Abstract
Several enzymes require thiamin diphosphate (ThDP) as an essential cofactor, and we have used one of these, pyruvate decarboxylase (PDC; EC 4.1.1.1) from Zymomonas mobilis, as a model for this group of enzymes. It is well suited for this purpose because of its stability, ease of purification and its simple kinetic properties. A sequence motif of approx. 30 residues, beginning with a glycine-aspartate-glycine (-GDG-) triplet and ending with a double asparagine (-NN-) sequence, has been identified in many of these enzymes [Hawkins, Borges and Perham (1989) FEBS Lett. 255, 77-82]. Other residues within this putative ThDP-binding motif are conserved, but to a lesser extent, including a glutamate and a proline residue. The role of the elements of this motif has been clarified by the determination of the three-dimensional structure of three of these enzymes [Muller, Lindqvist, Furey, Schulz, Jordan and Schneider (1993) Structure 1, 95-103]. Four of the residues within this motif were modified by site-directed mutagenesis of the cloned PDC gene to evaluate their role in cofactor binding. The mutant proteins were expressed in Escherichia coli and found to purify normally, indicating that the tertiary structure of these enzymes had not been grossly perturbed by the amino acid substitutions. We have shown previously [Diefenbach, Candy, Mattick and Duggleby (1992) FEBS Lett. 296, 95-98] that changing the aspartate in the -GDG- sequence to glycine, threonine or