Abstract
1. Regulation of unloaded cell shortening and relaxation by sarcolemmal Na(+)-Ca2+ exchange was investigated in rat ventricular myocytes. Contraction of single cells at 22 +/- 1 degrees C was measured simultaneously with membrane current and voltage using the whole-cell voltage clamp technique in combination with a video edge-detection device. 2. The extent of mechanical activation (cell shortening amplitude) was strongly dependent on diastolic membrane potential over the voltage range -140 to -50 mV. This voltage sensitivity of contraction was abolished completely when a recently described inhibitory peptide of the cardiac Na(+)-Ca2+ exchanger (XIP, 2 x 10(-5) M) was present in the recording pipette, demonstrating that in rat ventricular cells Na(+)-Ca2+ exchange is modulated by diastolic membrane potential. 3. Possible influences of Na(+)-Ca2+ exchange on contraction were studied from a holding potential of -80 mV. Depolarizations (-50 to +60 mV) resulted in a bell-shaped shortening-voltage (S-V) relationship. These contractions were suppressed completely by either Cd2+ (10(-4) M) or verapamil (10(-5) M), but remained unchanged during superfusion with tetrodotoxin (TTX, 1.5 x 10(-5) M), when [NA+]o was reduced from 140 to 10 mM by substitution with either Li+ or Cs+ ions or when pipette Na+ was varied between 8 and 13 mM. XIP (2 x 10(-5) M) increased the magnitude and duration of twitch contractions, but had no effect on the shape of the S-V relationship. Thus, the Ca2+ current but not the Na+ current or Ca2+ influx due to reversed Na(+)-Ca2+ exchange can release Ca2+ from the sarcoplasmic reticulum (SR) under these experimental conditions. 