Abstract
The quinonoid anthracycline, doxorubicin (Adriamycin) is a potent anti-neoplastic agent whose clinical use is limited by severe cardiotoxicity. Mitochondrial damage is a major component of this cardiotoxicity, and rival oxidative and non-oxidative mechanisms for inactivation of the electron transport chain have been proposed. Using bovine heart submitochondrial preparations (SMP) we have now found that both oxidative and non-oxidative mechanisms occur in vitro, depending solely on the concentration of doxorubicin employed. Redox cycling of doxorubicin by Complex I of the respiratory chain (which generates doxorubicin semiquinone radicals, O2-, H2O2, and .OH) caused a 70% decrease in the Vmax. for NADH dehydrogenase during 15 min incubation of SMP, and an 80% decrease in NADH oxidase activity after 2 h incubation. This inactivation required only 25-50 microM-doxorubicin and represents true oxidative damage, since both NADH (for doxorubicin redox cycling) and oxygen were obligatory participants. The damage appears localized between the NADH dehydrogenase flavin (site of doxorubicin reduction) and iron-sulphur centre N-1. Succinate dehydrogenase, succinate oxidase, and cytochrome c oxidase activities were strongly inhibited by higher doxorubicin concentrations, but this phenomenon did not involve doxorubicin redox cycling (no NADH or oxygen requirement). Doxorubicin concentrations of 0.5 mM were required for 50% decreases in these activities, except for cytochrome c oxidase which was only 30% inhibited following incubation with even 1.0 mM-doxorubicin. Our results indicate that low concentrations of doxorubicin (50 microM or less) can catalyse a