asparagine yields an inactive enzyme that is unable to bind ThDP, therefore verifying the role of the ThDP-binding motif. Here we demonstrate that substitution with glutamate yields an active enzyme with a greatly reduced affinity for both ThDP and Mg2+, but with normal kinetics for pyruvate. Unlike the wild-type tetrameric enzyme, this mutant protein usually exists as a dimer. Replacement of the second asparagine of the -NN- sequence by glutamine also yields an inactive enzyme which is unable to bind ThDP, whereas replacement with an aspartate residue results in an active enzyme with a reduced affinity for ThDP but which displays normal kinetics for both Mg2+ and pyruvate. Replacing the conserved glutamate with aspartate did not alter the properties of the enzyme, while the conserved proline, thought to be required for structural reasons, could be substituted with glycine or alanine without inactivating the enzyme, but these changes did reduce its stability.
Full text
PDF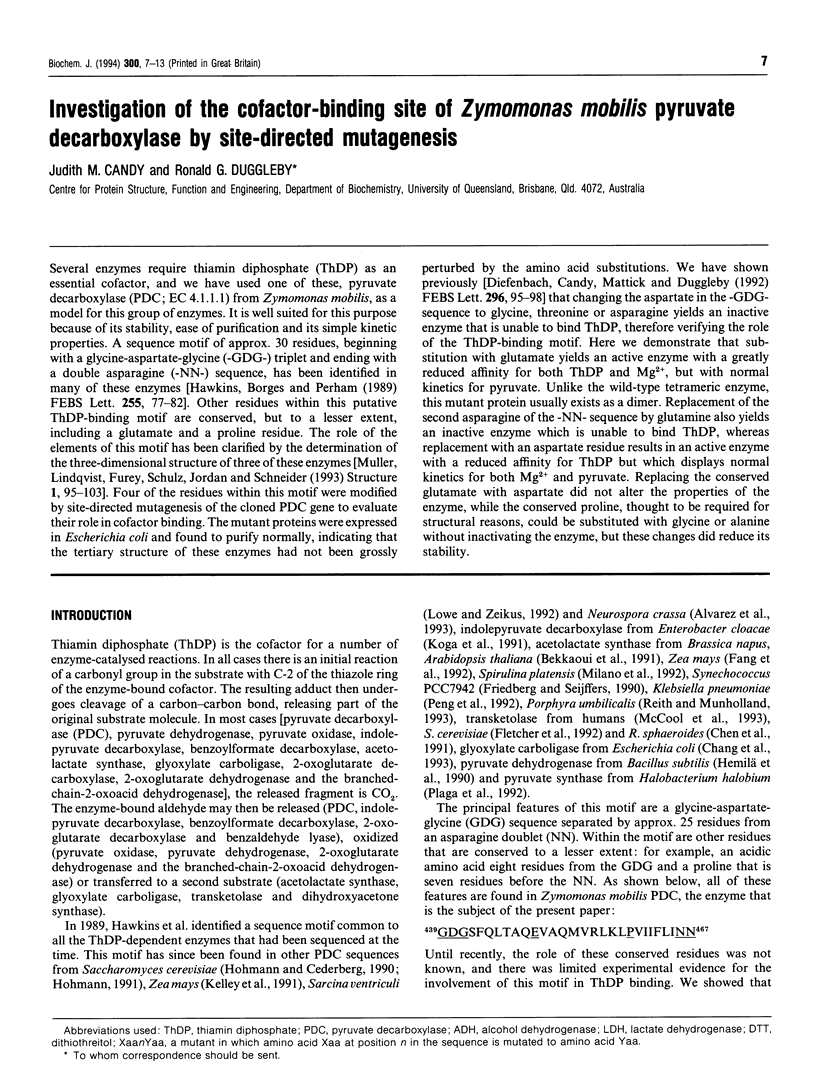
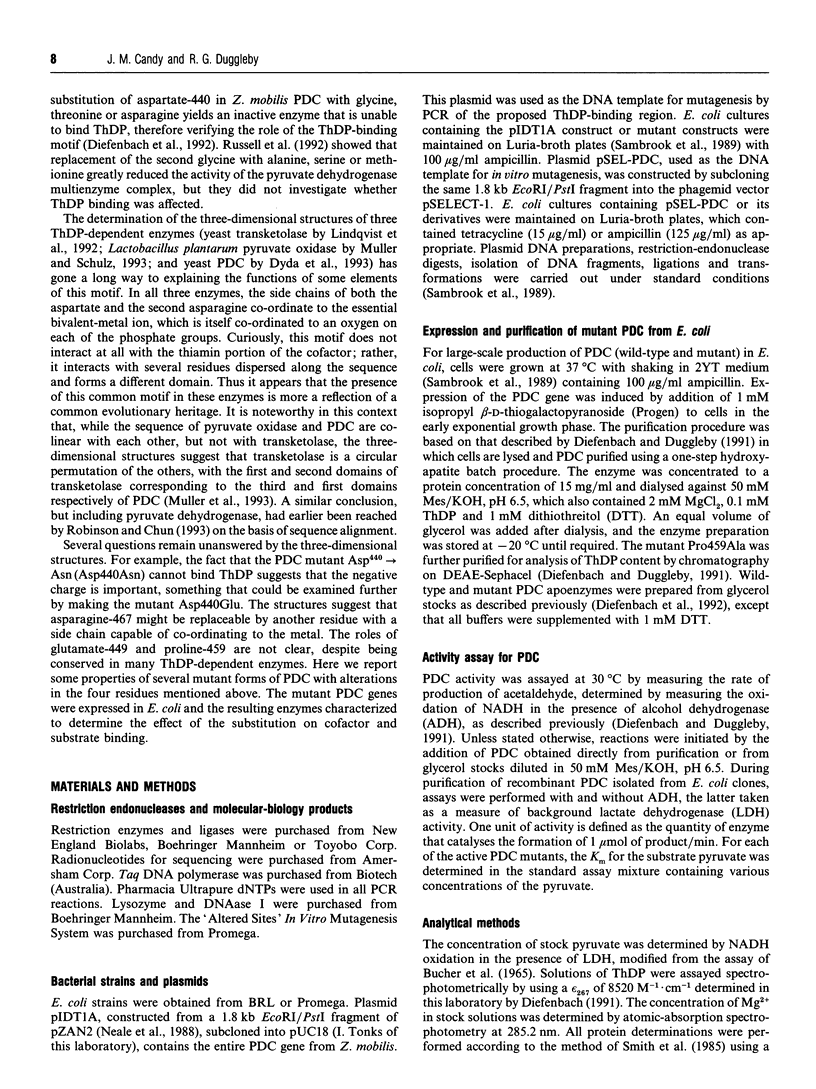
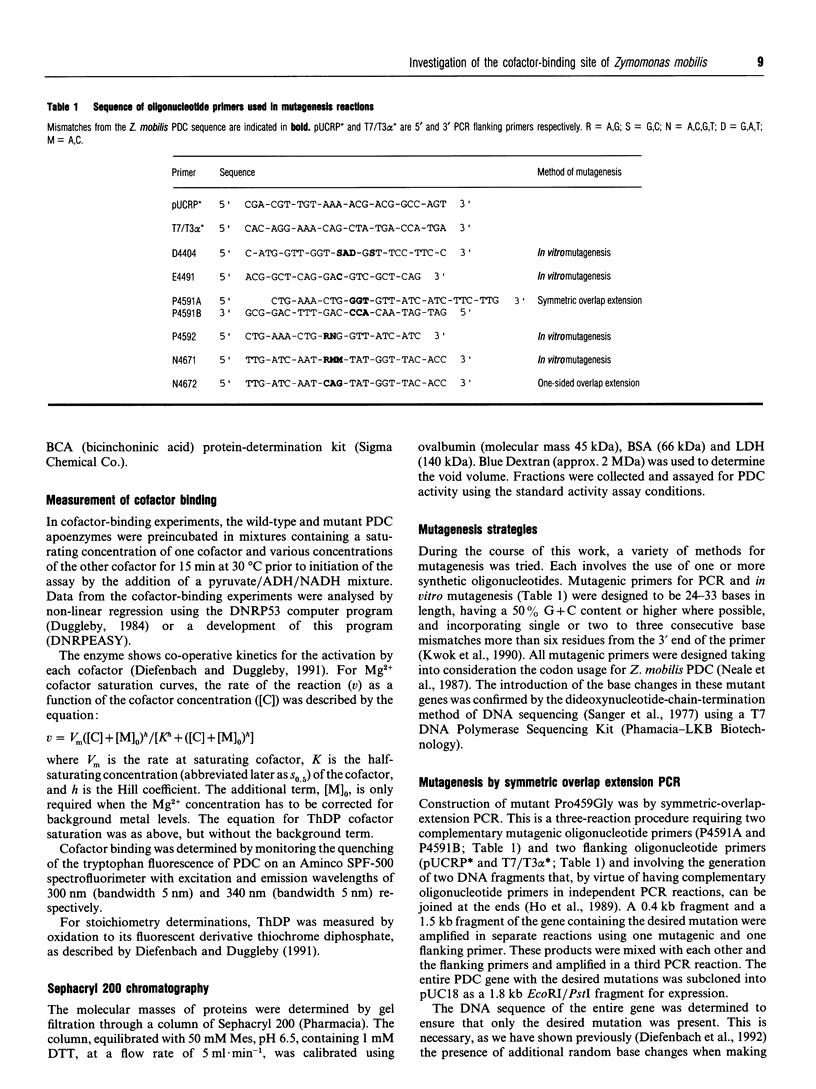
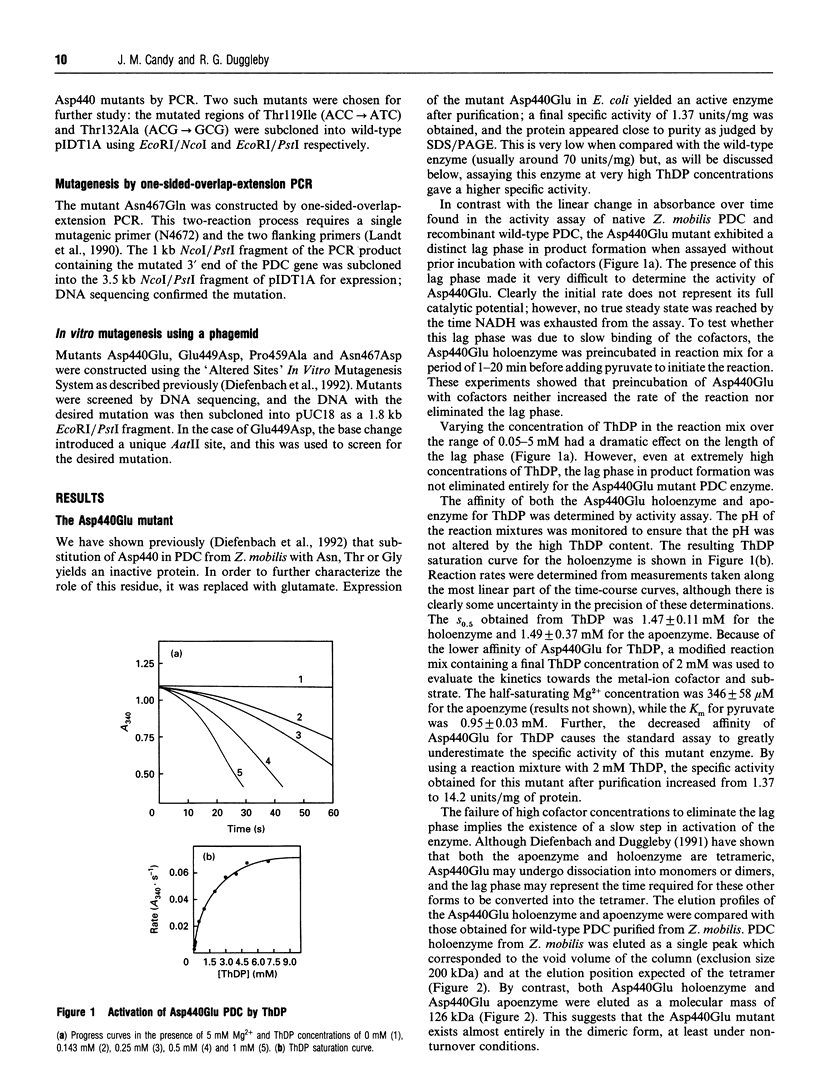
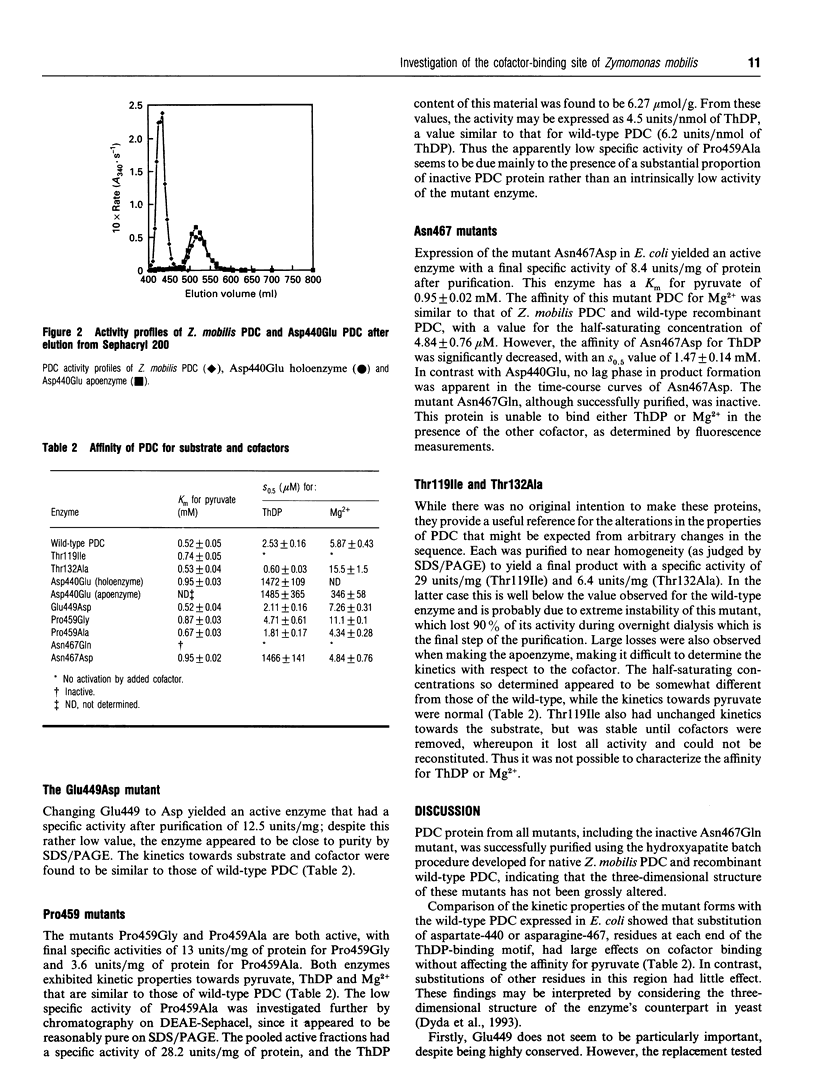
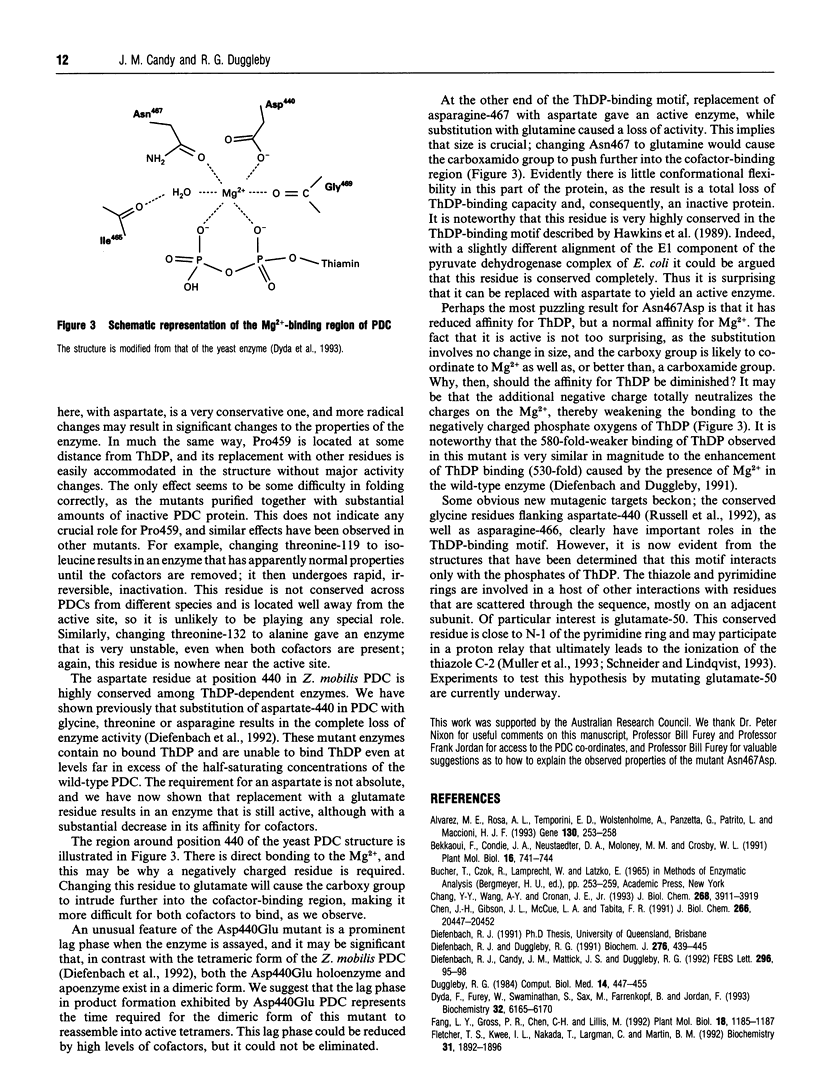
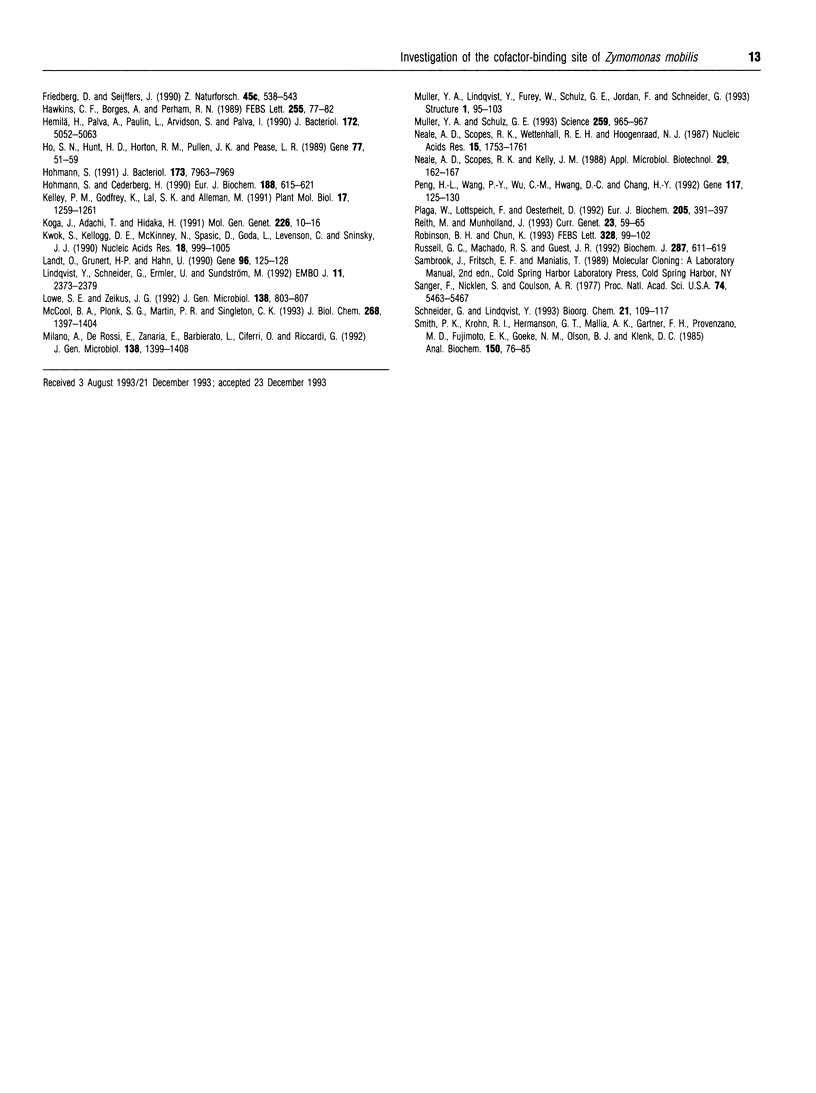
Selected References
These references are in PubMed. This may not be the complete list of references from this article.