4. The effect of the rate of repolarization on cell shortening was studied under voltage clamp by applying ramp waveforms immediately following the depolarizations which activated contraction. Although slowing of the rate of repolarization had no effect on the first contraction following a train of conditioning depolarizations, a positive inotropic effect developed thereafter. 5. Caffeine (10 mM) was applied to determine whether Na(+)-Ca2+ exchange and/or Ca2+ sequestration/buffering by the sarcoplasmic reticulum were primarily responsible for these inotropic effects. In the presence of caffeine the positive inotropic effect developed fully during the first test depolarization. Changes in the rate of repolarization had much less effect on shortening in cells dialysed intracellularly with XIP (2 x 10(-5) M). In combination, these results suggest that the changes in the inotropic effects resulting from changes in rate of repolarization may be due to altered loading and release of Ca2+ from the SR.(ABSTRACT TRUNCATED AT 400 WORDS)
Full text
PDF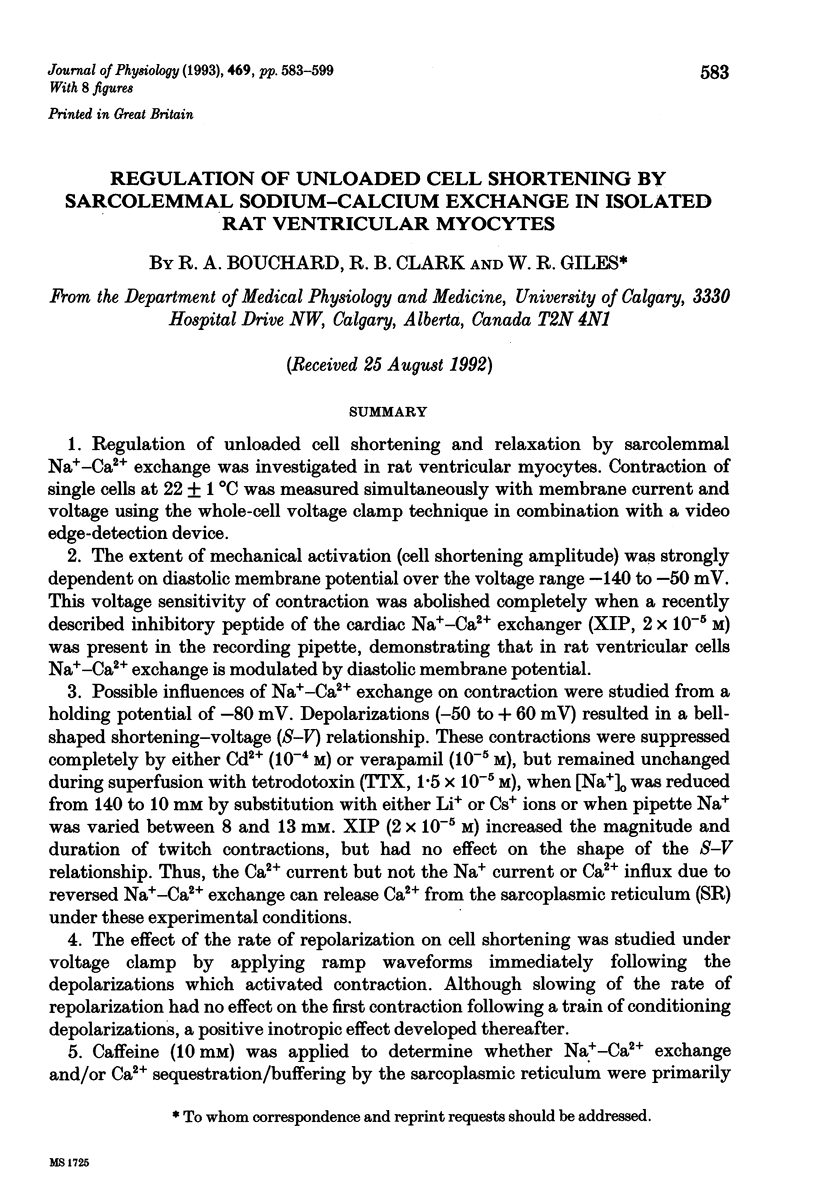
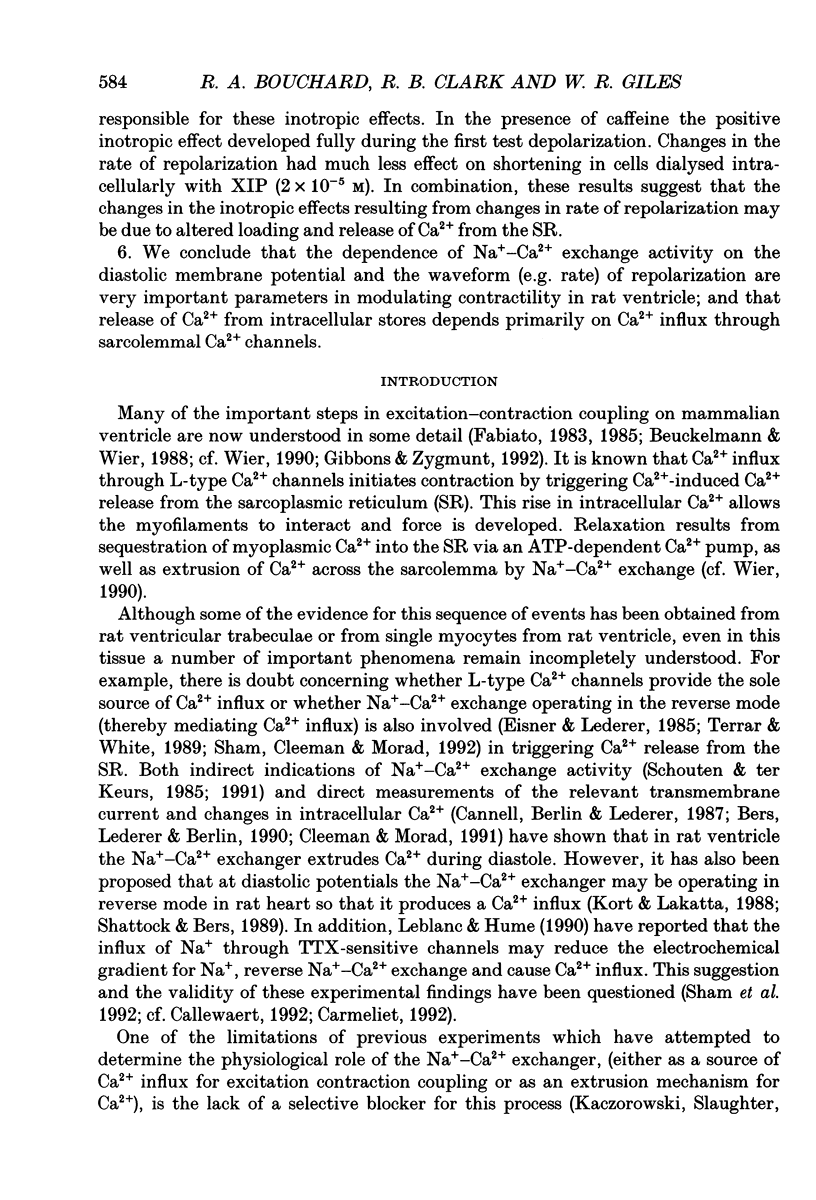
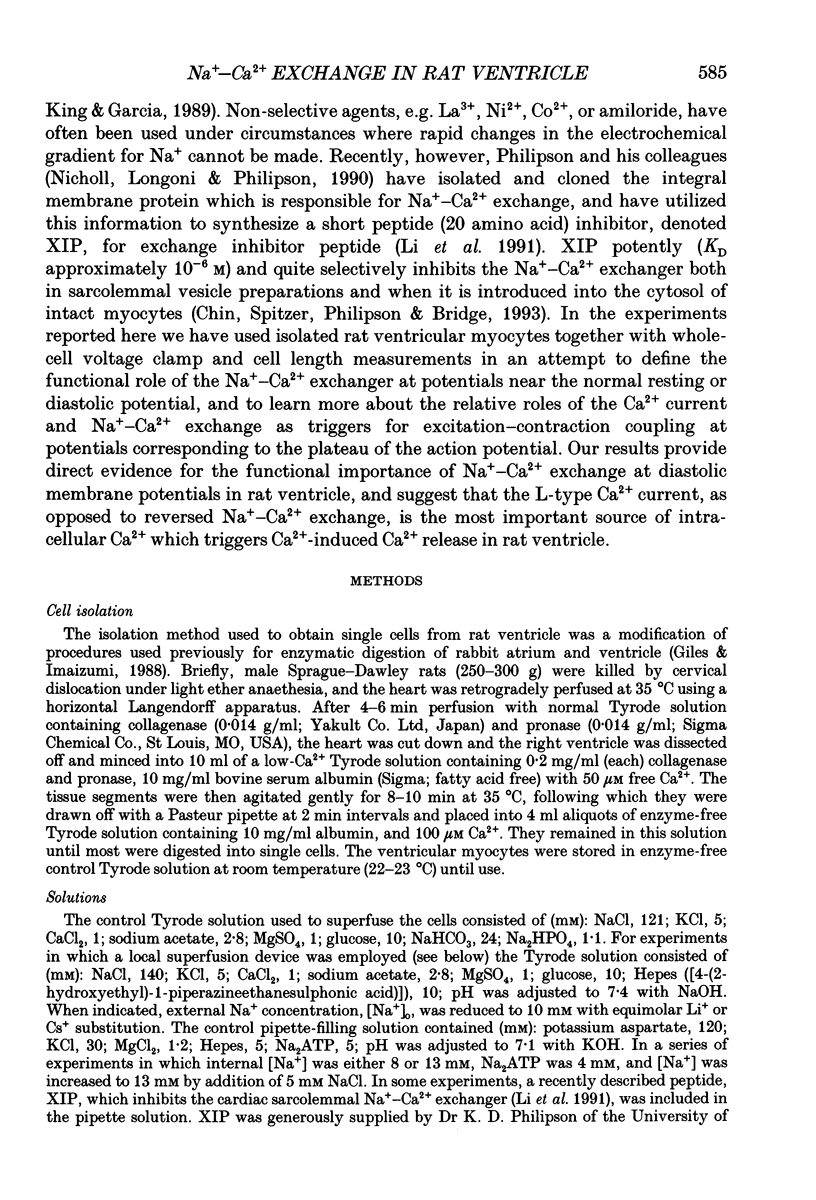
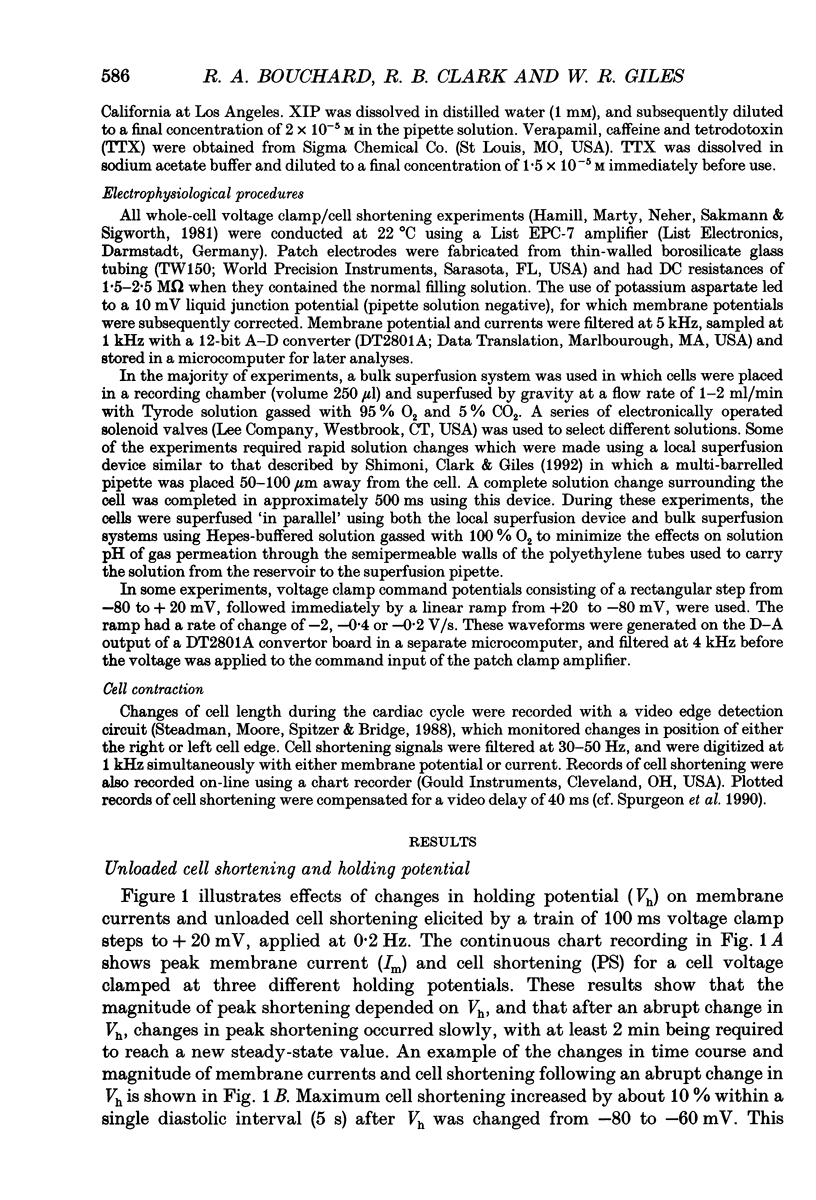
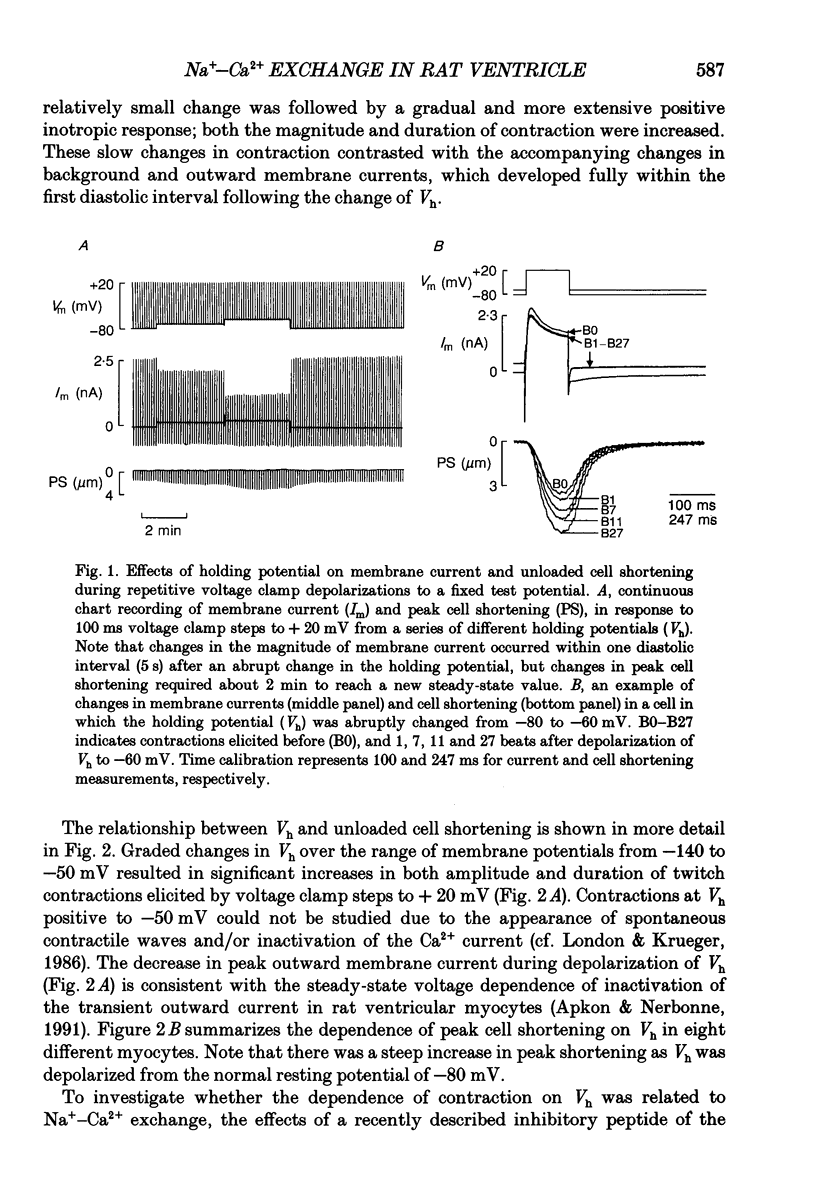
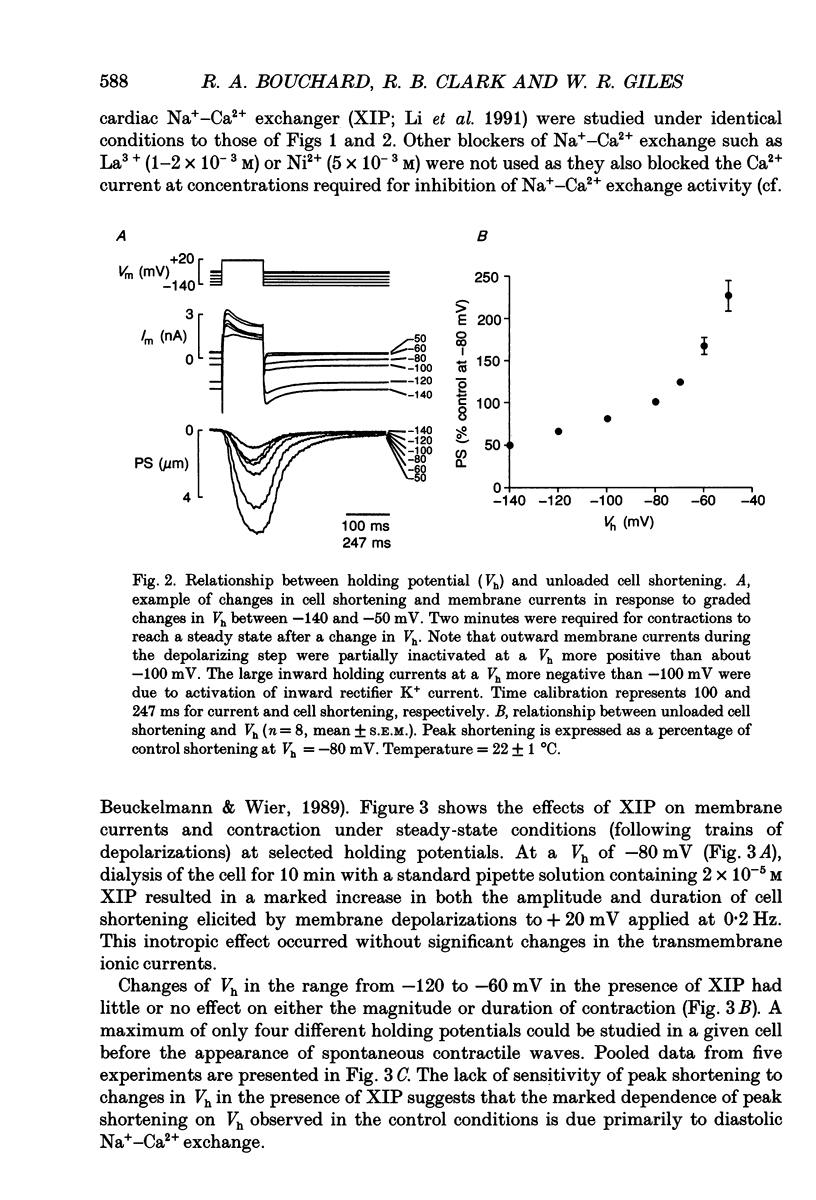
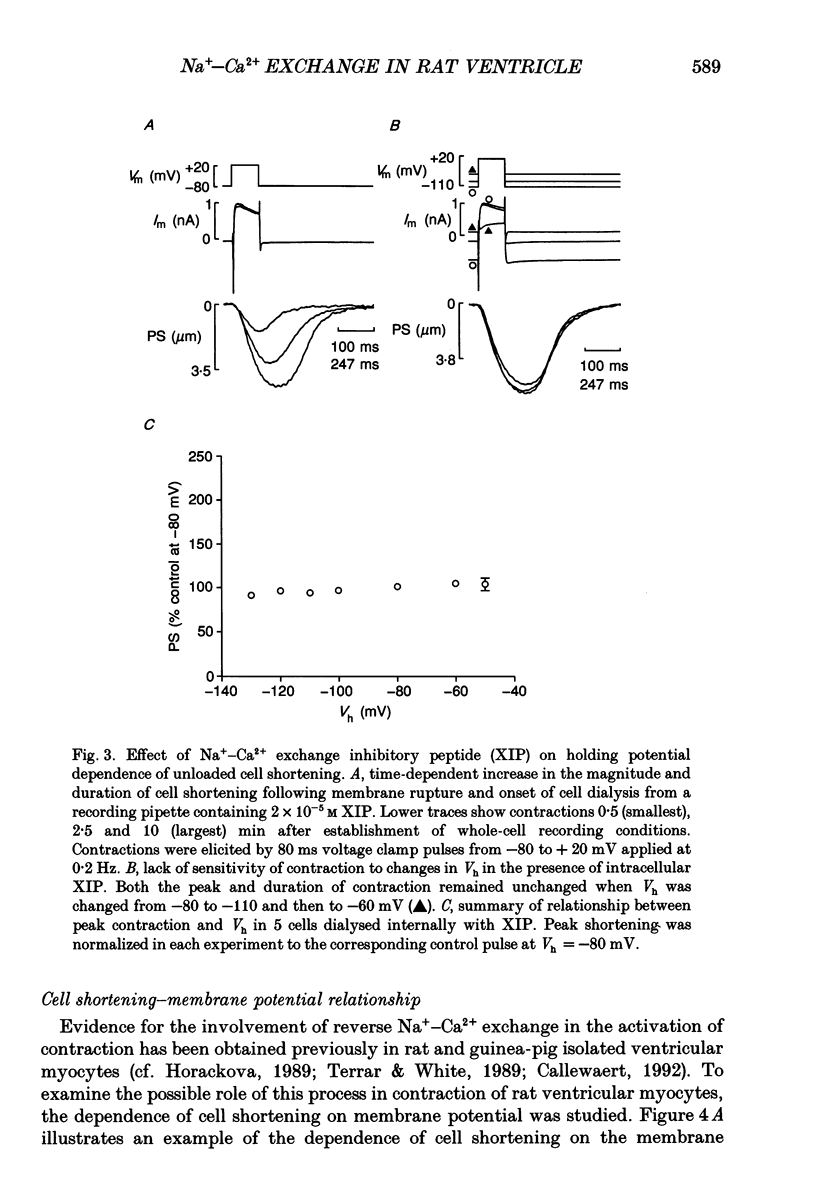
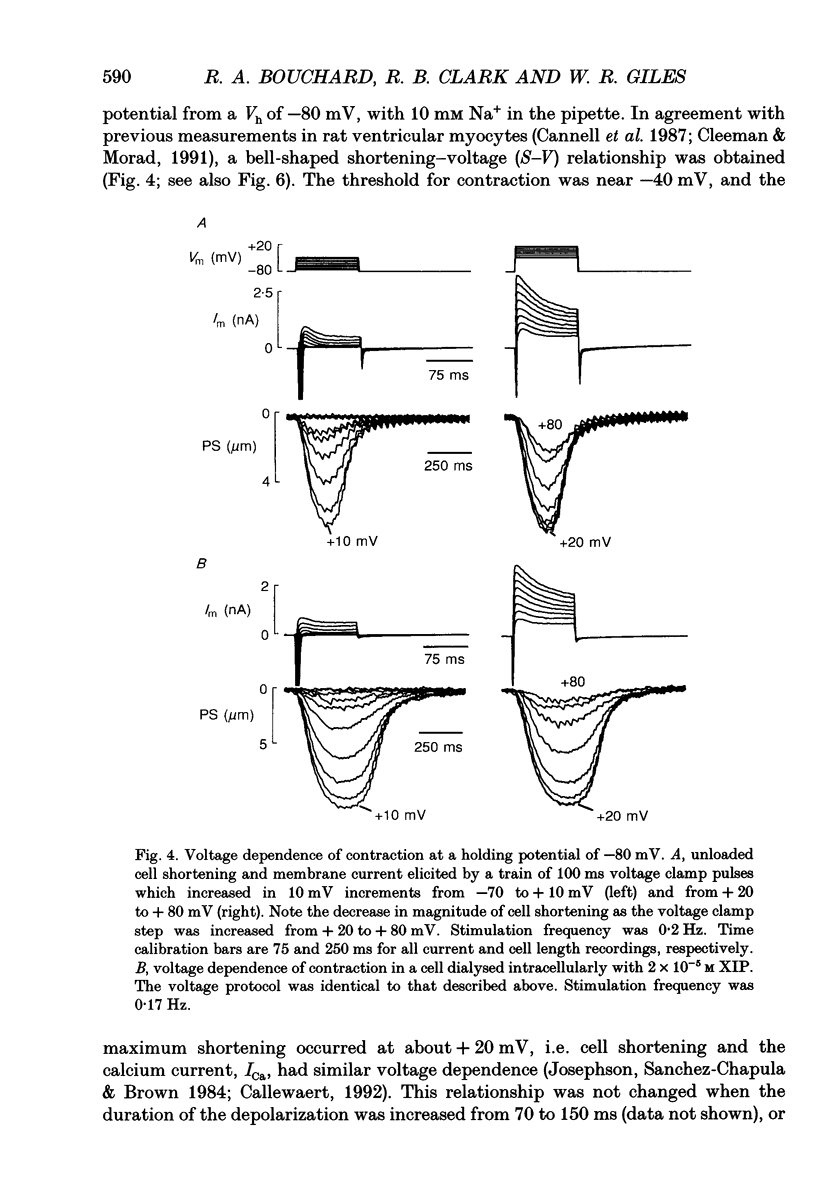
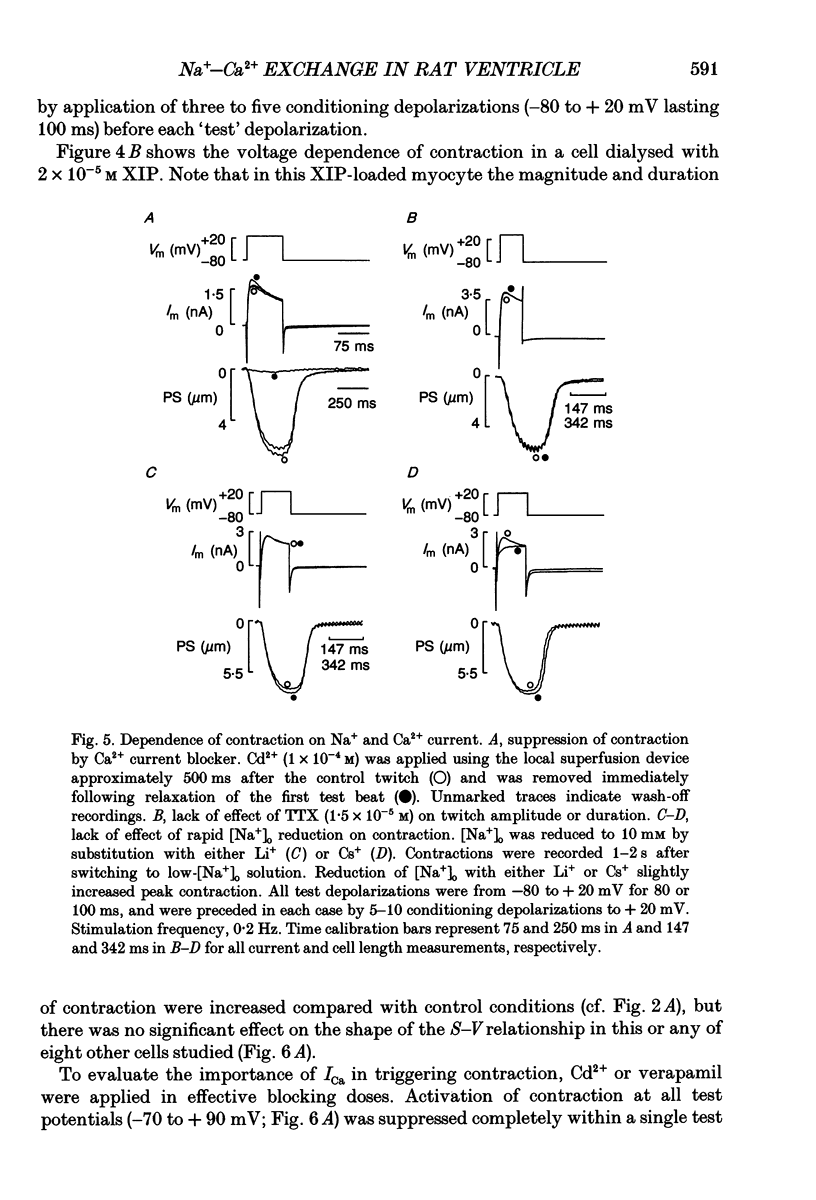
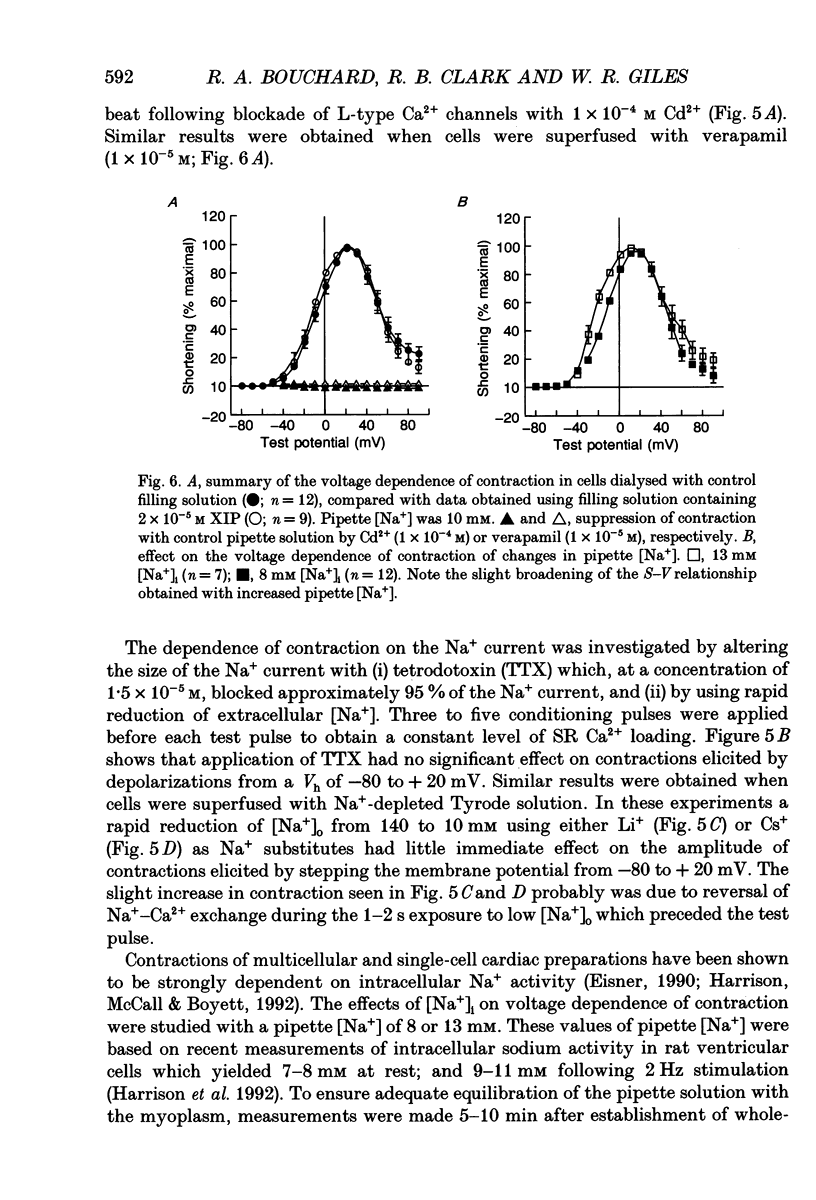
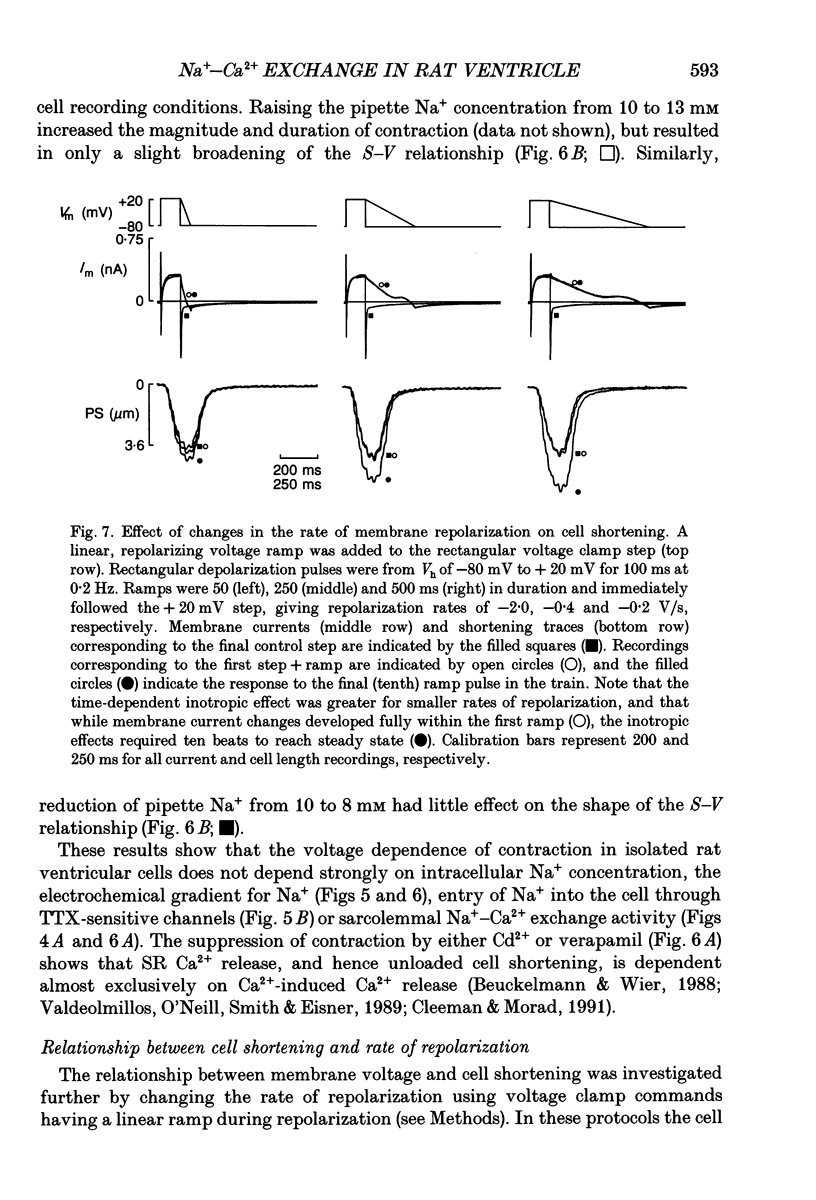
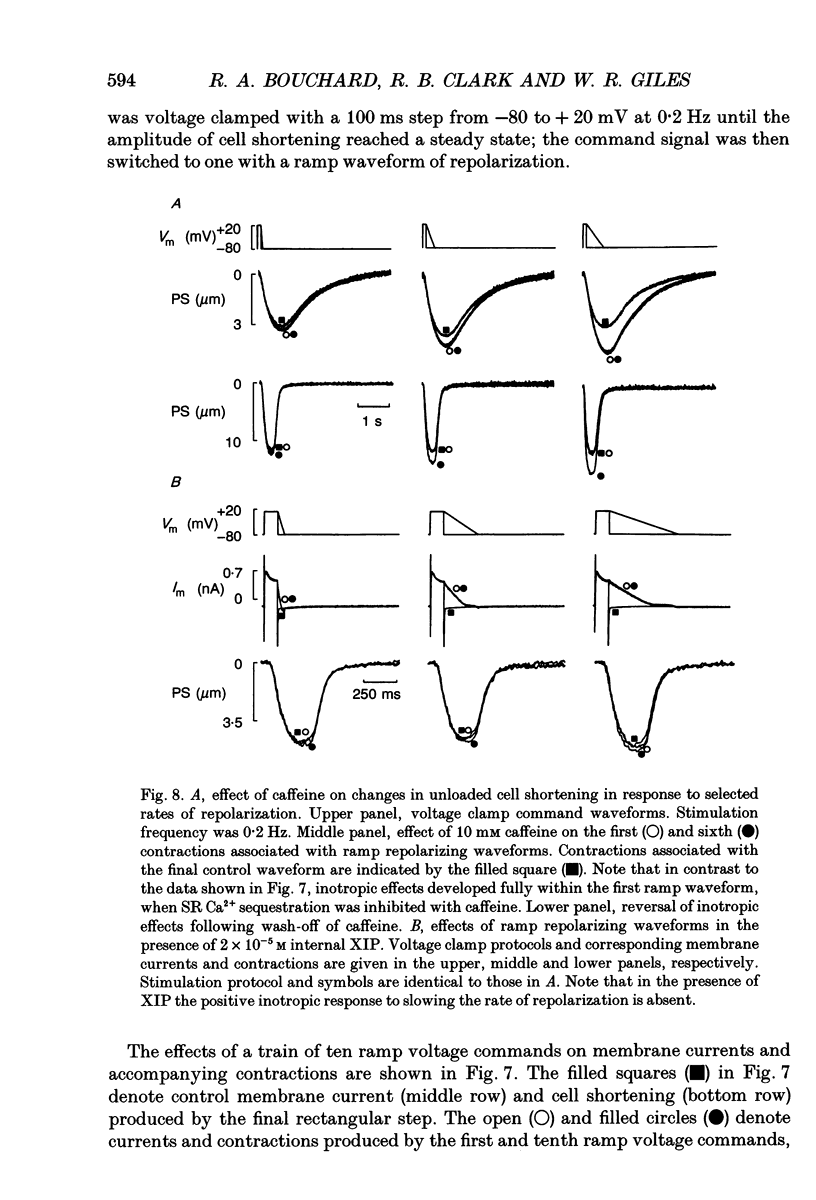
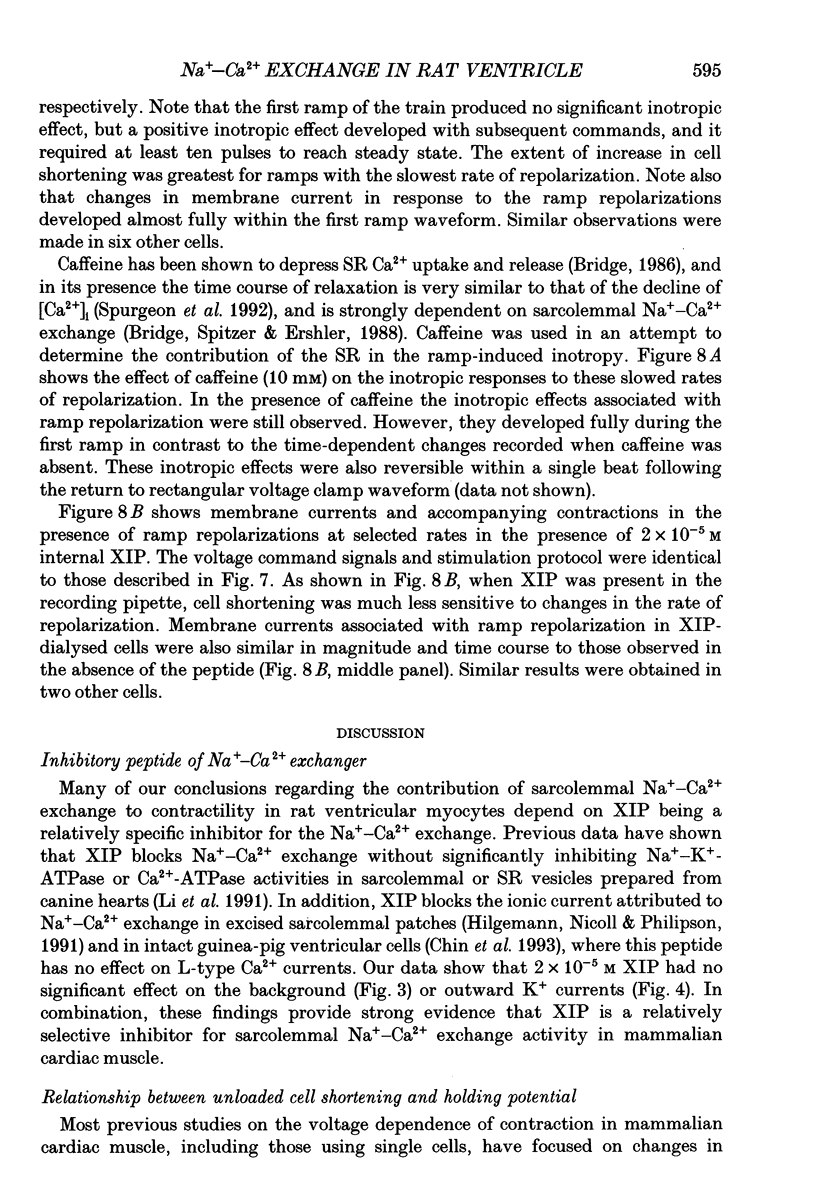
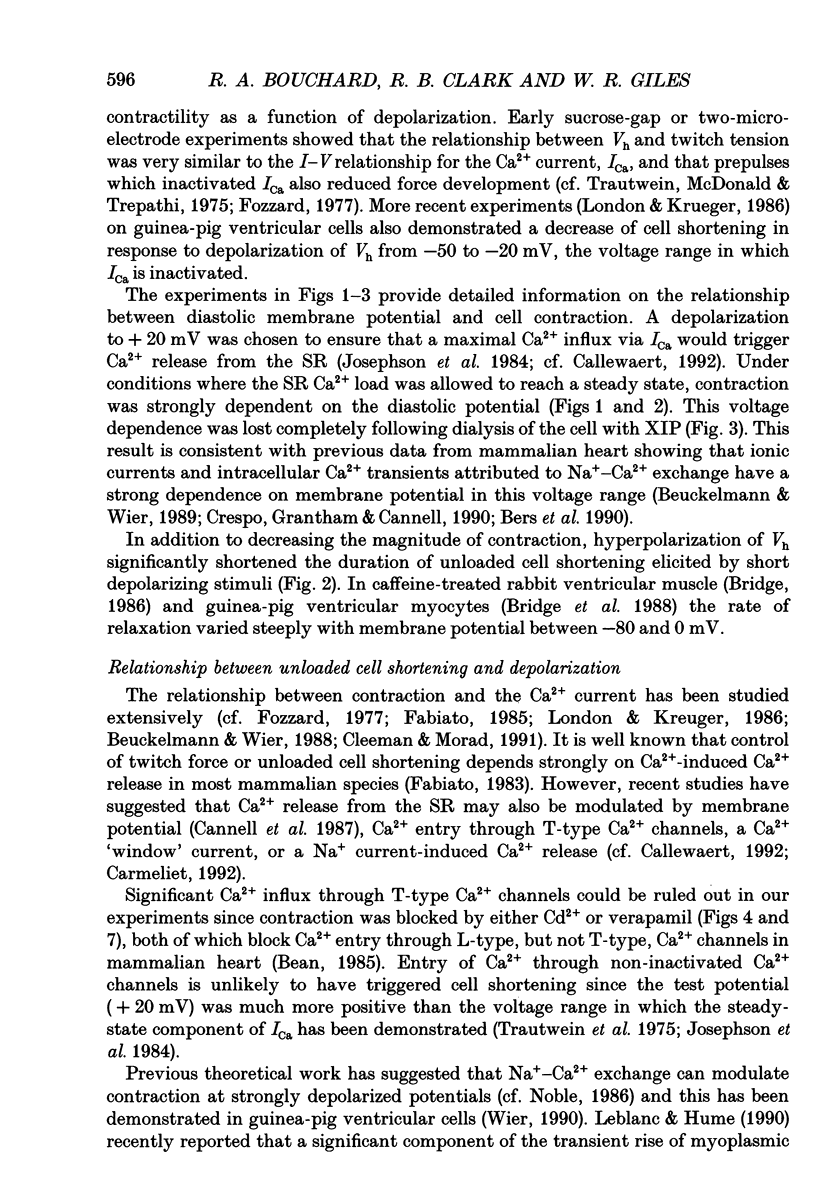
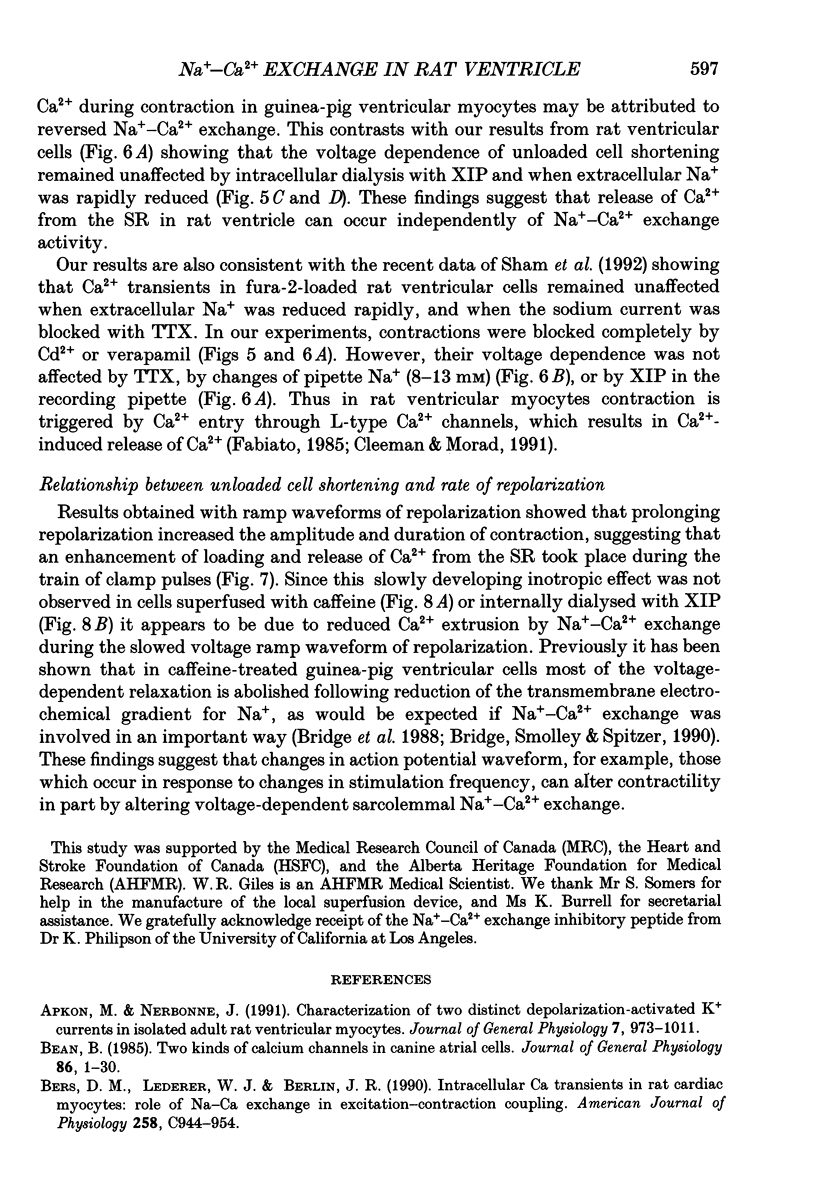
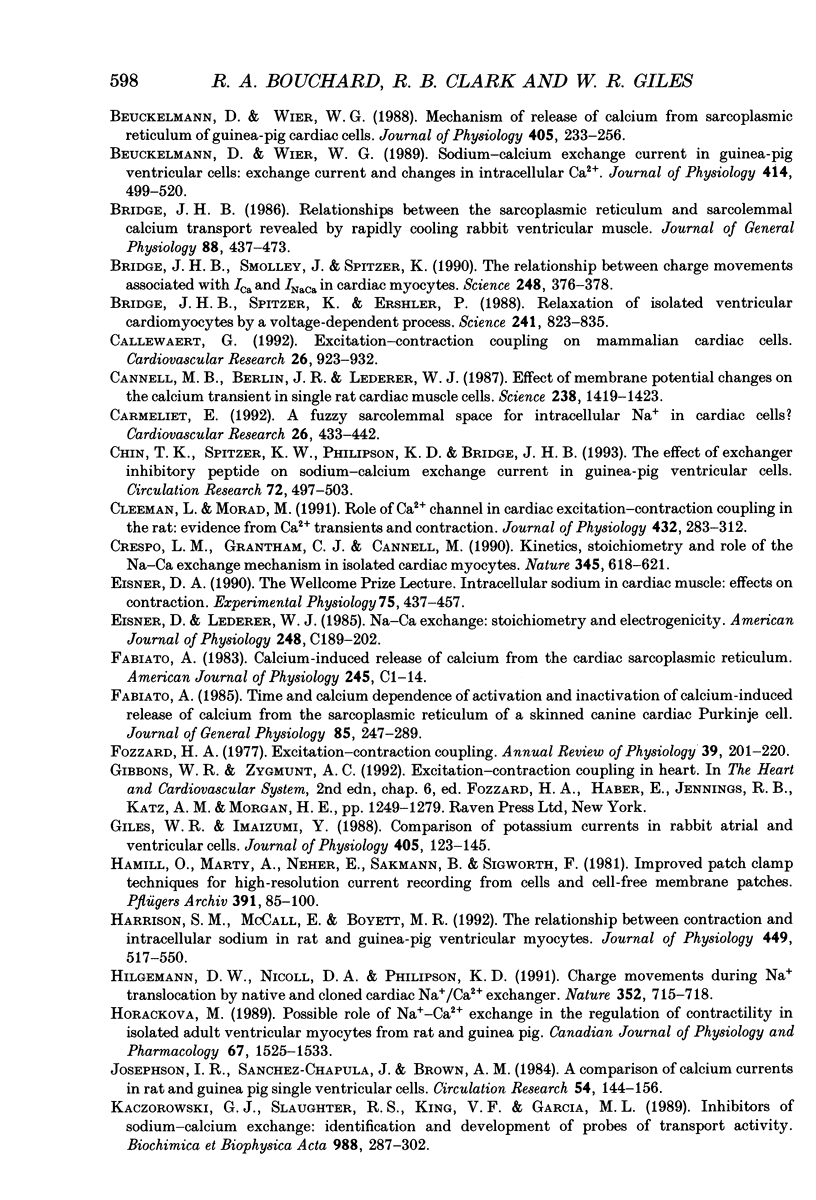
