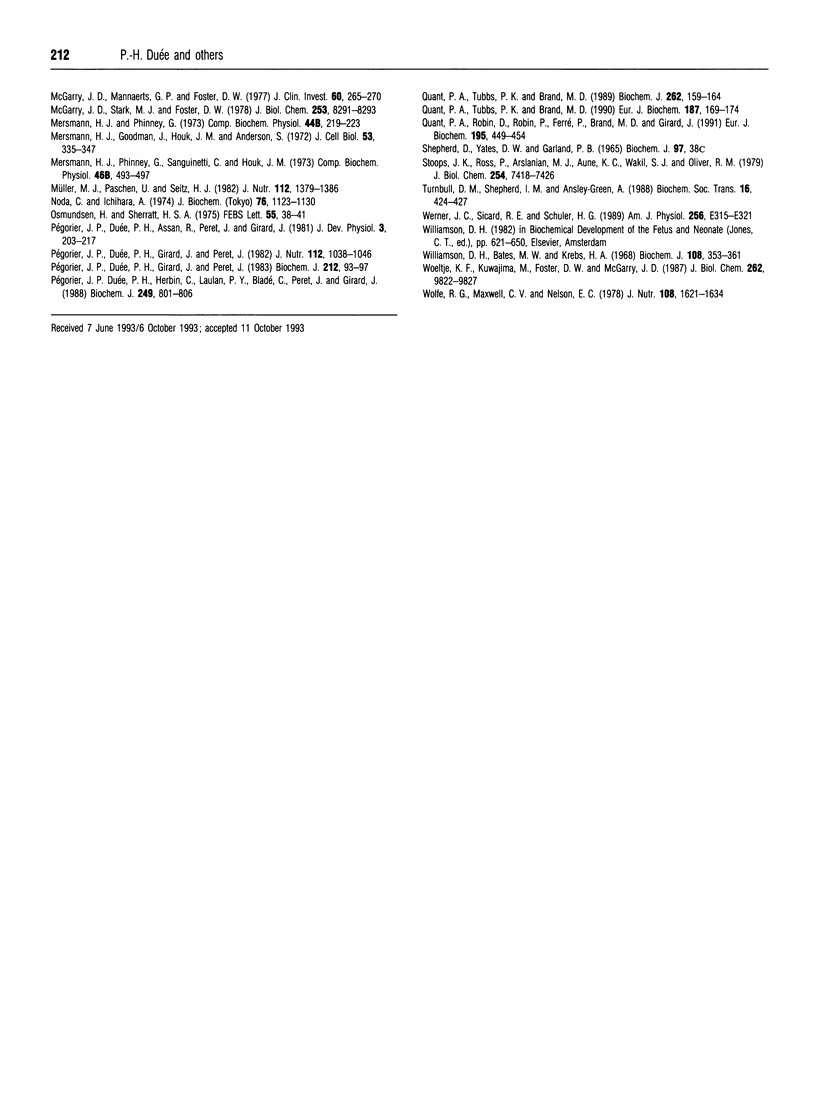
Images in this article
Selected References
These references are in PubMed. This may not be the complete list of references from this article.
- Aprille J. R., Asimakis G. K. Postnatal development of rat liver mitochondria: state 3 respiration, adenine nucleotide translocase activity, and the net accumulation of adenine nucleotides. Arch Biochem Biophys. 1980 May;201(2):564–575. doi: 10.1016/0003-9861(80)90546-9. [DOI] [PubMed] [Google Scholar]
- Ascuitto R. J., Ross-Ascuitto N. T., Chen V., Downing S. E. Ventricular function and fatty acid metabolism in neonatal piglet heart. Am J Physiol. 1989 Jan;256(1 Pt 2):H9–15. doi: 10.1152/ajpheart.1989.256.1.H9. [DOI] [PubMed] [Google Scholar]
- Bengtsson G., Gentz J., Hakkarainen J., Hellström R., Persson B. Plasma levels of FFA, glycerol, beta-hydroxybutyrate and blood glucose during the postnatal development of the pig. J Nutr. 1969 Mar;97(3):311–315. doi: 10.1093/jn/97.3.311. [DOI] [PubMed] [Google Scholar]
- Bieber L. L., Markwell M. A., Blair M., Helmrath T. A. Studies on the development of carnitine palmitoyltransferase and fatty acid oxidation in liver mitochondria of neonatal pigs. Biochim Biophys Acta. 1973 Nov 29;326(2):145–154. doi: 10.1016/0005-2760(73)90240-3. [DOI] [PubMed] [Google Scholar]
- Bremer J., Wojtczak A. B. Factors controlling the rate of fatty acid -oxidation in rat liver mitochondria. Biochim Biophys Acta. 1972 Dec 8;280(4):515–530. doi: 10.1016/0005-2760(72)90131-2. [DOI] [PubMed] [Google Scholar]
- El Manoubi L., Callikan S., Duee P. H., Ferre P., Girard J. Development of gluconeogenesis in isolated hepatocytes from the rabbit. Am J Physiol. 1983 Jan;244(1):E24–E30. doi: 10.1152/ajpendo.1983.244.1.E24. [DOI] [PubMed] [Google Scholar]
- Escriva F., Ferre P., Robin D., Robin P., Decaux J. F., Girard J. Evidence that the development of hepatic fatty acid oxidation at birth in the rat is concomitant with an increased intramitochondrial CoA concentration. Eur J Biochem. 1986 May 2;156(3):603–607. doi: 10.1111/j.1432-1033.1986.tb09620.x. [DOI] [PubMed] [Google Scholar]
- Esser V., Kuwajima M., Britton C. H., Krishnan K., Foster D. W., McGarry J. D. Inhibitors of mitochondrial carnitine palmitoyltransferase I limit the action of proteases on the enzyme. Isolation and partial amino acid analysis of a truncated form of the rat liver isozyme. J Biol Chem. 1993 Mar 15;268(8):5810–5816. [PubMed] [Google Scholar]
- Ferré P., Satabin P., El Manoubi L., Callikan S., Girard J. Relationship between ketogenesis and gluconeogenesis in isolated hepatocytes from newborn rats. Biochem J. 1981 Nov 15;200(2):429–433. doi: 10.1042/bj2000429. [DOI] [PMC free article] [PubMed] [Google Scholar]
- Gentz J., Bengtsson G., Hakkarainen J., Hellström R., Persson B. Metabolic effects of starvation during neonatal period in the piglet. Am J Physiol. 1970 Mar;218(3):662–668. doi: 10.1152/ajplegacy.1970.218.3.662. [DOI] [PubMed] [Google Scholar]
- Girard J., Ferré P., Pégorier J. P., Duée P. H. Adaptations of glucose and fatty acid metabolism during perinatal period and suckling-weaning transition. Physiol Rev. 1992 Apr;72(2):507–562. doi: 10.1152/physrev.1992.72.2.507. [DOI] [PubMed] [Google Scholar]