site-specific oxidative damage to the NADH oxidation pathway. In contrast, ten-fold higher doxorubicin concentrations (or more) are required for non-oxidative inactivation of the electron transport chain; probably via binding to cardiolipin and/or generalized membrane chaotropic effects. The development of agents to block doxorubicin toxicity in vivo will clearly require detailed clinical studies of doxorubicin uptake in the heart.
Full text
PDF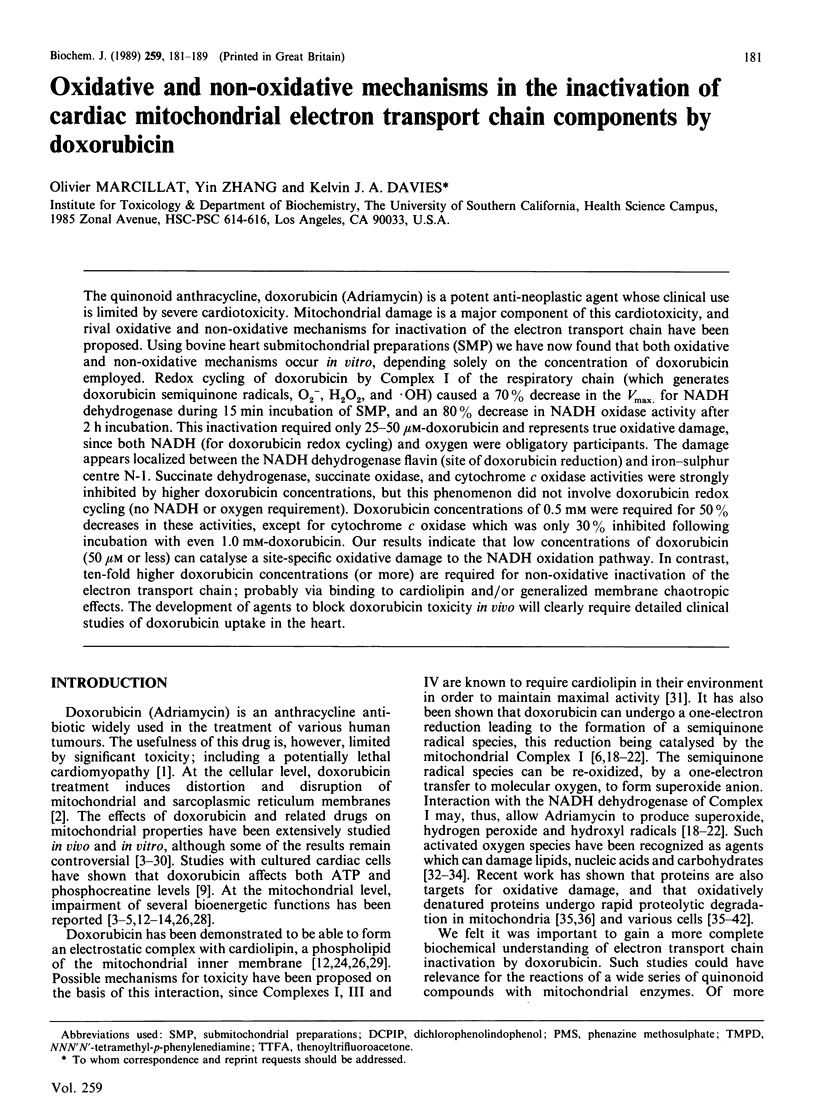
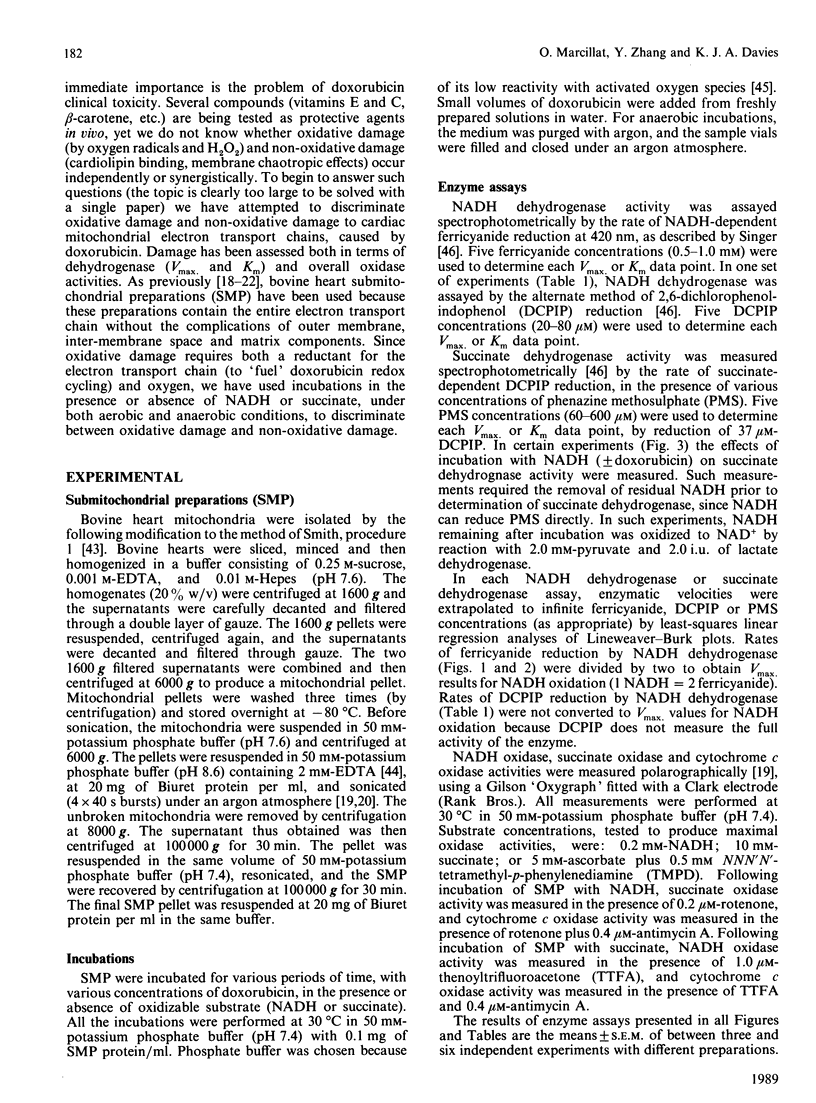
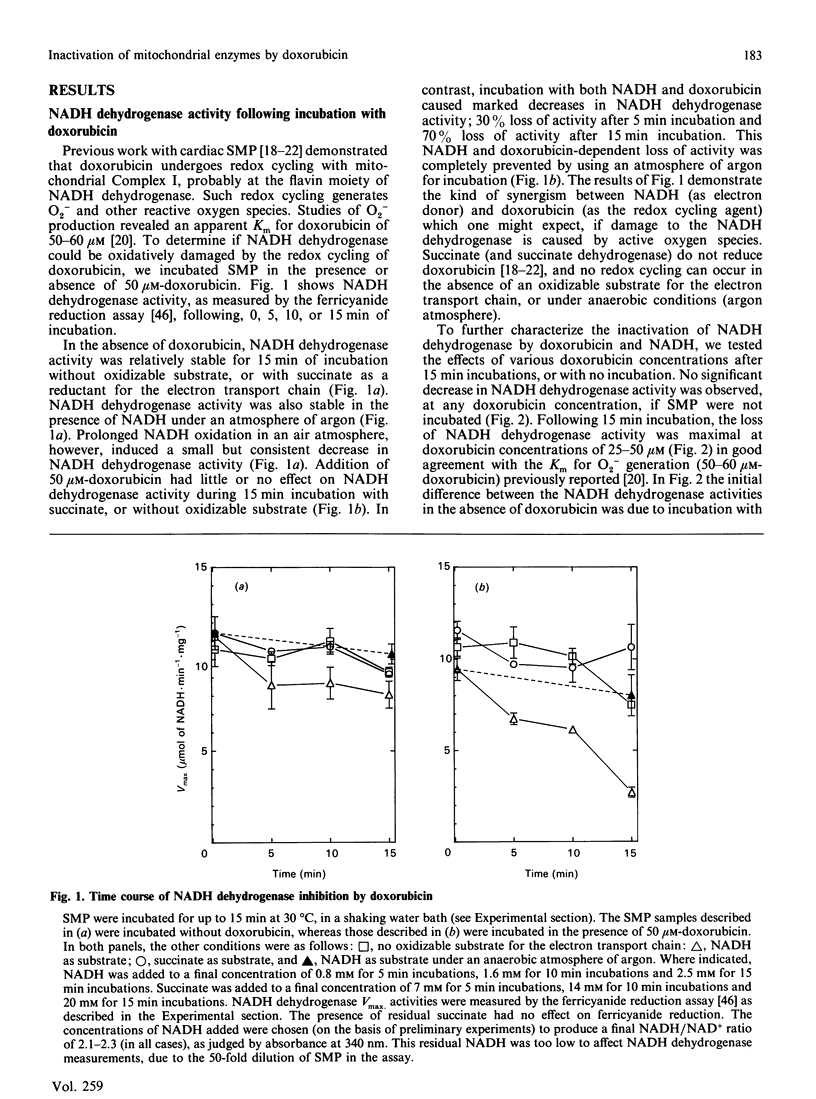
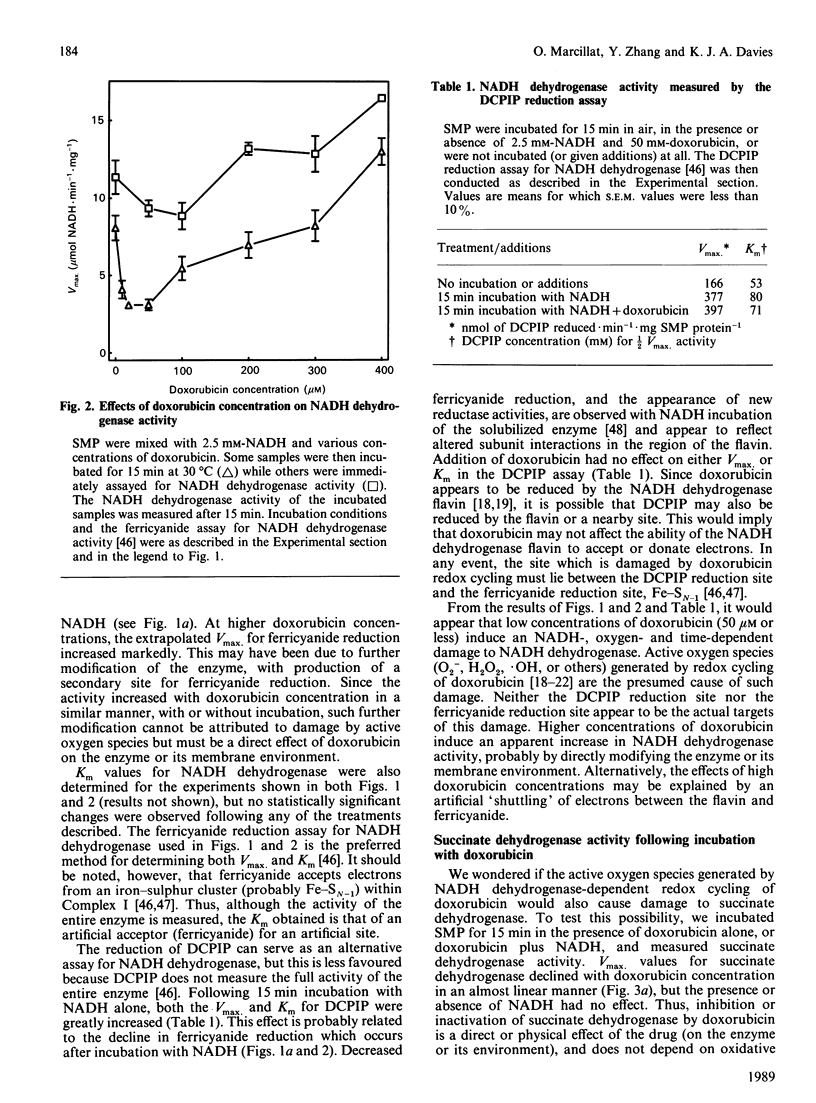
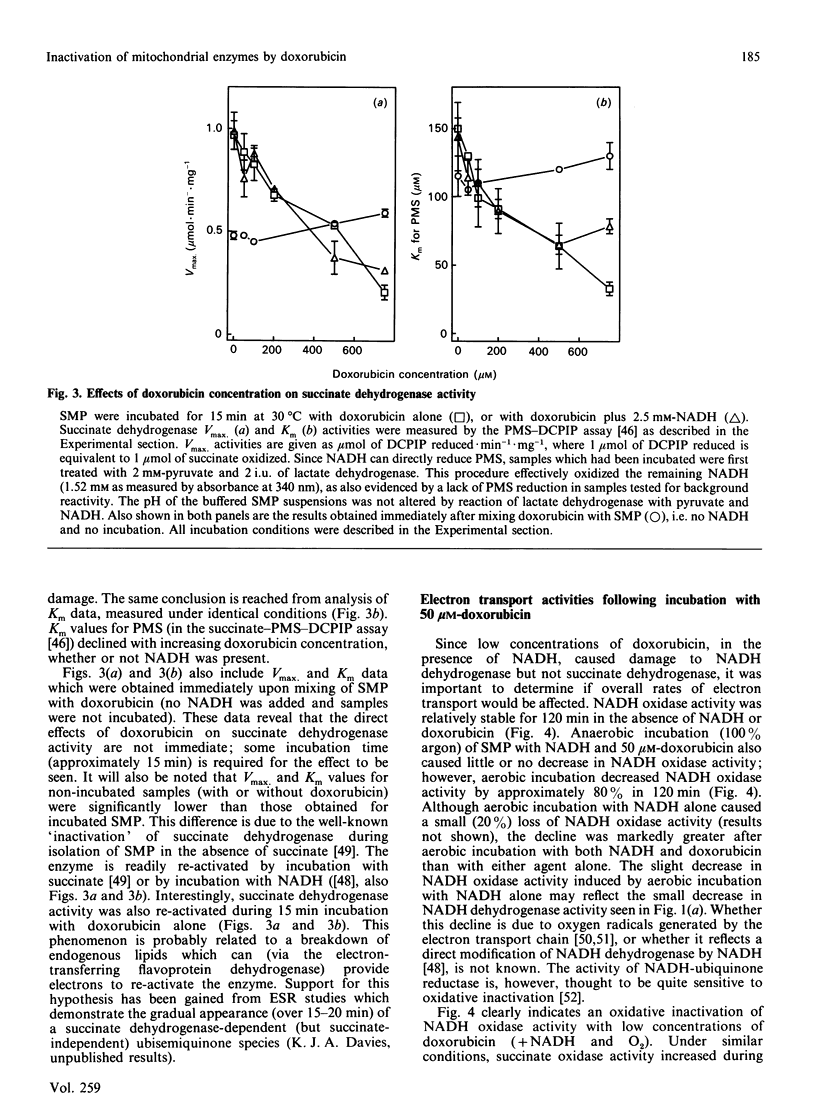
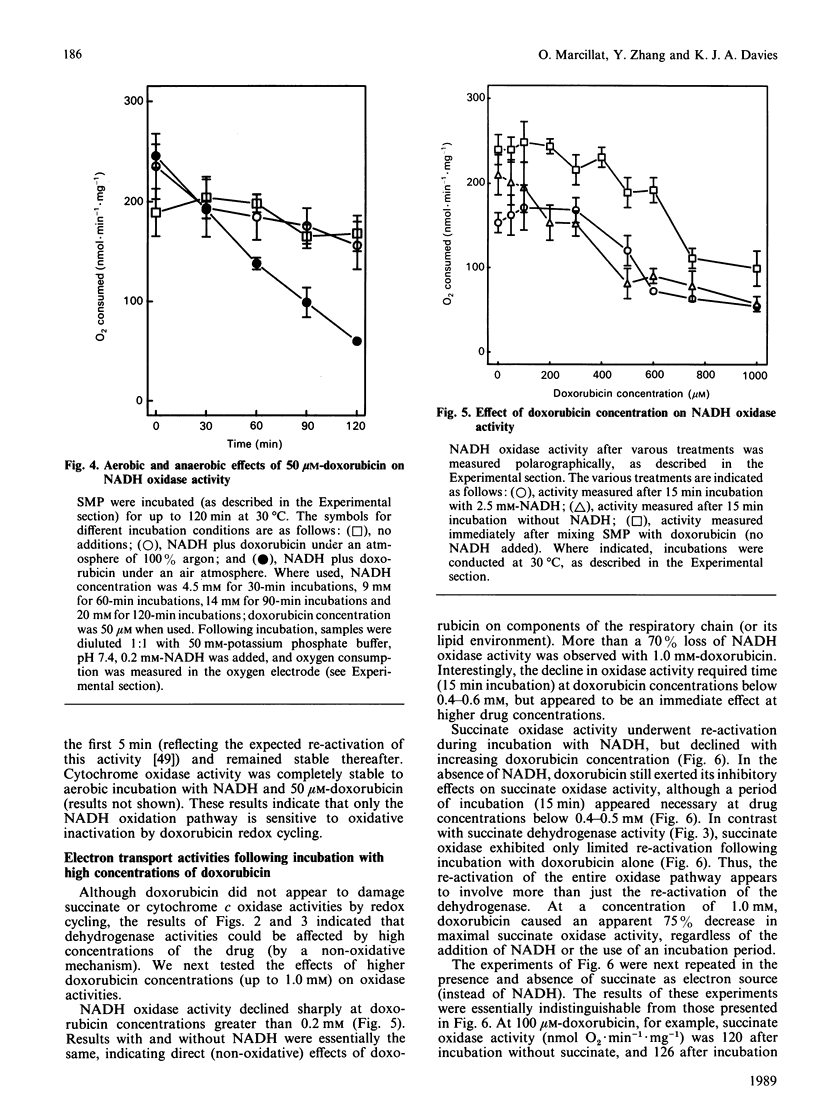
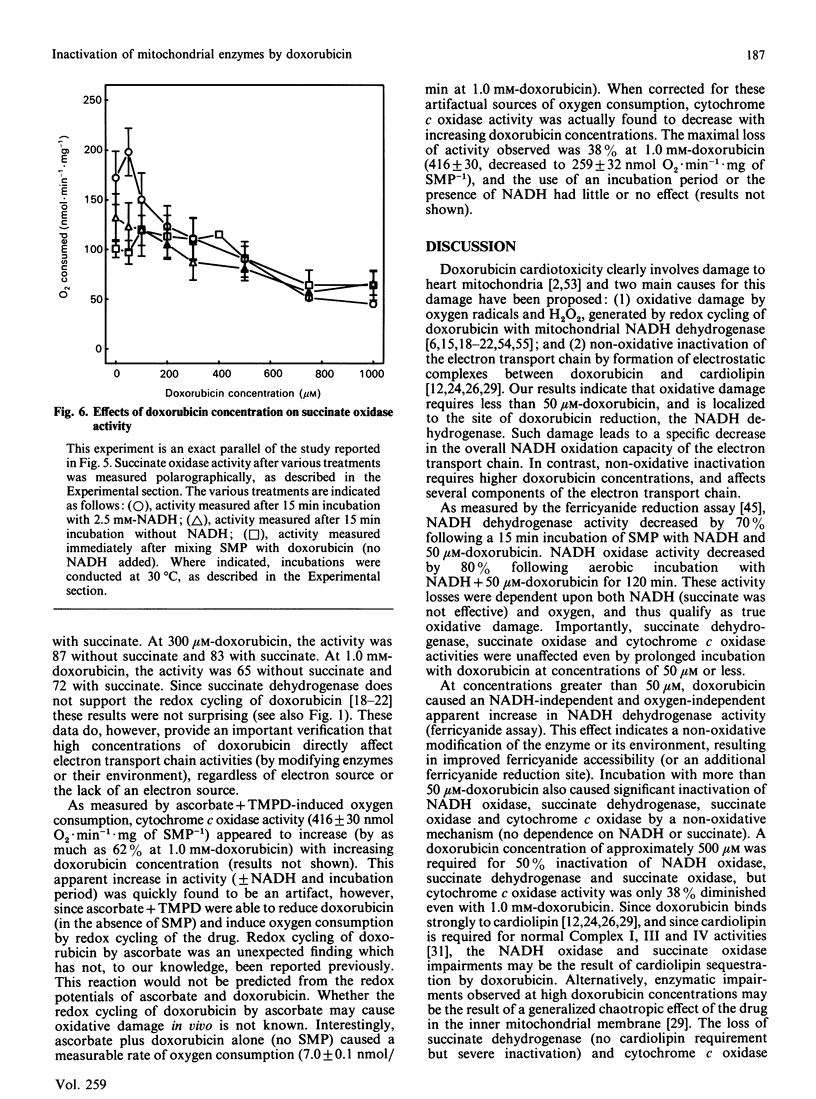
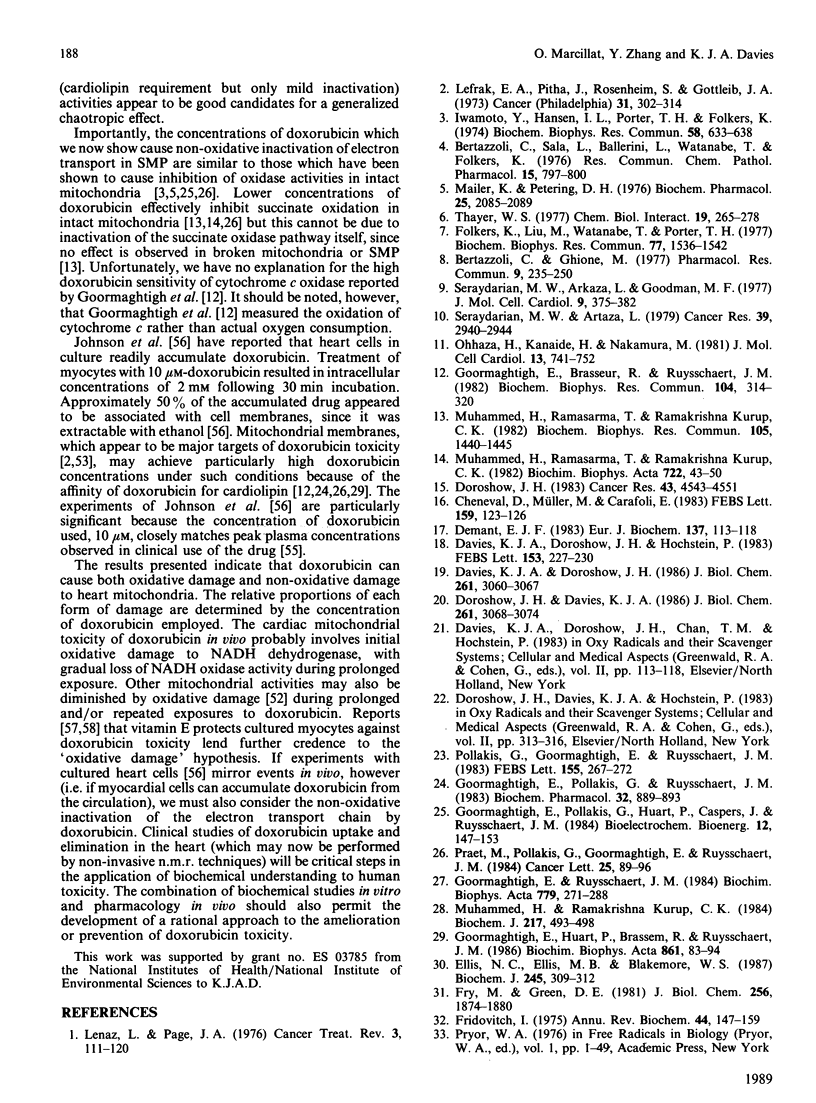
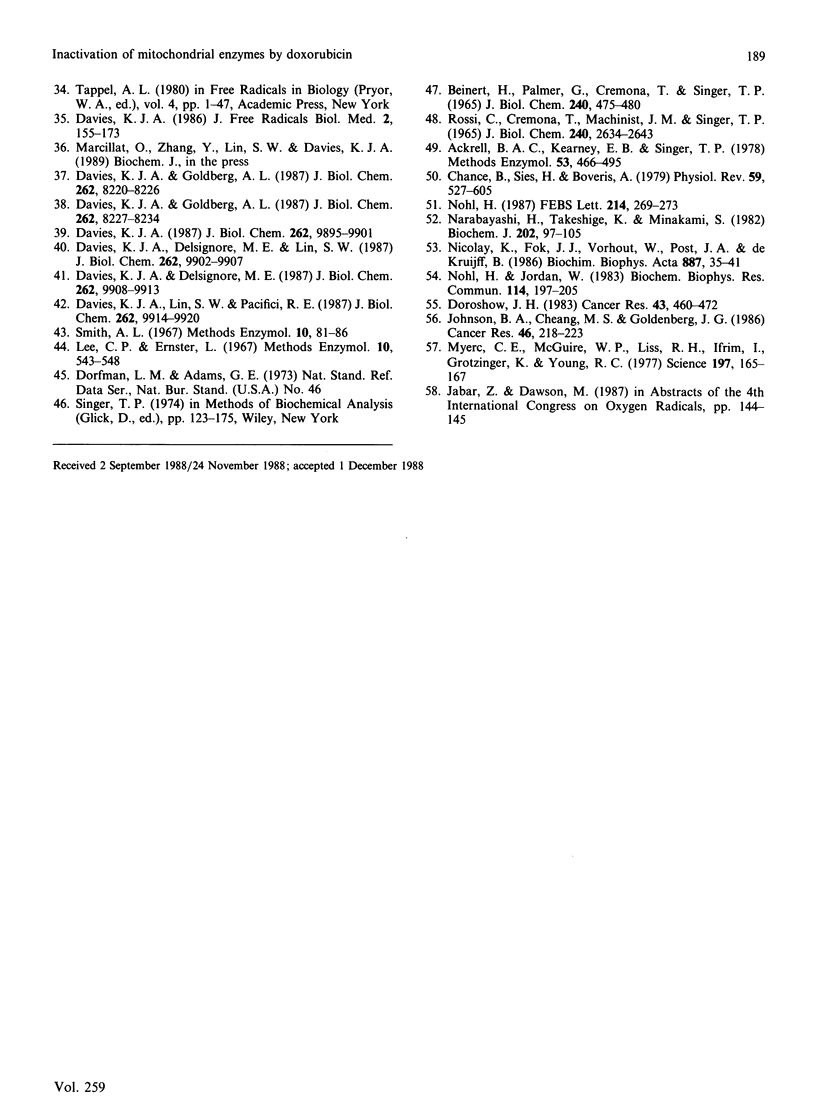
Selected References
These references are in PubMed. This may not be the complete list of references from this article.
- Ackrell B. A., Kearney E. B., Singer T. P. Mammalian succinate dehydrogenase. Methods Enzymol. 1978;53:466–483. doi: 10.1016/s0076-6879(78)53050-4. [DOI] [PubMed] [Google Scholar]
- Bertazzoli C., Ghione M. Adriamycin associated cardiotoxicity: research on prevention with coenzyme Q. Pharmacol Res Commun. 1977 Mar;9(3):235–250. doi: 10.1016/s0031-6989(77)80073-8. [DOI] [PubMed] [Google Scholar]
- Bertazzoli C., Sala L., Ballerini L., Watanabe T., Folkers K. Effect of adriamycin on the activity of the succinate dehydrogenase-coenzyme Q10 reductase of the rabbit myocardium. Res Commun Chem Pathol Pharmacol. 1976 Dec;15(4):797–800. [PubMed] [Google Scholar]