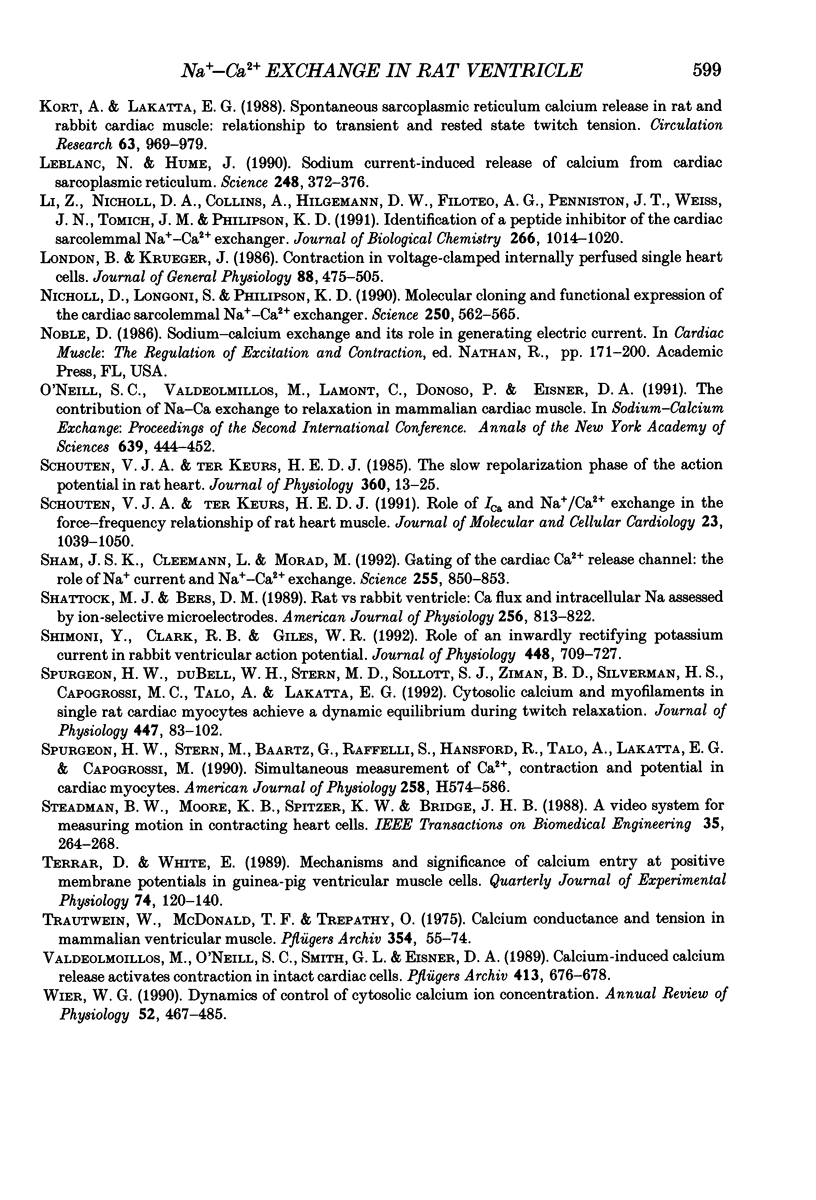
Selected References
These references are in PubMed. This may not be the complete list of references from this article.
- Apkon M., Nerbonne J. M. Characterization of two distinct depolarization-activated K+ currents in isolated adult rat ventricular myocytes. J Gen Physiol. 1991 May;97(5):973–1011. doi: 10.1085/jgp.97.5.973. [DOI] [PMC free article] [PubMed] [Google Scholar]
- Bean B. P. Two kinds of calcium channels in canine atrial cells. Differences in kinetics, selectivity, and pharmacology. J Gen Physiol. 1985 Jul;86(1):1–30. doi: 10.1085/jgp.86.1.1. [DOI] [PMC free article] [PubMed] [Google Scholar]
- Bers D. M., Lederer W. J., Berlin J. R. Intracellular Ca transients in rat cardiac myocytes: role of Na-Ca exchange in excitation-contraction coupling. Am J Physiol. 1990 May;258(5 Pt 1):C944–C954. doi: 10.1152/ajpcell.1990.258.5.C944. [DOI] [PubMed] [Google Scholar]
- Beuckelmann D. J., Wier W. G. Mechanism of release of calcium from sarcoplasmic reticulum of guinea-pig cardiac cells. J Physiol. 1988 Nov;405:233–255. doi: 10.1113/jphysiol.1988.sp017331. [DOI] [PMC free article] [PubMed] [Google Scholar]
- Beuckelmann D. J., Wier W. G. Sodium-calcium exchange in guinea-pig cardiac cells: exchange current and changes in intracellular Ca2+. J Physiol. 1989 Jul;414:499–520. doi: 10.1113/jphysiol.1989.sp017700. [DOI] [PMC free article] [PubMed] [Google Scholar]
- Bridge J. H. Relationships between the sarcoplasmic reticulum and sarcolemmal calcium transport revealed by rapidly cooling rabbit ventricular muscle. J Gen Physiol. 1986 Oct;88(4):437–473. doi: 10.1085/jgp.88.4.437. [DOI] [PMC free article] [PubMed] [Google Scholar]
