Abstract
The involvement of metabolic energy in platelet responses was investigated by measuring the energy consumption during aggregation and secretion from dense, alpha- and acid-hydrolase-containing granules. Gel-filtered human platelets were stimulated with different amounts of thrombin (0.05-5.0 units X ml-1). At various stages during aggregation and secretion the energy consumption was measured from the changes in metabolic ATP and ADP following abrupt arrest of ATP resynthesis. Stimulation with 5 units of thrombin X ml-1 increased the energy consumption from 6.2 +/- 0.9 to 17.8 +/- 0.4 mumol of ATPeq. X min-1 X (10(11) platelets)-1 during the first 15 s. It decreased thereafter and returned to values found in resting cells after about 30 s. With 0.05 unit of thrombin X ml-1, the energy consumption accelerated more slowly and took at least 3 min before it normalized. A strong positive correlation was found between the velocities of the three secretion responses and the concurrent energy consumption (a) at different stages of the responses induced by a given dose of thrombin, and (b) at different secretion velocities initiated by different amounts of thrombin. When, at different stages of the responses, the extent of secretion was compared with the amount of energy that had been consumed, a strong linear correlation was found with the increment in energy consumption but not with the total energy consumption. This correlation was independent of the concentration of thrombin and indicated that complete secretion from dense, alpha- and acid-hydrolase-containing granules was paralleled by an