- Chance B., Sies H., Boveris A. Hydroperoxide metabolism in mammalian organs. Physiol Rev. 1979 Jul;59(3):527–605. doi: 10.1152/physrev.1979.59.3.527. [DOI] [PubMed] [Google Scholar]
- Cheneval D., Müller M., Carafoli E. The mitochondrial phosphate carrier reconstituted in liposomes is inhibited by doxorubicin. FEBS Lett. 1983 Aug 8;159(1-2):123–126. doi: 10.1016/0014-5793(83)80429-3. [DOI] [PubMed] [Google Scholar]
- Davies K. J., Delsignore M. E., Lin S. W. Protein damage and degradation by oxygen radicals. II. Modification of amino acids. J Biol Chem. 1987 Jul 15;262(20):9902–9907. [PubMed] [Google Scholar]
- Davies K. J., Delsignore M. E. Protein damage and degradation by oxygen radicals. III. Modification of secondary and tertiary structure. J Biol Chem. 1987 Jul 15;262(20):9908–9913. [PubMed] [Google Scholar]
- Davies K. J., Doroshow J. H., Hochstein P. Mitochondrial NADH dehydrogenase-catalyzed oxygen radical production by adriamycin, and the relative inactivity of 5-iminodaunorubicin. FEBS Lett. 1983 Mar 7;153(1):227–230. doi: 10.1016/0014-5793(83)80153-7. [DOI] [PubMed] [Google Scholar]
- Davies K. J., Doroshow J. H. Redox cycling of anthracyclines by cardiac mitochondria. I. Anthracycline radical formation by NADH dehydrogenase. J Biol Chem. 1986 Mar 5;261(7):3060–3067. [PubMed] [Google Scholar]
- Davies K. J., Goldberg A. L. Oxygen radicals stimulate intracellular proteolysis and lipid peroxidation by independent mechanisms in erythrocytes. J Biol Chem. 1987 Jun 15;262(17):8220–8226. [PubMed] [Google Scholar]
- Davies K. J., Goldberg A. L. Proteins damaged by oxygen radicals are rapidly degraded in extracts of red blood cells. J Biol Chem. 1987 Jun 15;262(17):8227–8234. [PubMed] [Google Scholar]