- Bridge J. H., Smolley J. R., Spitzer K. W. The relationship between charge movements associated with ICa and INa-Ca in cardiac myocytes. Science. 1990 Apr 20;248(4953):376–378. doi: 10.1126/science.2158147. [DOI] [PubMed] [Google Scholar]
- Bridge J. H., Spitzer K. W., Ershler P. R. Relaxation of isolated ventricular cardiomyocytes by a voltage-dependent process. Science. 1988 Aug 12;241(4867):823–825. doi: 10.1126/science.3406740. [DOI] [PubMed] [Google Scholar]
- Callewaert G. Excitation-contraction coupling in mammalian cardiac cells. Cardiovasc Res. 1992 Oct;26(10):923–932. doi: 10.1093/cvr/26.10.923. [DOI] [PubMed] [Google Scholar]
- Cannell M. B., Berlin J. R., Lederer W. J. Effect of membrane potential changes on the calcium transient in single rat cardiac muscle cells. Science. 1987 Dec 4;238(4832):1419–1423. doi: 10.1126/science.2446391. [DOI] [PubMed] [Google Scholar]
- Carmeliet E. A fuzzy subsarcolemmal space for intracellular Na+ in cardiac cells? Cardiovasc Res. 1992 May;26(5):433–442. doi: 10.1093/cvr/26.5.433. [DOI] [PubMed] [Google Scholar]
- Chin T. K., Spitzer K. W., Philipson K. D., Bridge J. H. The effect of exchanger inhibitory peptide (XIP) on sodium-calcium exchange current in guinea pig ventricular cells. Circ Res. 1993 Mar;72(3):497–503. doi: 10.1161/01.res.72.3.497. [DOI] [PubMed] [Google Scholar]
- Cleemann L., Morad M. Role of Ca2+ channel in cardiac excitation-contraction coupling in the rat: evidence from Ca2+ transients and contraction. J Physiol. 1991 Jan;432:283–312. doi: 10.1113/jphysiol.1991.sp018385. [DOI] [PMC free article] [PubMed] [Google Scholar]