increment of 4.0, 6.5 and 6.7 mumol of ATPeq. X (10(11) platelets)-1, respectively. An energy cost of 0.7 mumol of ATPeq. X (10(11) platelets)-1 was calculated for separate dense-granule secretion, whereas the combined alpha- and acid-hydrolase granule secretion required 5.3 mumol of ATPeq. X (10(11) platelets)-1. There was no correlation between energy consumption and optical aggregation. In contrast, the rate of single platelet disappearance, which is a measure for the early formation of small aggregates, correlated closely with the rate of energy consumption. Compared with secretion, however, the energy requirement of single platelet disappearance was minor, since 2mM-EDTA completely prevented this response but decreased the energy consumption only slightly. An increase of 0.5-1.0 mumol of ATPeq. X (10(11) platelets)-1 was seen before single platelet disappearance and the three secretion responses were initiated, indicating an increase in energy consuming processes that preceded these responses.(ABSTRACT TRUNCATED AT 400 WORDS)
Full text
PDF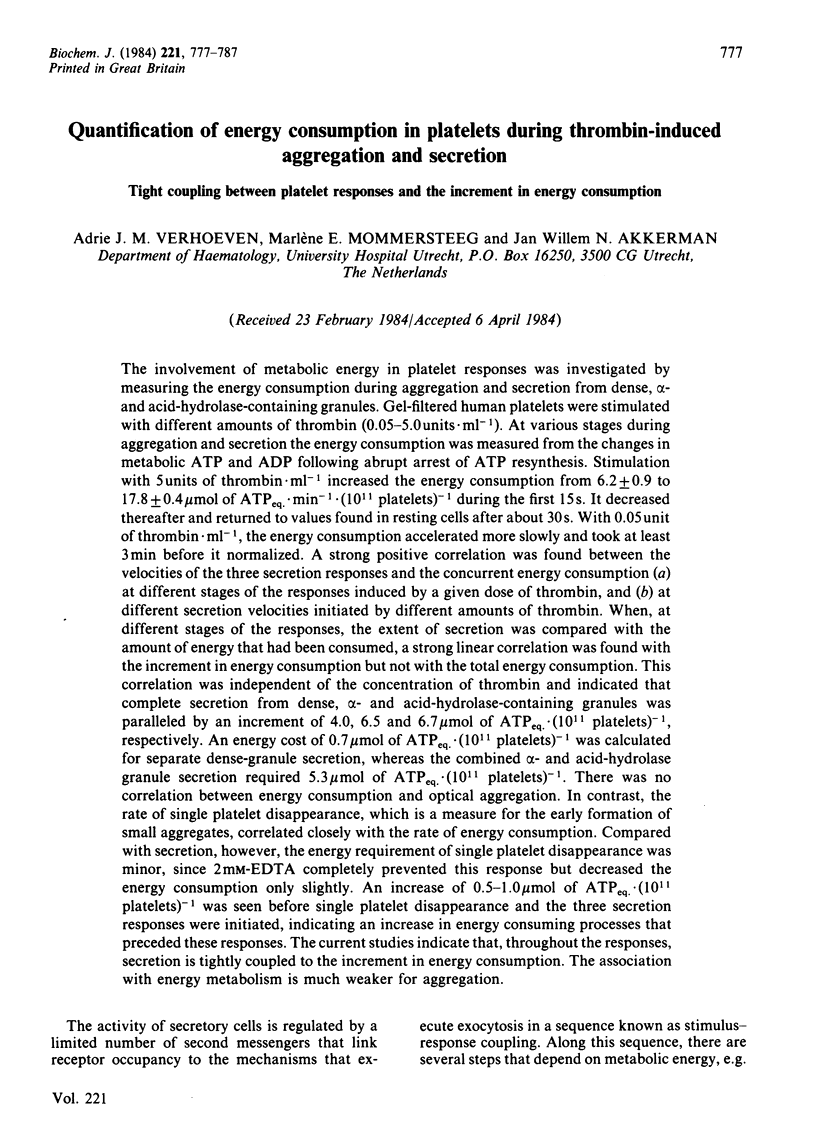