- Davies K. J., Lin S. W., Pacifici R. E. Protein damage and degradation by oxygen radicals. IV. Degradation of denatured protein. J Biol Chem. 1987 Jul 15;262(20):9914–9920. [PubMed] [Google Scholar]
- Davies K. J. Protein damage and degradation by oxygen radicals. I. general aspects. J Biol Chem. 1987 Jul 15;262(20):9895–9901. [PubMed] [Google Scholar]
- Demant E. J. NADH oxidation in submitochondrial particles protects respiratory chain activity against damage by adriamycin-Fe3+. Eur J Biochem. 1983 Dec 1;137(1-2):113–118. doi: 10.1111/j.1432-1033.1983.tb07803.x. [DOI] [PubMed] [Google Scholar]
- Doroshow J. H. Anthracycline antibiotic-stimulated superoxide, hydrogen peroxide, and hydroxyl radical production by NADH dehydrogenase. Cancer Res. 1983 Oct;43(10):4543–4551. [PubMed] [Google Scholar]
- Doroshow J. H., Davies K. J. Redox cycling of anthracyclines by cardiac mitochondria. II. Formation of superoxide anion, hydrogen peroxide, and hydroxyl radical. J Biol Chem. 1986 Mar 5;261(7):3068–3074. [PubMed] [Google Scholar]
- Doroshow J. H. Effect of anthracycline antibiotics on oxygen radical formation in rat heart. Cancer Res. 1983 Feb;43(2):460–472. [PubMed] [Google Scholar]
- Ellis C. N., Ellis M. B., Blakemore W. S. Effect of adriamycin on heart mitochondrial DNA. Biochem J. 1987 Jul 1;245(1):309–312. doi: 10.1042/bj2450309. [DOI] [PMC free article] [PubMed] [Google Scholar]
- Folkers K., Liu M., Watanabe T., Porter T. H. Inhibition by adriamycin of the mitochondrial biosynthesis of coenzyme Q10 and implication for the cardiotoxicity of adriamycin in cancer patients. Biochem Biophys Res Commun. 1977 Aug 22;77(4):1536–1542. doi: 10.1016/s0006-291x(77)80152-6. [DOI] [PubMed] [Google Scholar]