- Crespo L. M., Grantham C. J., Cannell M. B. Kinetics, stoichiometry and role of the Na-Ca exchange mechanism in isolated cardiac myocytes. Nature. 1990 Jun 14;345(6276):618–621. doi: 10.1038/345618a0. [DOI] [PubMed] [Google Scholar]
- Eisner D. A., Lederer W. J. Na-Ca exchange: stoichiometry and electrogenicity. Am J Physiol. 1985 Mar;248(3 Pt 1):C189–C202. doi: 10.1152/ajpcell.1985.248.3.C189. [DOI] [PubMed] [Google Scholar]
- Eisner D. A. The Wellcome prize lecture. Intracellular sodium in cardiac muscle: effects on contraction. Exp Physiol. 1990 Jul;75(4):437–457. doi: 10.1113/expphysiol.1990.sp003422. [DOI] [PubMed] [Google Scholar]
- Fabiato A. Calcium-induced release of calcium from the cardiac sarcoplasmic reticulum. Am J Physiol. 1983 Jul;245(1):C1–14. doi: 10.1152/ajpcell.1983.245.1.C1. [DOI] [PubMed] [Google Scholar]
- Fabiato A. Time and calcium dependence of activation and inactivation of calcium-induced release of calcium from the sarcoplasmic reticulum of a skinned canine cardiac Purkinje cell. J Gen Physiol. 1985 Feb;85(2):247–289. doi: 10.1085/jgp.85.2.247. [DOI] [PMC free article] [PubMed] [Google Scholar]
- Fozzard H. A. Heart: excitation-contraction coupling. Annu Rev Physiol. 1977;39:201–220. doi: 10.1146/annurev.ph.39.030177.001221. [DOI] [PubMed] [Google Scholar]
- Giles W. R., Imaizumi Y. Comparison of potassium currents in rabbit atrial and ventricular cells. J Physiol. 1988 Nov;405:123–145. doi: 10.1113/jphysiol.1988.sp017325. [DOI] [PMC free article] [PubMed] [Google Scholar]
- Hamill O. P., Marty A., Neher E., Sakmann B., Sigworth F. J. Improved patch-clamp techniques for high-resolution current recording from cells and cell-free membrane patches. Pflugers Arch. 1981 Aug;391(2):85–100. doi: 10.1007/BF00656997. [DOI] [PubMed] [Google Scholar]