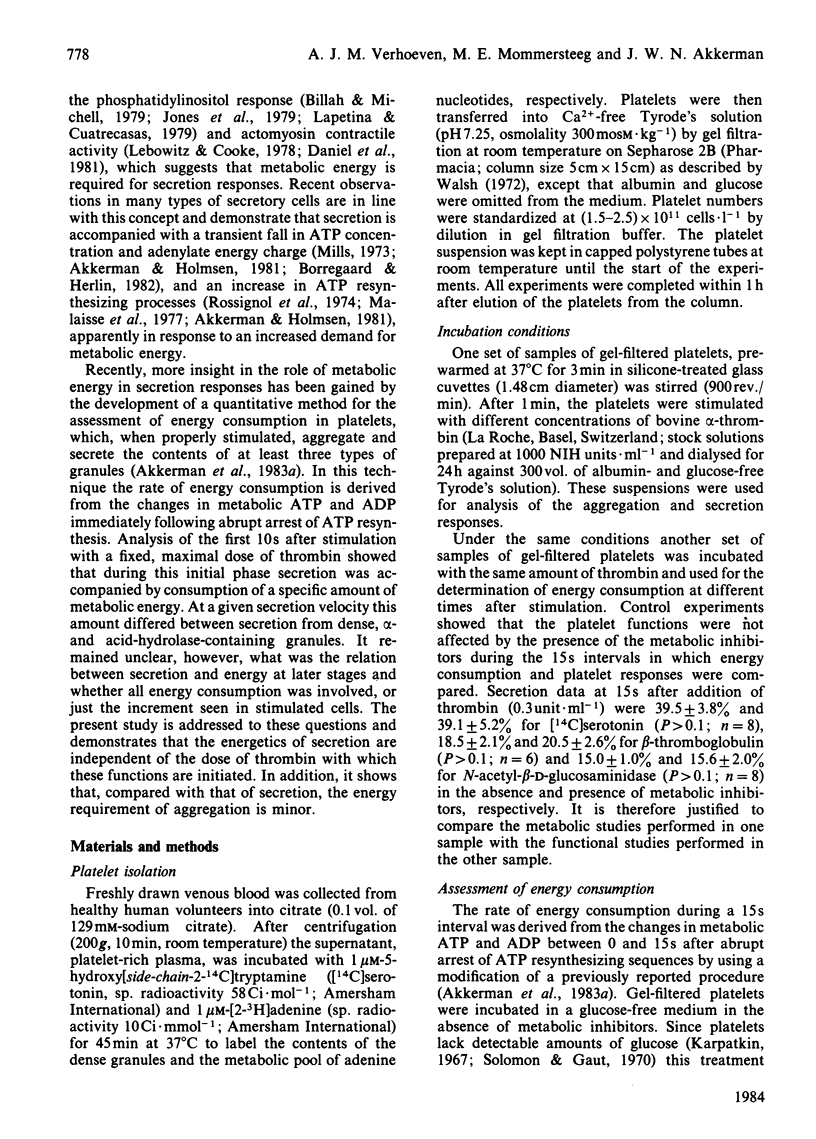
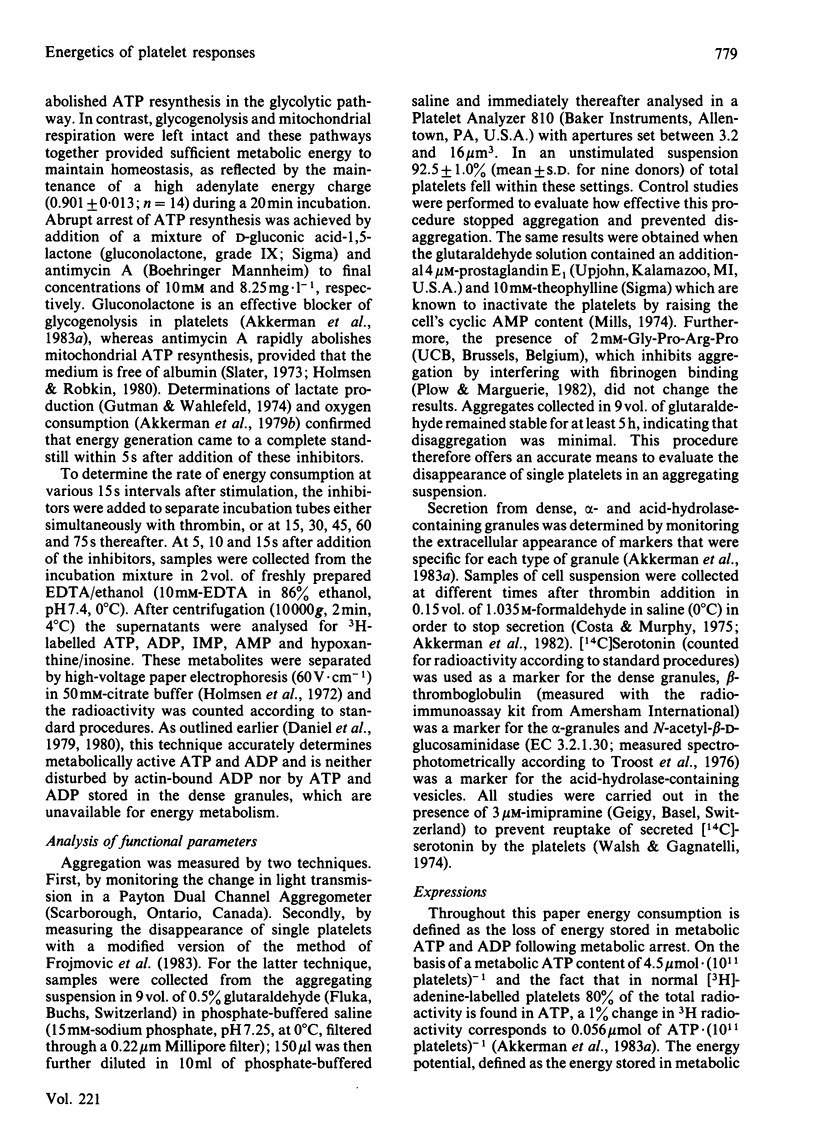
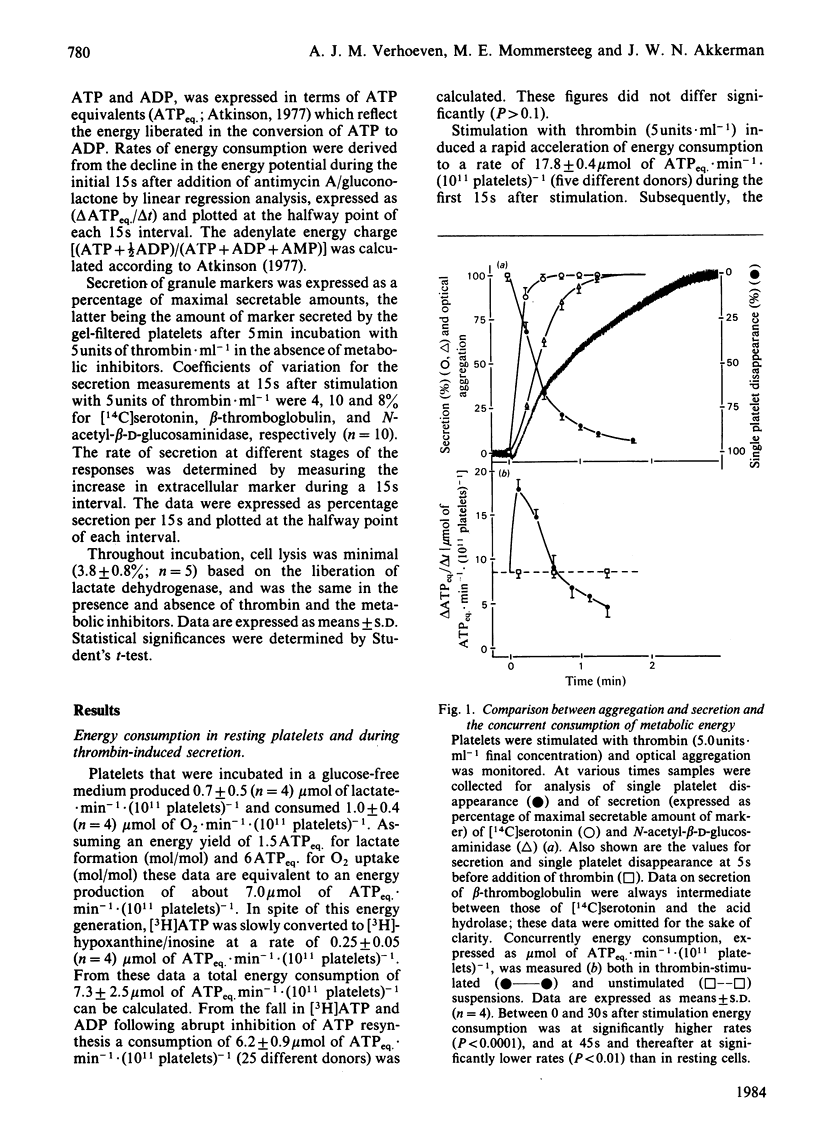
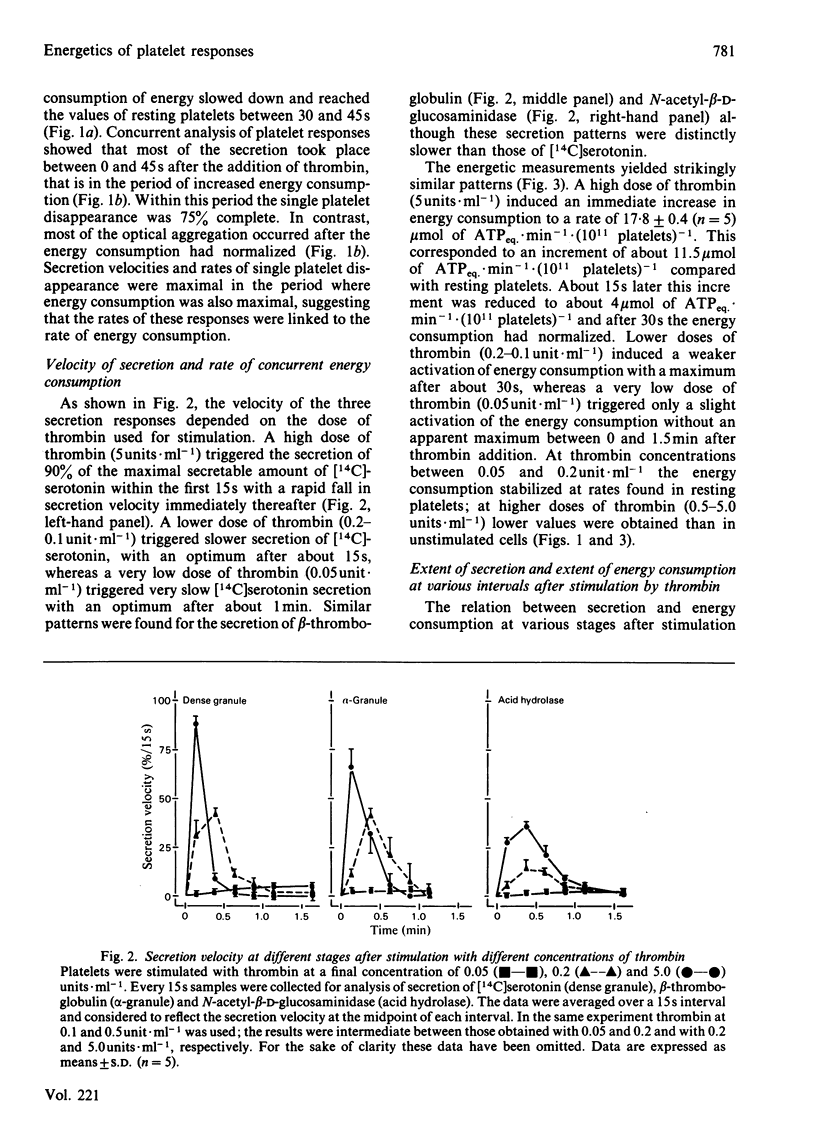
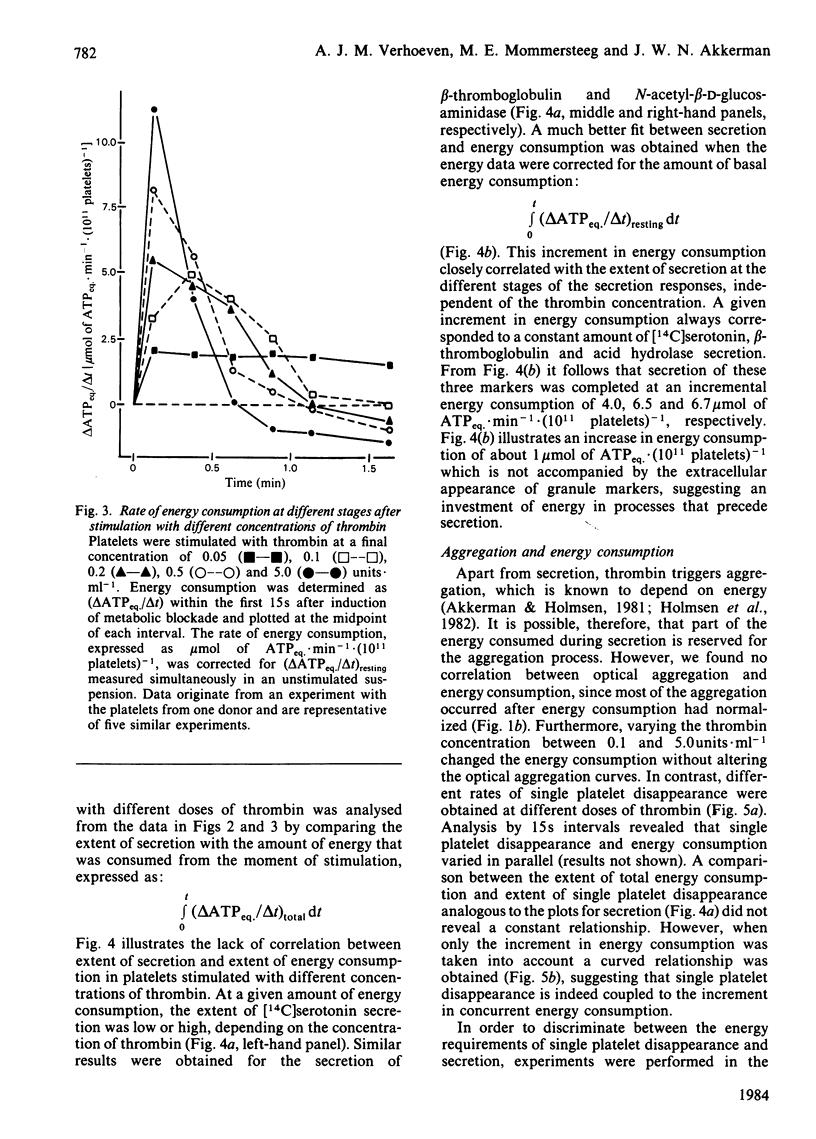
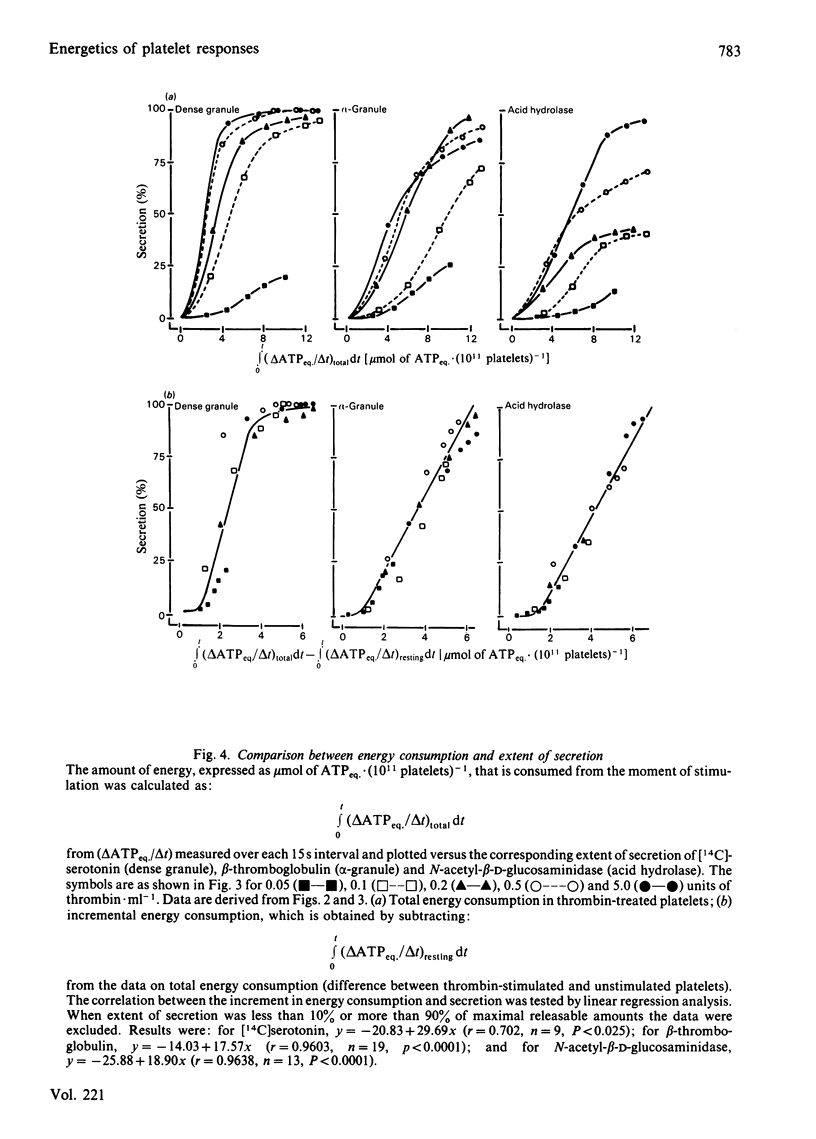
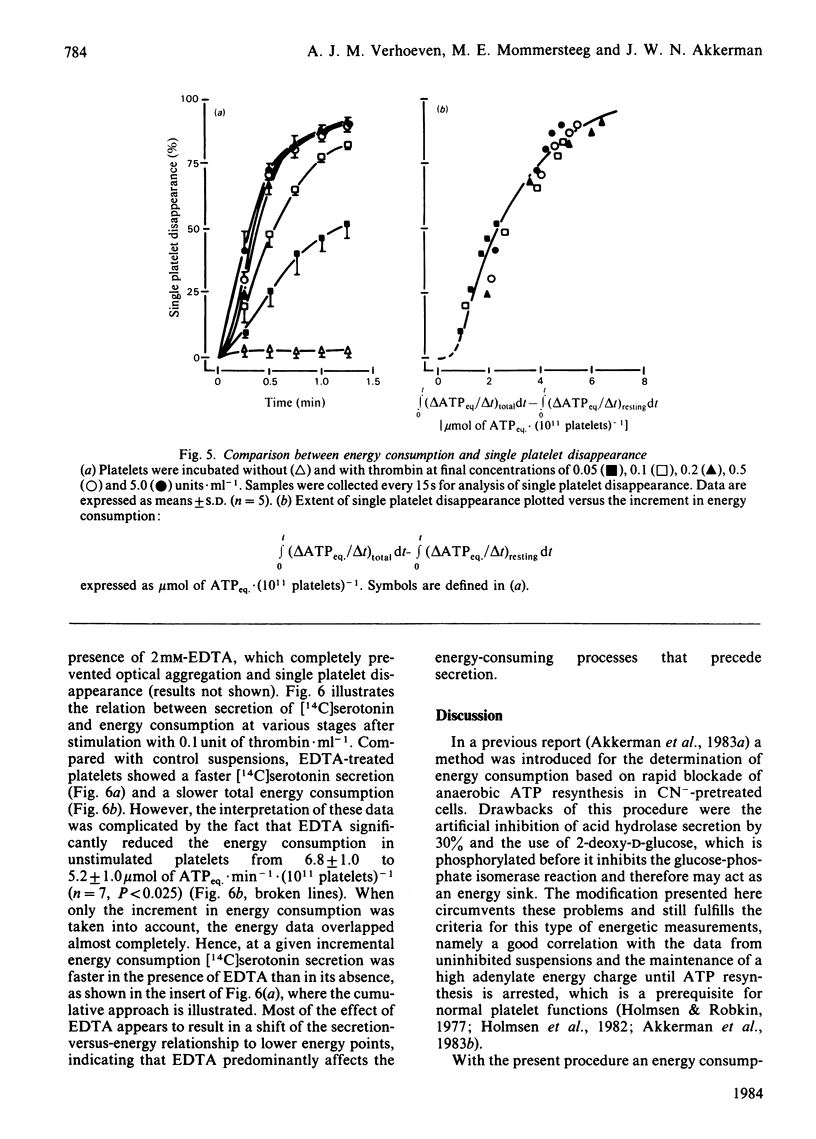
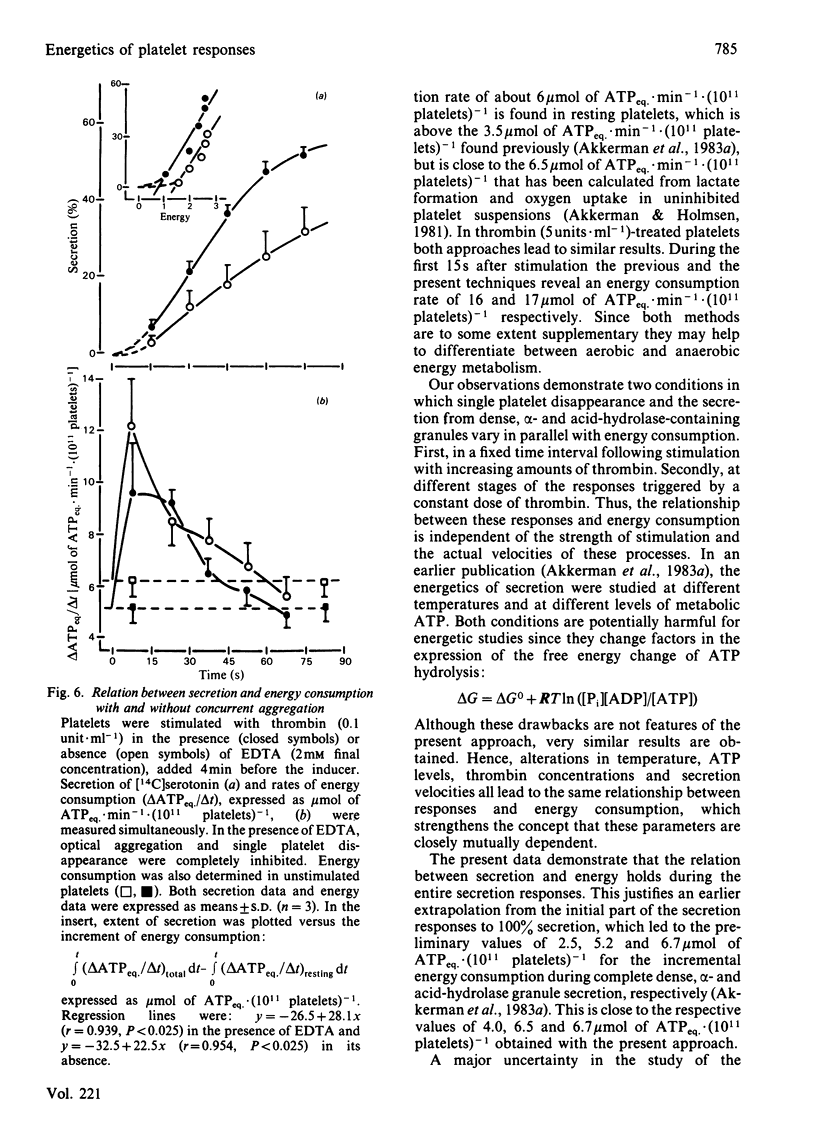
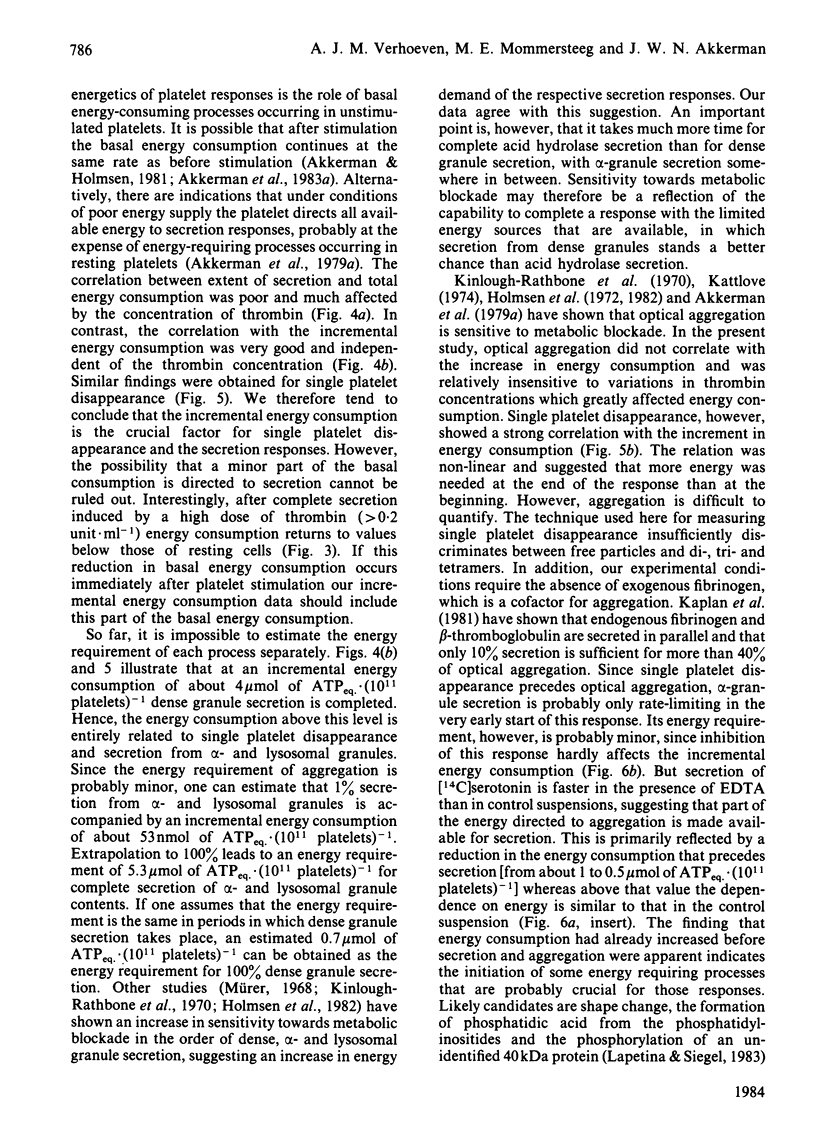
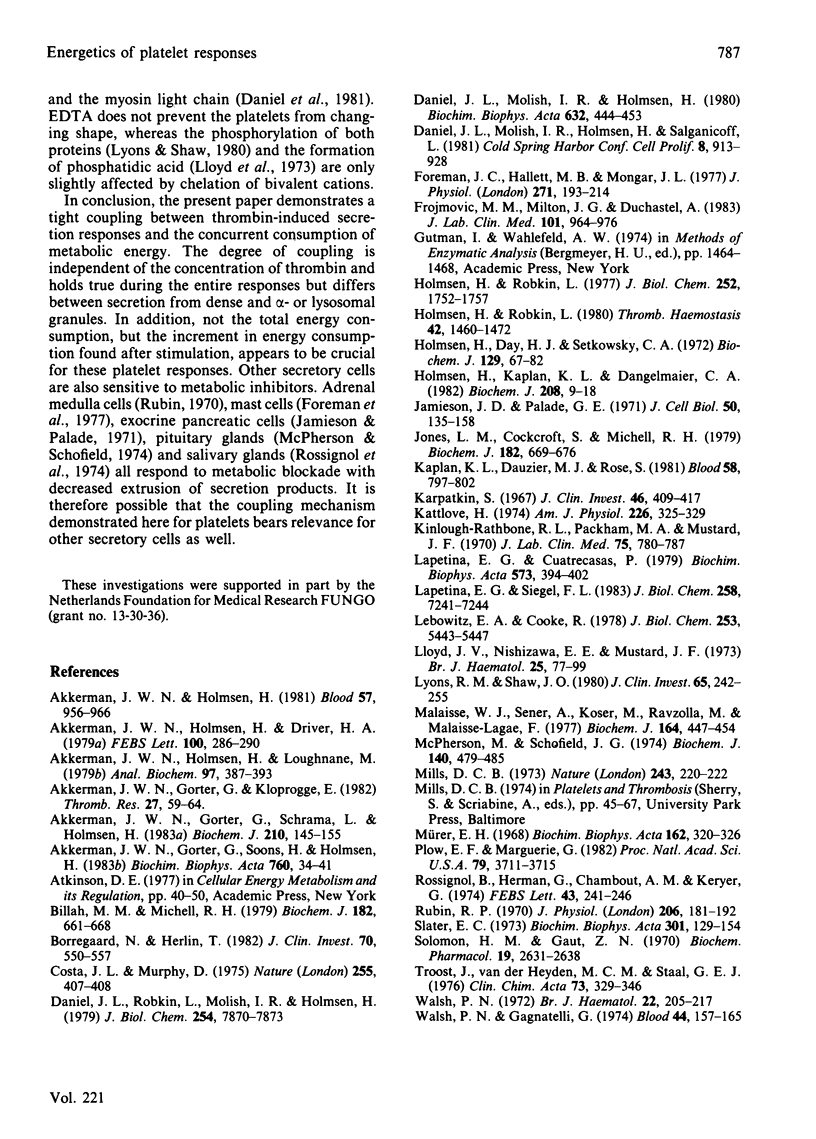
Selected References
These references are in PubMed. This may not be the complete list of references from this article.