- Fridovich I. Superoxide dismutases. Annu Rev Biochem. 1975;44:147–159. doi: 10.1146/annurev.bi.44.070175.001051. [DOI] [PubMed] [Google Scholar]
- Fry M., Green D. E. Cardiolipin requirement for electron transfer in complex I and III of the mitochondrial respiratory chain. J Biol Chem. 1981 Feb 25;256(4):1874–1880. [PubMed] [Google Scholar]
- Goormaghtigh E., Brasseur R., Ruysschaert J. M. Adriamycin inactivates cytochrome c oxidase by exclusion of the enzyme from its cardiolipin essential environment. Biochem Biophys Res Commun. 1982 Jan 15;104(1):314–320. doi: 10.1016/0006-291x(82)91976-3. [DOI] [PubMed] [Google Scholar]
- Goormaghtigh E., Huart P., Brasseur R., Ruysschaert J. M. Mechanism of inhibition of mitochondrial enzymatic complex I-III by adriamycin derivatives. Biochim Biophys Acta. 1986 Sep 25;861(1):83–94. doi: 10.1016/0005-2736(86)90374-3. [DOI] [PubMed] [Google Scholar]
- Goormaghtigh E., Pollakis G., Ruysschaert J. M. Mitochondrial membrane modifications induced by adriamycin-mediated electron transport. Biochem Pharmacol. 1983 Mar 1;32(5):889–893. doi: 10.1016/0006-2952(83)90593-2. [DOI] [PubMed] [Google Scholar]
- Goormaghtigh E., Ruysschaert J. M. Anthracycline glycoside-membrane interactions. Biochim Biophys Acta. 1984 Sep 3;779(3):271–288. doi: 10.1016/0304-4157(84)90013-3. [DOI] [PubMed] [Google Scholar]
- Iwamoto Y., Hansen I. L., Porter T. H., Folkers K. Inhibition of coenzyme Q10-enzymes, succinoxidase and NADH-oxidase, by adriamycin and other quinones having antitumor activity. Biochem Biophys Res Commun. 1974 Jun 4;58(3):633–638. doi: 10.1016/s0006-291x(74)80465-1. [DOI] [PubMed] [Google Scholar]