- Harrison S. M., McCall E., Boyett M. R. The relationship between contraction and intracellular sodium in rat and guinea-pig ventricular myocytes. J Physiol. 1992 Apr;449:517–550. doi: 10.1113/jphysiol.1992.sp019100. [DOI] [PMC free article] [PubMed] [Google Scholar]
- Hilgemann D. W., Nicoll D. A., Philipson K. D. Charge movement during Na+ translocation by native and cloned cardiac Na+/Ca2+ exchanger. Nature. 1991 Aug 22;352(6337):715–718. doi: 10.1038/352715a0. [DOI] [PubMed] [Google Scholar]
- Horackova M. Possible role of Na(+)-Ca2+ exchange in the regulation of contractility in isolated adult ventricular myocytes from rat and guinea pig. Can J Physiol Pharmacol. 1989 Dec;67(12):1525–1533. doi: 10.1139/y89-246. [DOI] [PubMed] [Google Scholar]
- Josephson I. R., Sanchez-Chapula J., Brown A. M. A comparison of calcium currents in rat and guinea pig single ventricular cells. Circ Res. 1984 Feb;54(2):144–156. doi: 10.1161/01.res.54.2.144. [DOI] [PubMed] [Google Scholar]
- Kaczorowski G. J., Slaughter R. S., King V. F., Garcia M. L. Inhibitors of sodium-calcium exchange: identification and development of probes of transport activity. Biochim Biophys Acta. 1989 May 9;988(2):287–302. doi: 10.1016/0304-4157(89)90022-1. [DOI] [PubMed] [Google Scholar]
- Kort A. A., Lakatta E. G. Spontaneous sarcoplasmic reticulum calcium release in rat and rabbit cardiac muscle: relation to transient and rested-state twitch tension. Circ Res. 1988 Nov;63(5):969–979. doi: 10.1161/01.res.63.5.969. [DOI] [PubMed] [Google Scholar]
- Leblanc N., Hume J. R. Sodium current-induced release of calcium from cardiac sarcoplasmic reticulum. Science. 1990 Apr 20;248(4953):372–376. doi: 10.1126/science.2158146. [DOI] [PubMed] [Google Scholar]
