Abstract
1. The Km for NAD+ of cholera toxin working as an NAD+ glycohydrolase is 4 mM, and this is increased to about 50 mM in the presence of low-Mr ADP-ribose acceptors. Only molecules having both the adenine and nicotinamide moieties of NAD+ with minor alterations in the nicotinamide ring can be competitive inhibitors of this reaction. 2. This high Km for NAD+ is also reflected in the dissociation constant, Kd, which was determined by a variety of methods. 3. Results from equilibrium dialysis were subject to high error, but showed one binding site and a Kd of about 3 mM. 4. The A1 peptide of the toxin is digested by trypsin, and this digestion is completely prevented by concentrations of NAD+ above 50 mM. Measurement (by densitometric scanning of polyacrylamide-gel electrophoretograms) of the rate of tryptic digestion at different concentrations of NAD+ allowed a more accurate determination of Kd = 4.0 +/- 0.4 mM. Some analogues of NAD+ that are competitive inhibitors of the glycohydrolase reaction also prevented digestion.
Full text
PDF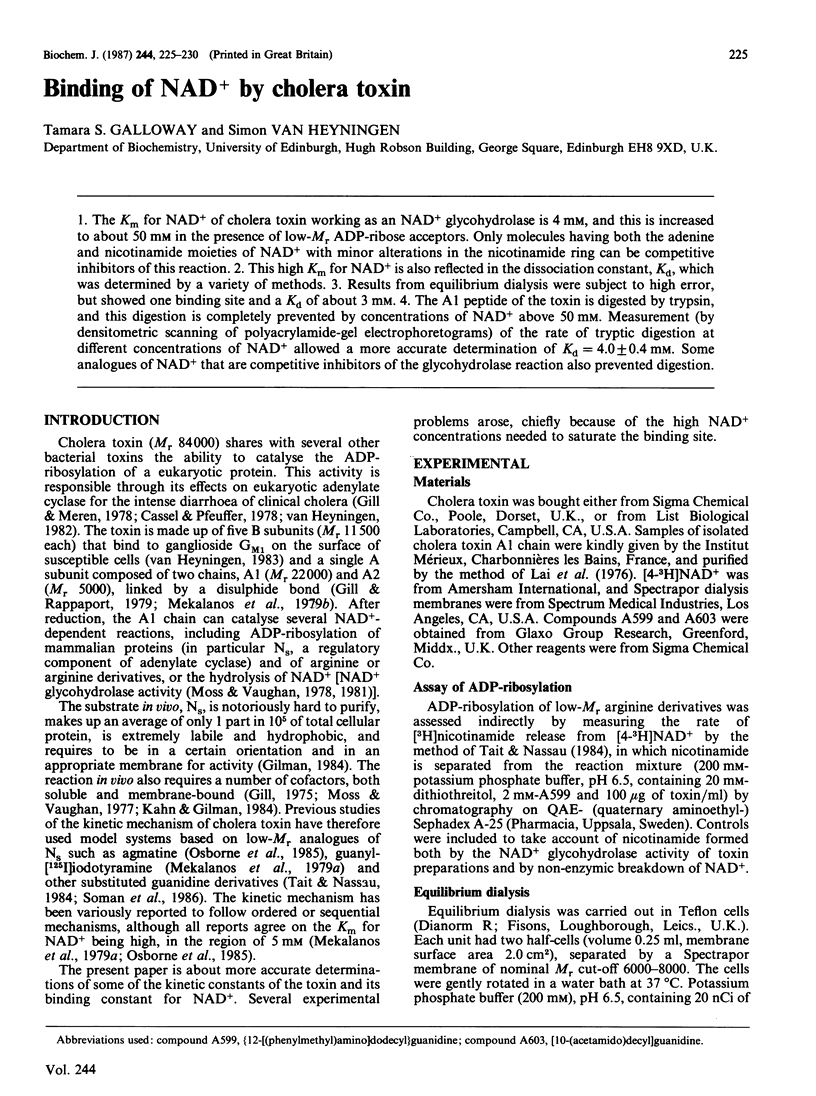
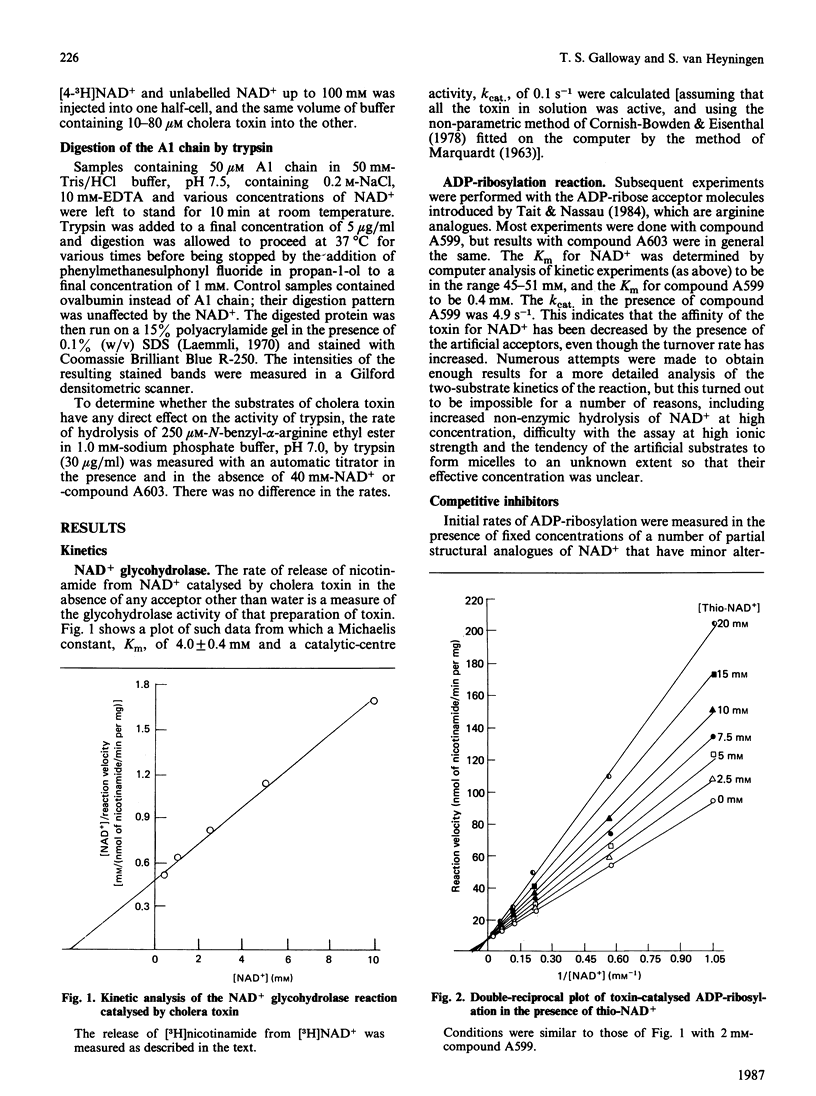
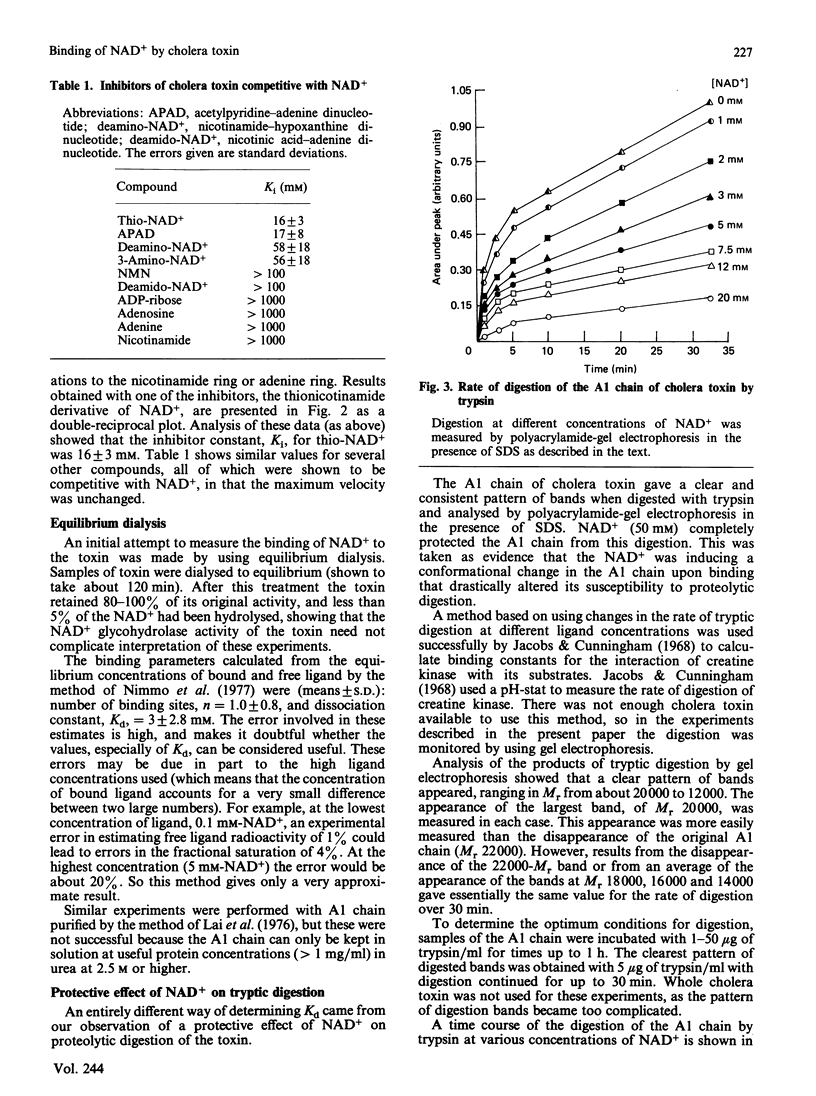
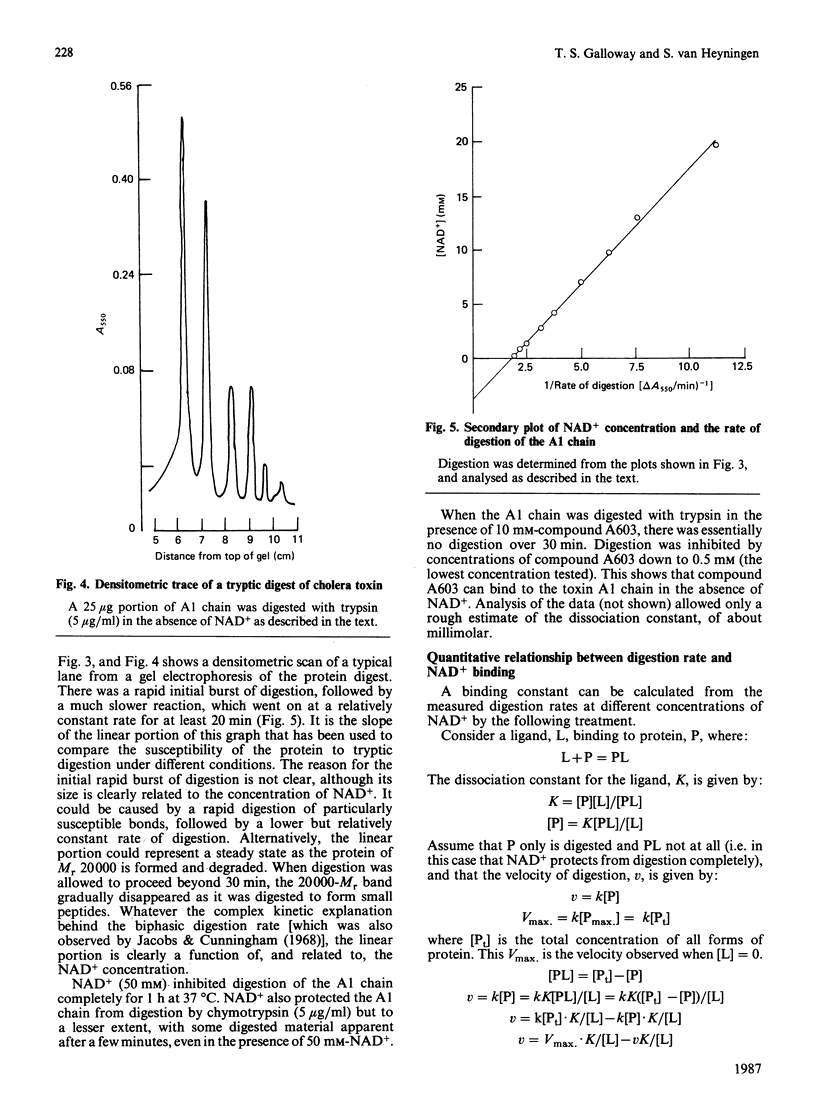
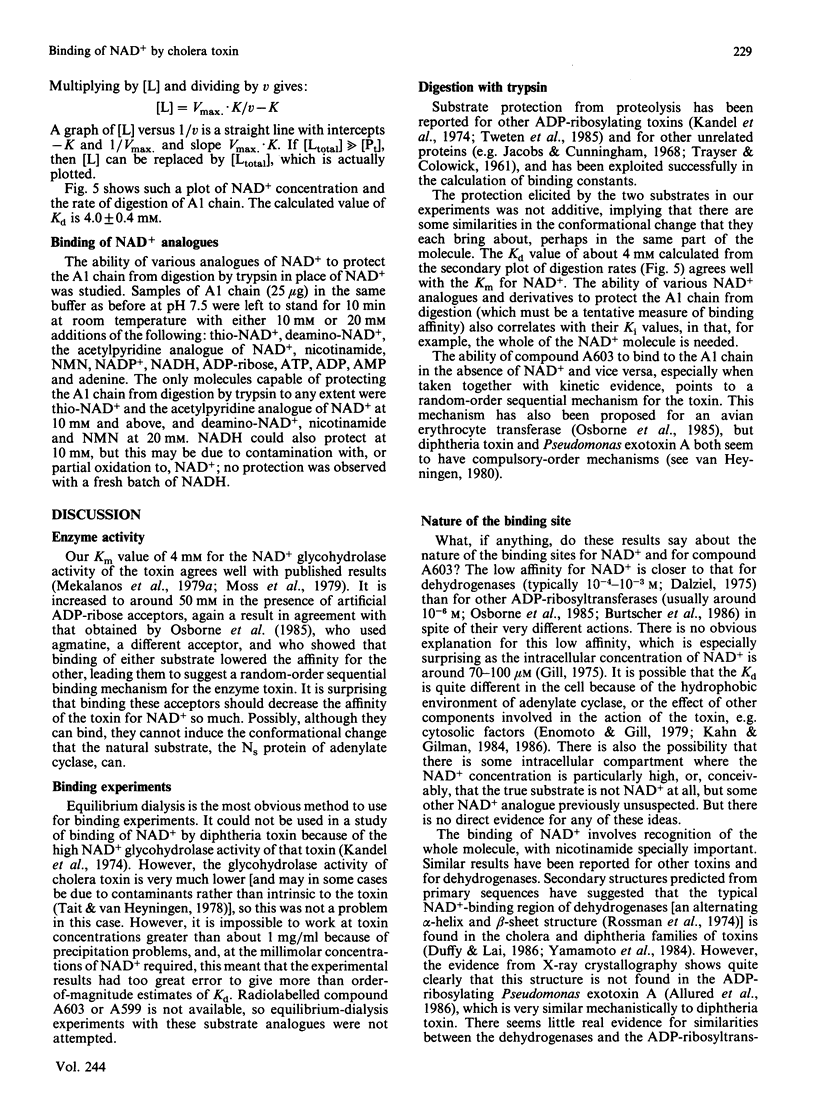
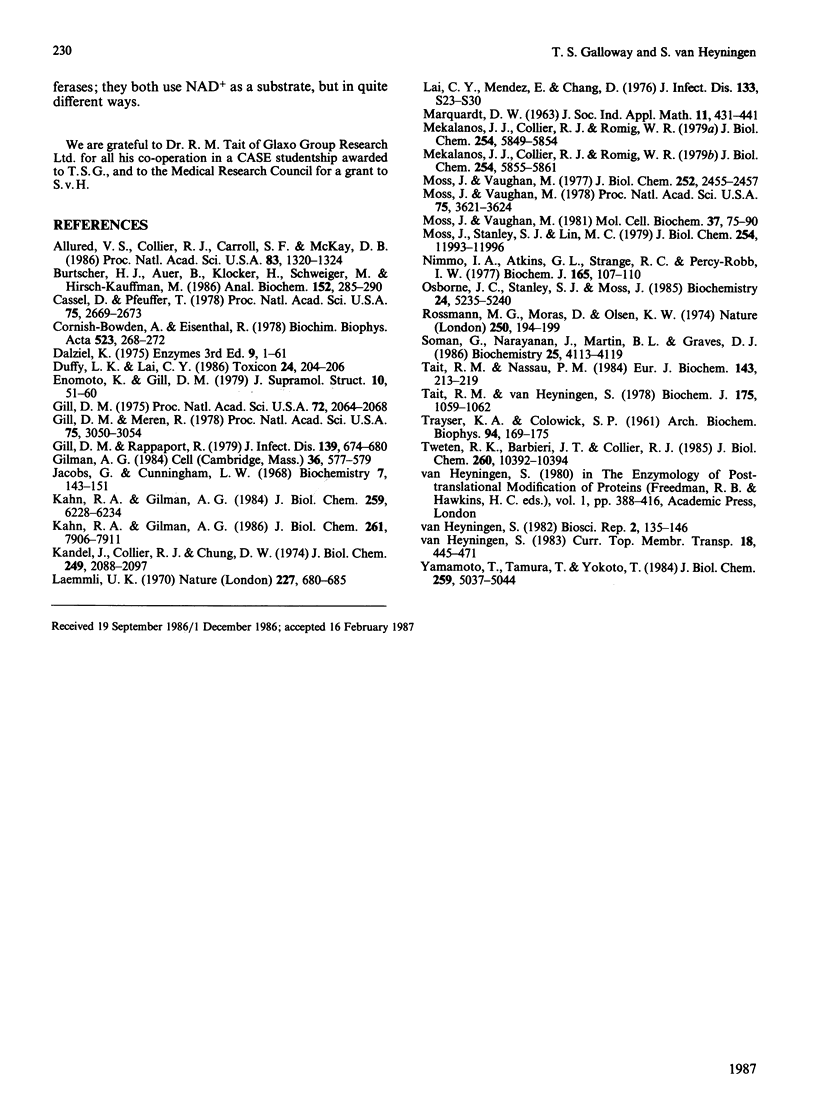
Selected References
These references are in PubMed. This may not be the complete list of references from this article.