- Johnson B. A., Cheang M. S., Goldenberg G. J. Comparison of adriamycin uptake in chick embryo heart and liver cells an murine L5178Y lymphoblasts in vitro: role of drug uptake in cardiotoxicity. Cancer Res. 1986 Jan;46(1):218–223. [PubMed] [Google Scholar]
- Lefrak E. A., Pitha J., Rosenheim S., Gottlieb J. A. A clinicopathologic analysis of adriamycin cardiotoxicity. Cancer. 1973 Aug;32(2):302–314. doi: 10.1002/1097-0142(197308)32:2<302::aid-cncr2820320205>3.0.co;2-2. [DOI] [PubMed] [Google Scholar]
- Lenaz L., Page J. A. Cardiotoxicity of adriamycin and related anthracyclines. Cancer Treat Rev. 1976 Sep;3(3):111–120. doi: 10.1016/s0305-7372(76)80018-7. [DOI] [PubMed] [Google Scholar]
- Mailer K., Petering D. H. Inhibition of oxidative phosphorylation in tumor cells and mitochondria by daunomycin and adriamycin. Biochem Pharmacol. 1976 Sep 15;25(18):2085–2089. doi: 10.1016/0006-2952(76)90434-2. [DOI] [PubMed] [Google Scholar]
- Muhammed H., Kurup C. K. Influence of ubiquinone on the inhibitory effect of adriamycin on mitochondrial oxidative phosphorylation. Biochem J. 1984 Jan 15;217(2):493–498. doi: 10.1042/bj2170493. [DOI] [PMC free article] [PubMed] [Google Scholar]
- Muhammed H., Ramasarma T., Kurup C. K. A 'hexokinase effect' in the inhibition of oxidative phosphorylation in heart muscle mitochondria by adriamycin. Biochem Biophys Res Commun. 1982 Apr 29;105(4):1440–1445. doi: 10.1016/0006-291x(82)90949-4. [DOI] [PubMed] [Google Scholar]
- Muhammed H., Ramasarma T., Kurup C. K. Inhibition of mitochondrial oxidative phosphorylation by adriamycin. Biochim Biophys Acta. 1983 Jan 13;722(1):43–50. doi: 10.1016/0005-2728(83)90155-x. [DOI] [PubMed] [Google Scholar]