- Li Z., Nicoll D. A., Collins A., Hilgemann D. W., Filoteo A. G., Penniston J. T., Weiss J. N., Tomich J. M., Philipson K. D. Identification of a peptide inhibitor of the cardiac sarcolemmal Na(+)-Ca2+ exchanger. J Biol Chem. 1991 Jan 15;266(2):1014–1020. [PubMed] [Google Scholar]
- London B., Krueger J. W. Contraction in voltage-clamped, internally perfused single heart cells. J Gen Physiol. 1986 Oct;88(4):475–505. doi: 10.1085/jgp.88.4.475. [DOI] [PMC free article] [PubMed] [Google Scholar]
- Nicoll D. A., Longoni S., Philipson K. D. Molecular cloning and functional expression of the cardiac sarcolemmal Na(+)-Ca2+ exchanger. Science. 1990 Oct 26;250(4980):562–565. doi: 10.1126/science.1700476. [DOI] [PubMed] [Google Scholar]
- O'Neill S. C., Valdeolmillos M., Lamont C., Donoso P., Eisner D. A. The contribution of Na-Ca exchange to relaxation in mammalian cardiac muscle. Ann N Y Acad Sci. 1991;639:444–452. doi: 10.1111/j.1749-6632.1991.tb17331.x. [DOI] [PubMed] [Google Scholar]
- Schouten V. J., ter Keurs H. E. Role of Ica and Na+/Ca2+ exchange in the force-frequency relationship of rat heart muscle. J Mol Cell Cardiol. 1991 Sep;23(9):1039–1050. doi: 10.1016/0022-2828(91)91639-9. [DOI] [PubMed] [Google Scholar]
- Schouten V. J., ter Keurs H. E. The slow repolarization phase of the action potential in rat heart. J Physiol. 1985 Mar;360:13–25. doi: 10.1113/jphysiol.1985.sp015601. [DOI] [PMC free article] [PubMed] [Google Scholar]
- Sham J. S., Cleemann L., Morad M. Gating of the cardiac Ca2+ release channel: the role of Na+ current and Na(+)-Ca2+ exchange. Science. 1992 Feb 14;255(5046):850–853. doi: 10.1126/science.1311127. [DOI] [PubMed] [Google Scholar]