- Herbin C., Pegorier J. P., Duee P. H., Kohl C., Girard J. Regulation of fatty acid oxidation in isolated hepatocytes and liver mitochondria from newborn rabbits. Eur J Biochem. 1987 May 15;165(1):201–207. doi: 10.1111/j.1432-1033.1987.tb11212.x. [DOI] [PubMed] [Google Scholar]
- Jenness R. Proceedings: Biosynthesis and composition of milk. J Invest Dermatol. 1974 Jul;63(1):109–118. doi: 10.1111/1523-1747.ep12678111. [DOI] [PubMed] [Google Scholar]
- LOWRY O. H., ROSEBROUGH N. J., FARR A. L., RANDALL R. J. Protein measurement with the Folin phenol reagent. J Biol Chem. 1951 Nov;193(1):265–275. [PubMed] [Google Scholar]
- Laemmli U. K. Cleavage of structural proteins during the assembly of the head of bacteriophage T4. Nature. 1970 Aug 15;227(5259):680–685. doi: 10.1038/227680a0. [DOI] [PubMed] [Google Scholar]
- McGarry J. D., Foster D. W. Regulation of hepatic fatty acid oxidation and ketone body production. Annu Rev Biochem. 1980;49:395–420. doi: 10.1146/annurev.bi.49.070180.002143. [DOI] [PubMed] [Google Scholar]
- McGarry J. D., Mannaerts G. P., Foster D. W. A possible role for malonyl-CoA in the regulation of hepatic fatty acid oxidation and ketogenesis. J Clin Invest. 1977 Jul;60(1):265–270. doi: 10.1172/JCI108764. [DOI] [PMC free article] [PubMed] [Google Scholar]
- McGarry J. D., Stark M. J., Foster D. W. Hepatic malonyl-CoA levels of fed, fasted and diabetic rats as measured using a simple radioisotopic assay. J Biol Chem. 1978 Nov 25;253(22):8291–8293. [PubMed] [Google Scholar]
- Mersmann H. J., Goodman J., Houk J. M., Anderson S. Studies on the biochemistry of mitochondria and cell morphology in the neonatal swine hepatocyte. J Cell Biol. 1972 May;53(2):335–347. doi: 10.1083/jcb.53.2.335. [DOI] [PMC free article] [PubMed] [Google Scholar]
- Mersmann H. J., Phinney G. In vitro fatty acid oxidation in liver and heart from neonatal swine (Sus domesticus). Comp Biochem Physiol B. 1973 Jan 15;44(1):219–223. doi: 10.1016/0305-0491(73)90359-3. [DOI] [PubMed] [Google Scholar]
- Mersmann H. J., Phinney G., Sanguinetti M. C., Houk J. M. Lipogenic capacity of liver from perinatal swine (Sus domesticus). Comp Biochem Physiol B. 1973 Nov 15;46(3):493–497. doi: 10.1016/0305-0491(73)90089-8. [DOI] [PubMed] [Google Scholar]
- Müller M. J., Paschen U., Seitz H. J. Starvation-induced ketone body production in the conscious unrestrained miniature pig. J Nutr. 1982 Jul;112(7):1379–1386. doi: 10.1093/jn/112.7.1379. [DOI] [PubMed] [Google Scholar]
- Noda C., Ichihara A. Control of ketogenesis from amino acids. II. Ketone bodies formation from alpha-ketoisocaproate, the keto-analogue of leucine, by rat liver mitochondria. J Biochem. 1974 Nov;76(5):1123–1130. [PubMed] [Google Scholar]
- Osmundsen H., Sherratt H. S. A novel mechanism for inhibition of beta-oxidation by methylenecyclopropylacetyl-CoA, a metabolite of hypoglycin. FEBS Lett. 1975 Jul 15;55(1):38–41. doi: 10.1016/0014-5793(75)80951-3. [DOI] [PubMed] [Google Scholar]
- Pegorier J. P., Duee P. H., Girard J., Peret J. Development of gluconeogenesis in isolated hepatocytes from fasting or suckling newborn pigs. J Nutr. 1982 Jun;112(6):1038–1046. doi: 10.1093/jn/112.6.1038. [DOI] [PubMed] [Google Scholar]