- Myers C. E., McGuire W. P., Liss R. H., Ifrim I., Grotzinger K., Young R. C. Adriamycin: the role of lipid peroxidation in cardiac toxicity and tumor response. Science. 1977 Jul 8;197(4299):165–167. doi: 10.1126/science.877547. [DOI] [PubMed] [Google Scholar]
- Narabayashi H., Takeshige K., Minakami S. Alteration of inner-membrane components and damage to electron-transfer activities of bovine heart submitochondrial particles induced by NADPH-dependent lipid peroxidation. Biochem J. 1982 Jan 15;202(1):97–105. doi: 10.1042/bj2020097. [DOI] [PMC free article] [PubMed] [Google Scholar]
- Nicolay K., Fok J. J., Voorhout W., Post J. A., de Kruijff B. Cytofluorescence detection of adriamycin-mitochondria interactions in isolated, perfused rat heart. Biochim Biophys Acta. 1986 Jun 16;887(1):35–41. doi: 10.1016/0167-4889(86)90119-9. [DOI] [PubMed] [Google Scholar]
- Nohl H. A novel superoxide radical generator in heart mitochondria. FEBS Lett. 1987 Apr 20;214(2):269–273. doi: 10.1016/0014-5793(87)80068-6. [DOI] [PubMed] [Google Scholar]
- Nohl H., Jordan W. OH.-generation by adriamycin semiquinone and H2O2; an explanation for the cardiotoxicity of anthracycline antibiotics. Biochem Biophys Res Commun. 1983 Jul 18;114(1):197–205. doi: 10.1016/0006-291x(83)91613-3. [DOI] [PubMed] [Google Scholar]
- Ohhara H., Kanaide H., Nakamura M. A protective effect of coenzyme Q10 on the adriamycin-induced cardiotoxicity in the isolated perfused rat heart. J Mol Cell Cardiol. 1981 Aug;13(8):741–752. doi: 10.1016/0022-2828(81)90256-x. [DOI] [PubMed] [Google Scholar]