- Shimoni Y., Clark R. B., Giles W. R. Role of an inwardly rectifying potassium current in rabbit ventricular action potential. J Physiol. 1992 Mar;448:709–727. doi: 10.1113/jphysiol.1992.sp019066. [DOI] [PMC free article] [PubMed] [Google Scholar]
- Spurgeon H. A., Stern M. D., Baartz G., Raffaeli S., Hansford R. G., Talo A., Lakatta E. G., Capogrossi M. C. Simultaneous measurement of Ca2+, contraction, and potential in cardiac myocytes. Am J Physiol. 1990 Feb;258(2 Pt 2):H574–H586. doi: 10.1152/ajpheart.1990.258.2.H574. [DOI] [PubMed] [Google Scholar]
- Spurgeon H. A., duBell W. H., Stern M. D., Sollott S. J., Ziman B. D., Silverman H. S., Capogrossi M. C., Talo A., Lakatta E. G. Cytosolic calcium and myofilaments in single rat cardiac myocytes achieve a dynamic equilibrium during twitch relaxation. J Physiol. 1992 Feb;447:83–102. doi: 10.1113/jphysiol.1992.sp018992. [DOI] [PMC free article] [PubMed] [Google Scholar]
- Steadman B. W., Moore K. B., Spitzer K. W., Bridge J. H. A video system for measuring motion in contracting heart cells. IEEE Trans Biomed Eng. 1988 Apr;35(4):264–272. doi: 10.1109/10.1375. [DOI] [PubMed] [Google Scholar]
- Trautwein W., McDonald T. F., Tripathi O. Calcium conductance and tension in mammalian ventricular muscle. Pflugers Arch. 1975;354(1):55–74. doi: 10.1007/BF00584503. [DOI] [PubMed] [Google Scholar]
- Valdeolmillos M., O'Neill S. C., Smith G. L., Eisner D. A. Calcium-induced calcium release activates contraction in intact cardiac cells. Pflugers Arch. 1989 Apr;413(6):676–678. doi: 10.1007/BF00581820. [DOI] [PubMed] [Google Scholar]