- Akkerman J. W., Gorter G., Kloprogge E. Kinetic analysis of alpha-granule secretion by platelets. A methodological report. Thromb Res. 1982 Jul 1;27(1):59–64. doi: 10.1016/0049-3848(82)90278-x. [DOI] [PubMed] [Google Scholar]
- Akkerman J. W., Gorter G., Schrama L., Holmsen H. A novel technique for rapid determination of energy consumption in platelets. Demonstration of different energy consumption associated with three secretory responses. Biochem J. 1983 Jan 15;210(1):145–155. doi: 10.1042/bj2100145. [DOI] [PMC free article] [PubMed] [Google Scholar]
- Akkerman J. W., Gorter G., Soons H., Holmsen H. Close correlation between platelet responses and adenylate energy charge during transient substrate depletion. Biochim Biophys Acta. 1983 Oct 4;760(1):34–41. doi: 10.1016/0304-4165(83)90121-6. [DOI] [PubMed] [Google Scholar]
- Akkerman J. W., Holmsen H., Driver H. A. Platelet aggregation and Ca2+ secretion are independent of simultaneous ATP production. FEBS Lett. 1979 Apr 15;100(2):286–290. doi: 10.1016/0014-5793(79)80353-1. [DOI] [PubMed] [Google Scholar]
- Akkerman J. W., Holmsen H. Interrelationships among platelet responses: studies on the burst in proton liberation, lactate production, and oxygen uptake during platelet aggregation and Ca2+ secretion. Blood. 1981 May;57(5):956–966. [PubMed] [Google Scholar]
- Akkerman J. W., Holmsen H., Loughnane M. Simultaneous measurement of aggregation, secretion, oxygen uptake, proton production, and intracellular metabolites in the same platelet suspension. Anal Biochem. 1979 Sep 1;97(2):387–393. doi: 10.1016/0003-2697(79)90090-3. [DOI] [PubMed] [Google Scholar]
- Billah M. M., Michell R. H. Phosphatidylinositol metabolism in rat hepatocytes stimulated by glycogenolytic hormones. Effects of angiotensin, vasopressin, adrenaline, ionophore A23187 and calcium-ion deprivation. Biochem J. 1979 Sep 15;182(3):661–668. doi: 10.1042/bj1820661. [DOI] [PMC free article] [PubMed] [Google Scholar]
- Borregaard N., Herlin T. Energy metabolism of human neutrophils during phagocytosis. J Clin Invest. 1982 Sep;70(3):550–557. doi: 10.1172/JCI110647. [DOI] [PMC free article] [PubMed] [Google Scholar]
- Costa J. L., Murphy D. L. Platelet 5-HT uptake and release stopped rapidly by formaldehyde. Nature. 1975 May 29;255(5507):407–408. doi: 10.1038/255407a0. [DOI] [PubMed] [Google Scholar]
- Daniel J. L., Molish I. R., Holmsen H. Radioactive labeling of the adenine nucleotide pool of cells as a method to distinguish among intracellular compartments. Studies on human platelets. Biochim Biophys Acta. 1980 Oct 15;632(3):444–453. doi: 10.1016/0304-4165(80)90240-8. [DOI] [PubMed] [Google Scholar]
- Daniel J. L., Robkin L., Molish I. R., Holmsen H. Determination of the ADP concentration available to participate in energy metabolism in an actin-rich cell, the platelet. J Biol Chem. 1979 Aug 25;254(16):7870–7873. [PubMed] [Google Scholar]
- Foreman J. C., Hallett M. B., Mongar J. L. The relationship between histamine secretion and 45calcium uptake by mast cells. J Physiol. 1977 Sep;271(1):193–214. doi: 10.1113/jphysiol.1977.sp011996. [DOI] [PMC free article] [PubMed] [Google Scholar]
- Frojmovic M. M., Milton J. G., Duchastel A. Microscopic measurements of platelet aggregation reveal a low ADP-dependent process distinct from turbidometrically measured aggregation. J Lab Clin Med. 1983 Jun;101(6):964–976. [PubMed] [Google Scholar]
- Holmsen H., Day H. J., Setkowsky C. A. Secretory mechanisms. Behaviour of adenine nucleotides during the platelet release reaction induced by adenosine diphosphate and adrenaline. Biochem J. 1972 Aug;129(1):67–82. doi: 10.1042/bj1290067. [DOI] [PMC free article] [PubMed] [Google Scholar]
- Holmsen H., Kaplan K. L., Dangelmaier C. A. Differential energy requirements for platelet responses. A simultaneous study of aggregation, three secretory processes, arachidonate liberation, phosphatidylinositol breakdown and phosphatidate production. Biochem J. 1982 Oct 15;208(1):9–18. doi: 10.1042/bj2080009. [DOI] [PMC free article] [PubMed] [Google Scholar]
- Holmsen H., Robkin L. Effects of antimycin A and 2-deoxyglucose on energy metabolism in washed human platelets. Thromb Haemost. 1980 Feb 29;42(5):1460–1472. [PubMed] [Google Scholar]
- Holmsen H., Robkin L. Hydrogen peroxide lowers ATP levels in platelets without altering adenyalte energy charge and platelet function. J Biol Chem. 1977 Mar 10;252(5):1752–1757. [PubMed] [Google Scholar]
- Jamieson J. D., Palade G. E. Synthesis, intracellular transport, and discharge of secretory proteins in stimulated pancreatic exocrine cells. J Cell Biol. 1971 Jul;50(1):135–158. doi: 10.1083/jcb.50.1.135. [DOI] [PMC free article] [PubMed] [Google Scholar]
- Jones L. M., Cockcroft S., Michell R. H. Stimulation of phosphatidylinositol turnover in various tissues by cholinergic and adrenergic agonists, by histamine and by caerulein. Biochem J. 1979 Sep 15;182(3):669–676. doi: 10.1042/bj1820669. [DOI] [PMC free article] [PubMed] [Google Scholar]