- Alvarez M. E., Rosa A. L., Temporini E. D., Wolstenholme A., Panzetta G., Patrito L., Maccioni H. J. The 59-kDa polypeptide constituent of 8-10-nm cytoplasmic filaments in Neurospora crassa is a pyruvate decarboxylase. Gene. 1993 Aug 25;130(2):253–258. doi: 10.1016/0378-1119(93)90427-5. [DOI] [PubMed] [Google Scholar]
- Bekkaoui F., Condie J. A., Neustaedter D. A., Moloney M. M., Crosby W. L. Isolation, structure and expression of a cDNA for acetolactate synthase from Brassica napus. Plant Mol Biol. 1991 Apr;16(4):741–744. doi: 10.1007/BF00023440. [DOI] [PubMed] [Google Scholar]
- Chang Y. Y., Wang A. Y., Cronan J. E., Jr Molecular cloning, DNA sequencing, and biochemical analyses of Escherichia coli glyoxylate carboligase. An enzyme of the acetohydroxy acid synthase-pyruvate oxidase family. J Biol Chem. 1993 Feb 25;268(6):3911–3919. [PubMed] [Google Scholar]
- Chen J. H., Gibson J. L., McCue L. A., Tabita F. R. Identification, expression, and deduced primary structure of transketolase and other enzymes encoded within the form II CO2 fixation operon of Rhodobacter sphaeroides. J Biol Chem. 1991 Oct 25;266(30):20447–20452. [PubMed] [Google Scholar]
- Diefenbach R. J., Candy J. M., Mattick J. S., Duggleby R. G. Effects of substitution of aspartate-440 and tryptophan-487 in the thiamin diphosphate binding region of pyruvate decarboxylase from Zymomonas mobilis. FEBS Lett. 1992 Jan 13;296(1):95–98. doi: 10.1016/0014-5793(92)80411-9. [DOI] [PubMed] [Google Scholar]