- Pégorier J. P., Duée P. H., Assan R., Peret J., Girard J. Changes in circulating fuels, pancreatic hormones and liver glycogen concentration in fasting or suckling newborn pigs. J Dev Physiol. 1981 Jun;3(3):203–217. [PubMed] [Google Scholar]
- Pégorier J. P., Duée P. H., Girard J., Peret J. Metabolic fate of non-esterified fatty acids in isolated hepatocytes from newborn and young pigs. Evidence for a limited capacity for oxidation and increased capacity for esterification. Biochem J. 1983 Apr 15;212(1):93–97. doi: 10.1042/bj2120093. [DOI] [PMC free article] [PubMed] [Google Scholar]
- Pégorier J. P., Duée P. H., Herbin C., Laulan P. Y., Bladé C., Peret J., Girard J. Fatty acid metabolism in hepatocytes isolated from rats adapted to high-fat diets containing long- or medium-chain triacylglycerols. Biochem J. 1988 Feb 1;249(3):801–806. doi: 10.1042/bj2490801. [DOI] [PMC free article] [PubMed] [Google Scholar]
- Quant P. A., Robin D., Robin P., Ferre P., Brand M. D., Girard J. Control of hepatic mitochondrial 3-hydroxy-3-methylglutaryl-CoA synthase during the foetal/neonatal transition, suckling and weaning in the rat. Eur J Biochem. 1991 Jan 30;195(2):449–454. doi: 10.1111/j.1432-1033.1991.tb15724.x. [DOI] [PubMed] [Google Scholar]
- Quant P. A., Tubbs P. K., Brand M. D. Glucagon activates mitochondrial 3-hydroxy-3-methylglutaryl-CoA synthase in vivo by decreasing the extent of succinylation of the enzyme. Eur J Biochem. 1990 Jan 12;187(1):169–174. doi: 10.1111/j.1432-1033.1990.tb15291.x. [DOI] [PubMed] [Google Scholar]
- Quant P. A., Tubbs P. K., Brand M. D. Treatment of rats with glucagon or mannoheptulose increases mitochondrial 3-hydroxy-3-methylglutaryl-CoA synthase activity and decreases succinyl-CoA content in liver. Biochem J. 1989 Aug 15;262(1):159–164. doi: 10.1042/bj2620159. [DOI] [PMC free article] [PubMed] [Google Scholar]
- Stoops J. K., Ross P., Arslanian M. J., Aune K. C., Wakil S. J., Oliver R. M. Physicochemical studies of the rat liver and adipose fatty acid synthetases. J Biol Chem. 1979 Aug 10;254(15):7418–7426. [PubMed] [Google Scholar]
- Turnbull D. M., Shepherd I. M., Aynsley-Green A. Inherited defects of mitochondrial fatty acid oxidation. Biochem Soc Trans. 1988 Jun;16(3):424–427. doi: 10.1042/bst0160424. [DOI] [PubMed] [Google Scholar]
- Werner J. C., Sicard R. E., Schuler H. G. Palmitate oxidation by isolated working fetal and newborn pig hearts. Am J Physiol. 1989 Feb;256(2 Pt 1):E315–E321. doi: 10.1152/ajpendo.1989.256.2.E315. [DOI] [PubMed] [Google Scholar]
- Williamson D. H., Bates M. W., Krebs H. A. Activity and intracellular distribution of enzymes of ketone-body metabolism in rat liver. Biochem J. 1968 Jul;108(3):353–361. doi: 10.1042/bj1080353. [DOI] [PMC free article] [PubMed] [Google Scholar]
- Woeltje K. F., Kuwajima M., Foster D. W., McGarry J. D. Characterization of the mitochondrial carnitine palmitoyltransferase enzyme system. II. Use of detergents and antibodies. J Biol Chem. 1987 Jul 15;262(20):9822–9827. [PubMed] [Google Scholar]
- Wolfe R. G., Maxwell C. V., Nelson E. C. Effect of age and dietary fat level on fatty acid oxidation in the neonatal pig. J Nutr. 1978 Oct;108(10):1621–1634. doi: 10.1093/jn/108.10.1621. [DOI] [PubMed] [Google Scholar]