- Kaplan K. L., Dauzier M. J., Rose S. ADP and epinephrine-induced release of platelet fibrinogen. Blood. 1981 Oct;58(4):797–802. [PubMed] [Google Scholar]
- Karpatkin S. Studies on human platelet glycolysis. Effect of glucose, cyanide, insulin, citrate, and agglutination and contraction on platelet glycolysis. J Clin Invest. 1967 Mar;46(3):409–417. doi: 10.1172/JCI105542. [DOI] [PMC free article] [PubMed] [Google Scholar]
- Kattlove H. E. Platelet ATP in ADP-induced aggregation. Am J Physiol. 1974 Feb;226(2):325–329. doi: 10.1152/ajplegacy.1974.226.2.325. [DOI] [PubMed] [Google Scholar]
- Kinlough-Rathbone R. L., Packham M. A., Mustard J. F. The effect of glucose on adenosine diphosphate-induced platelet aggregation. J Lab Clin Med. 1970 May;75(5):780–787. [PubMed] [Google Scholar]
- Lapetina E. G., Cuatrecasas P. Stimulation of phosphatidic acid production in platelets precedes the formation of arachidonate and parallels the release of serotonin. Biochim Biophys Acta. 1979 May 25;573(2):394–402. doi: 10.1016/0005-2760(79)90072-9. [DOI] [PubMed] [Google Scholar]
- Lapetina E. G., Siegel F. L. Shape change induced in human platelets by platelet-activating factor. Correlation with the formation of phosphatidic acid and phosphorylation of a 40,000-dalton protein. J Biol Chem. 1983 Jun 25;258(12):7241–7244. [PubMed] [Google Scholar]
- Lebowitz E. A., Cooke R. Contractile properties of actomyosin from human blood platelets. J Biol Chem. 1978 Aug 10;253(15):5443–5447. [PubMed] [Google Scholar]
- Lloyd J. V., Nishizawa E. E., Mustard J. F. Effect of ADP-induced shape change on incorporation of 32P into platelet phosphatidic acid and mono-, di- and triphosphatidyl inositol. Br J Haematol. 1973 Jul;25(1):77–99. doi: 10.1111/j.1365-2141.1973.tb01718.x. [DOI] [PubMed] [Google Scholar]
- Lyons R. M., Shaw J. O. Interaction of Ca2+ and protein phosphorylation in the rabbit platelet release reaction. J Clin Invest. 1980 Feb;65(2):242–255. doi: 10.1172/JCI109666. [DOI] [PMC free article] [PubMed] [Google Scholar]
- Malaisse W. J., Sener A., Koser M., Ravazzola M., Malaisse-Lagae F. The stimulus-secretion coupling of glucose-induced insulin release. Insulin release due to glycogenolysis in glucose-deprived islets. Biochem J. 1977 May 15;164(2):447–454. doi: 10.1042/bj1640447. [DOI] [PMC free article] [PubMed] [Google Scholar]
- McPherson M., Schofield J. G. Requirement for adenosine triphosphate for stimulation in vitro of ox growth-hormone release. Biochem J. 1974 Jun;140(3):479–485. doi: 10.1042/bj1400479. [DOI] [PMC free article] [PubMed] [Google Scholar]
- Mills D. C. Changes in the adenylate energy charge in human blood platelets induced by adenosine diphosphate. Nat New Biol. 1973 Jun 13;243(128):220–222. doi: 10.1038/newbio243220a0. [DOI] [PubMed] [Google Scholar]
- Mürer E. H. Release reaction and energy metabolism in blood platelets with special reference to the burst in oxygen uptake. Biochim Biophys Acta. 1968 Oct 1;162(3):320–326. doi: 10.1016/0005-2728(68)90118-7. [DOI] [PubMed] [Google Scholar]
- Plow E. F., Marguerie G. Inhibition of fibrinogen binding to human platelets by the tetrapeptide glycyl-L-prolyl-L-arginyl-L-proline. Proc Natl Acad Sci U S A. 1982 Jun;79(12):3711–3715. doi: 10.1073/pnas.79.12.3711. [DOI] [PMC free article] [PubMed] [Google Scholar]
- Rossignol B., Herman G., Chambaut A. M., Keryer G. The calcium ionophore A 23 187 as a probe for studying the role of Ca2+ ions in the mediation of carbachol effects on rat salivary glands: protein secretion and metabolism of phospholipids and glycogen. FEBS Lett. 1974 Jul 15;43(2):241–246. doi: 10.1016/0014-5793(74)81010-0. [DOI] [PubMed] [Google Scholar]
- Rubin R. P. The role of energy metabolism in calcium-evoked secretion from the adrenal medulla. J Physiol. 1970 Jan;206(1):181–192. doi: 10.1113/jphysiol.1970.sp009005. [DOI] [PMC free article] [PubMed] [Google Scholar]
- Slater E. C. The mechanism of action of the respiratory inhibitor, antimycin. Biochim Biophys Acta. 1973 Dec 7;301(2):129–154. doi: 10.1016/0304-4173(73)90002-5. [DOI] [PubMed] [Google Scholar]
- Solomon H. M., Gaut Z. N. Accumulation and metabolism of 2-deoxy-D-glucose-1-14C in the human platelet. Biochem Pharmacol. 1970 Sep;19(9):2631–2638. doi: 10.1016/0006-2952(70)90013-4. [DOI] [PubMed] [Google Scholar]
- Troost J., van der Heijden M. C., Staal G. E. Characterization of alpha-L-fucosidase from two different families with fucosidosis. Clin Chim Acta. 1976 Dec 1;73(2):329–346. doi: 10.1016/0009-8981(76)90180-7. [DOI] [PubMed] [Google Scholar]
- Walsh P. N. Albumin density gradient separation and washing of platelets and the study of platelet coagulant activities. Br J Haematol. 1972 Feb;22(2):205–217. doi: 10.1111/j.1365-2141.1972.tb08801.x. [DOI] [PubMed] [Google Scholar]
- Walsh P. N., Gagnatelli G. Platelet antiheparin activity: storage site and release mechanism. Blood. 1974 Aug;44(2):157–168. [PubMed] [Google Scholar]