- Diefenbach R. J., Duggleby R. G. Pyruvate decarboxylase from Zymomonas mobilis. Structure and re-activation of apoenzyme by the cofactors thiamin diphosphate and magnesium ion. Biochem J. 1991 Jun 1;276(Pt 2):439–445. doi: 10.1042/bj2760439. [DOI] [PMC free article] [PubMed] [Google Scholar]
- Duggleby R. G. Regression analysis of nonlinear Arrhenius plots: an empirical model and a computer program. Comput Biol Med. 1984;14(4):447–455. doi: 10.1016/0010-4825(84)90045-3. [DOI] [PubMed] [Google Scholar]
- Dyda F., Furey W., Swaminathan S., Sax M., Farrenkopf B., Jordan F. Catalytic centers in the thiamin diphosphate dependent enzyme pyruvate decarboxylase at 2.4-A resolution. Biochemistry. 1993 Jun 22;32(24):6165–6170. doi: 10.1021/bi00075a008. [DOI] [PubMed] [Google Scholar]
- Fang L. Y., Gross P. R., Chen C. H., Lillis M. Sequence of two acetohydroxyacid synthase genes from Zea mays. Plant Mol Biol. 1992 Apr;18(6):1185–1187. doi: 10.1007/BF00047723. [DOI] [PubMed] [Google Scholar]
- Fletcher T. S., Kwee I. L., Nakada T., Largman C., Martin B. M. DNA sequence of the yeast transketolase gene. Biochemistry. 1992 Feb 18;31(6):1892–1896. doi: 10.1021/bi00121a044. [DOI] [PubMed] [Google Scholar]
- Friedberg D., Seijffers J. Molecular characterization of genes coding for wild-type and sulfonylurea-resistant acetolactate synthase in the cyanobacterium Synechococcus PCC7942. Z Naturforsch C. 1990 May;45(5):538–543. doi: 10.1515/znc-1990-0541. [DOI] [PubMed] [Google Scholar]