- Pollakis G., Goormaghtigh E., Ruysschaert J. M. Role of the quinone structure in the mitochondrial damage induced by antitumor anthracyclines. Comparison of adriamycin and 5-iminodaunorubicin. FEBS Lett. 1983 May 8;155(2):267–272. doi: 10.1016/0014-5793(82)80618-2. [DOI] [PubMed] [Google Scholar]
- Praet M., Pollakis G., Goormaghtigh E., Ruysschaert J. M. Damages of the mitochondrial membrane in Adriamycin treated mice. Cancer Lett. 1984 Nov;25(1):89–96. doi: 10.1016/s0304-3835(84)80030-0. [DOI] [PubMed] [Google Scholar]
- ROSSI C., CREMONA T., MACHINIST J. M., SINGER T. P. STUDIES ON THE RESPIRATORY CHAIN-LINKED REDUCED NICOTINAMIDE ADENINE DINUCLEOTIDE DEHYDROGENASE.8. INACTIVATION, FRAGMENTATION, AND PROTECTION BY SUBSTRATES. J Biol Chem. 1965 Jun;240:2634–2643. [PubMed] [Google Scholar]
- SCOTT E. M., DUNCAN I. W., EKSTRAND V. THE REDUCED PYRIDINE NUCLEOTIDE DEHYDROGENASES OF HUMAN ERYTHROCYTES. J Biol Chem. 1965 Jan;240:475–480. [PubMed] [Google Scholar]
- Seraydarian M. W., Artaza L., Goodman M. F. Adriamycin: effect on mammalian cardiac cells in culture. I. Cell population and energy metabolism. J Mol Cell Cardiol. 1977 May;9(5):375–382. doi: 10.1016/s0022-2828(77)80004-7. [DOI] [PubMed] [Google Scholar]
- Seraydarian M. W., Artaza L. Modification by adenosine of the effect of adriamycin on myocardial cells in culture. Cancer Res. 1979 Aug;39(8):2940–2944. [PubMed] [Google Scholar]
- Singer T. P. Determination of the activity of succinate, NADH, choline, and alpha-glycerophosphate dehydrogenases. Methods Biochem Anal. 1974;22:123–175. doi: 10.1002/9780470110423.ch3. [DOI] [PubMed] [Google Scholar]
- Thayer W. S. Adriamycin stimulated superoxide formation in submitochondrial particles. Chem Biol Interact. 1977 Dec;19(3):265–278. doi: 10.1016/0009-2797(77)90050-3. [DOI] [PubMed] [Google Scholar]