- Hawkins C. F., Borges A., Perham R. N. A common structural motif in thiamin pyrophosphate-binding enzymes. FEBS Lett. 1989 Sep 11;255(1):77–82. doi: 10.1016/0014-5793(89)81064-6. [DOI] [PubMed] [Google Scholar]
- Hemilä H., Palva A., Paulin L., Arvidson S., Palva I. Secretory S complex of Bacillus subtilis: sequence analysis and identity to pyruvate dehydrogenase. J Bacteriol. 1990 Sep;172(9):5052–5063. doi: 10.1128/jb.172.9.5052-5063.1990. [DOI] [PMC free article] [PubMed] [Google Scholar]
- Ho S. N., Hunt H. D., Horton R. M., Pullen J. K., Pease L. R. Site-directed mutagenesis by overlap extension using the polymerase chain reaction. Gene. 1989 Apr 15;77(1):51–59. doi: 10.1016/0378-1119(89)90358-2. [DOI] [PubMed] [Google Scholar]
- Hohmann S., Cederberg H. Autoregulation may control the expression of yeast pyruvate decarboxylase structural genes PDC1 and PDC5. Eur J Biochem. 1990 Mar 30;188(3):615–621. doi: 10.1111/j.1432-1033.1990.tb15442.x. [DOI] [PubMed] [Google Scholar]
- Hohmann S. Characterization of PDC6, a third structural gene for pyruvate decarboxylase in Saccharomyces cerevisiae. J Bacteriol. 1991 Dec;173(24):7963–7969. doi: 10.1128/jb.173.24.7963-7969.1991. [DOI] [PMC free article] [PubMed] [Google Scholar]
- Kelley P. M., Godfrey K., Lal S. K., Alleman M. Characterization of the maize pyruvate decarboxylase gene. Plant Mol Biol. 1991 Dec;17(6):1259–1261. doi: 10.1007/BF00028743. [DOI] [PubMed] [Google Scholar]
- Koga J., Adachi T., Hidaka H. Molecular cloning of the gene for indolepyruvate decarboxylase from Enterobacter cloacae. Mol Gen Genet. 1991 Apr;226(1-2):10–16. doi: 10.1007/BF00273581. [DOI] [PubMed] [Google Scholar]
- Kwok S., Kellogg D. E., McKinney N., Spasic D., Goda L., Levenson C., Sninsky J. J. Effects of primer-template mismatches on the polymerase chain reaction: human immunodeficiency virus type 1 model studies. Nucleic Acids Res. 1990 Feb 25;18(4):999–1005. doi: 10.1093/nar/18.4.999. [DOI] [PMC free article] [PubMed] [Google Scholar]
- Landt O., Grunert H. P., Hahn U. A general method for rapid site-directed mutagenesis using the polymerase chain reaction. Gene. 1990 Nov 30;96(1):125–128. doi: 10.1016/0378-1119(90)90351-q. [DOI] [PubMed] [Google Scholar]
- Lindqvist Y., Schneider G., Ermler U., Sundström M. Three-dimensional structure of transketolase, a thiamine diphosphate dependent enzyme, at 2.5 A resolution. EMBO J. 1992 Jul;11(7):2373–2379. doi: 10.1002/j.1460-2075.1992.tb05301.x. [DOI] [PMC free article] [PubMed] [Google Scholar]
- Lowe S. E., Zeikus J. G. Purification and characterization of pyruvate decarboxylase from Sarcina ventriculi. J Gen Microbiol. 1992 Apr;138(4):803–807. doi: 10.1099/00221287-138-4-803. [DOI] [PubMed] [Google Scholar]
- McCool B. A., Plonk S. G., Martin P. R., Singleton C. K. Cloning of human transketolase cDNAs and comparison of the nucleotide sequence of the coding region in Wernicke-Korsakoff and non-Wernicke-Korsakoff individuals. J Biol Chem. 1993 Jan 15;268(2):1397–1404. [PubMed] [Google Scholar]
- Milano A., De Rossi E., Zanaria E., Barbierato L., Ciferri O., Riccardi G. Molecular characterization of the genes encoding acetohydroxy acid synthase in the cyanobacterium Spirulina platensis. J Gen Microbiol. 1992 Jul;138(7):1399–1408. doi: 10.1099/00221287-138-7-1399. [DOI] [PubMed] [Google Scholar]
- Muller Y. A., Lindqvist Y., Furey W., Schulz G. E., Jordan F., Schneider G. A thiamin diphosphate binding fold revealed by comparison of the crystal structures of transketolase, pyruvate oxidase and pyruvate decarboxylase. Structure. 1993 Oct 15;1(2):95–103. doi: 10.1016/0969-2126(93)90025-c. [DOI] [PubMed] [Google Scholar]
- Muller Y. A., Schulz G. E. Structure of the thiamine- and flavin-dependent enzyme pyruvate oxidase. Science. 1993 Feb 12;259(5097):965–967. doi: 10.1126/science.8438155. [DOI] [PubMed] [Google Scholar]
- Neale A. D., Scopes R. K., Wettenhall R. E., Hoogenraad N. J. Nucleotide sequence of the pyruvate decarboxylase gene from Zymomonas mobilis. Nucleic Acids Res. 1987 Feb 25;15(4):1753–1761. doi: 10.1093/nar/15.4.1753. [DOI] [PMC free article] [PubMed] [Google Scholar]
- Peng H. L., Wang P. Y., Wu C. M., Hwang D. C., Chang H. Y. Cloning, sequencing and heterologous expression of a Klebsiella pneumoniae gene encoding an FAD-independent acetolactate synthase. Gene. 1992 Aug 1;117(1):125–130. doi: 10.1016/0378-1119(92)90500-o. [DOI] [PubMed] [Google Scholar]
- Plaga W., Lottspeich F., Oesterhelt D. Improved purification, crystallization and primary structure of pyruvate:ferredoxin oxidoreductase from Halobacterium halobium. Eur J Biochem. 1992 Apr 1;205(1):391–397. doi: 10.1111/j.1432-1033.1992.tb16792.x. [DOI] [PubMed] [Google Scholar]
- Reith M., Munholland J. Two amino-acid biosynthetic genes are encoded on the plastid genome of the red alga Porphyra umbilicalis. Curr Genet. 1993 Jan;23(1):59–65. doi: 10.1007/BF00336751. [DOI] [PubMed] [Google Scholar]
- Robinson B. H., Chun K. The relationships between transketolase, yeast pyruvate decarboxylase and pyruvate dehydrogenase of the pyruvate dehydrogenase complex. FEBS Lett. 1993 Aug 9;328(1-2):99–102. doi: 10.1016/0014-5793(93)80973-x. [DOI] [PubMed] [Google Scholar]
- Russell G. C., Machado R. S., Guest J. R. Overproduction of the pyruvate dehydrogenase multienzyme complex of Escherichia coli and site-directed substitutions in the E1p and E2p subunits. Biochem J. 1992 Oct 15;287(Pt 2):611–619. doi: 10.1042/bj2870611. [DOI] [PMC free article] [PubMed] [Google Scholar]
- Sanger F., Nicklen S., Coulson A. R. DNA sequencing with chain-terminating inhibitors. Proc Natl Acad Sci U S A. 1977 Dec;74(12):5463–5467. doi: 10.1073/pnas.74.12.5463. [DOI] [PMC free article] [PubMed] [Google Scholar]
- Smith P. K., Krohn R. I., Hermanson G. T., Mallia A. K., Gartner F. H., Provenzano M. D., Fujimoto E. K., Goeke N. M., Olson B. J., Klenk D. C. Measurement of protein using bicinchoninic acid. Anal Biochem. 1985 Oct;150(1):76–85. doi: 10.1016/0003-2697(85)90442-7. [DOI] [PubMed] [Google Scholar]