- Allured V. S., Collier R. J., Carroll S. F., McKay D. B. Structure of exotoxin A of Pseudomonas aeruginosa at 3.0-Angstrom resolution. Proc Natl Acad Sci U S A. 1986 Mar;83(5):1320–1324. doi: 10.1073/pnas.83.5.1320. [DOI] [PMC free article] [PubMed] [Google Scholar]
- Burtscher H. J., Auer B., Klocker H., Schweiger M., Hirsch-Kauffmann M. Isolation of ADP-ribosyltransferase by affinity chromatography. Anal Biochem. 1986 Feb 1;152(2):285–290. doi: 10.1016/0003-2697(86)90410-0. [DOI] [PubMed] [Google Scholar]
- Cassel D., Pfeuffer T. Mechanism of cholera toxin action: covalent modification of the guanyl nucleotide-binding protein of the adenylate cyclase system. Proc Natl Acad Sci U S A. 1978 Jun;75(6):2669–2673. doi: 10.1073/pnas.75.6.2669. [DOI] [PMC free article] [PubMed] [Google Scholar]
- Cornish-Bowden A., Eisenthal R. Estimation of Michaelis constant and maximum velocity from the direct linear plot. Biochim Biophys Acta. 1978 Mar 14;523(1):268–272. doi: 10.1016/0005-2744(78)90030-x. [DOI] [PubMed] [Google Scholar]
- Duffy L. K., Lai C. Y. A note on the predicted secondary structures of the active chains of cholera and diphtheria toxins. Toxicon. 1986;24(2):204–206. doi: 10.1016/0041-0101(86)90124-8. [DOI] [PubMed] [Google Scholar]
- Enomoto K., Gill D. M. Requirement for guanosine triphosphate in the activation of adenylate cyclase by cholera toxin. J Supramol Struct. 1979;10(1):51–60. doi: 10.1002/jss.400100106. [DOI] [PubMed] [Google Scholar]
- Gill D. M. Involvement of nicotinamide adenine dinucleotide in the action of cholera toxin in vitro. Proc Natl Acad Sci U S A. 1975 Jun;72(6):2064–2068. doi: 10.1073/pnas.72.6.2064. [DOI] [PMC free article] [PubMed] [Google Scholar]
- Gill D. M., Meren R. ADP-ribosylation of membrane proteins catalyzed by cholera toxin: basis of the activation of adenylate cyclase. Proc Natl Acad Sci U S A. 1978 Jul;75(7):3050–3054. doi: 10.1073/pnas.75.7.3050. [DOI] [PMC free article] [PubMed] [Google Scholar]
- Gill D. M., Rappaport R. S. Origin of the enzymatically active A1 fragment of cholera toxin. J Infect Dis. 1979 Jun;139(6):674–680. doi: 10.1093/infdis/139.6.674. [DOI] [PubMed] [Google Scholar]
- Gilman A. G. G proteins and dual control of adenylate cyclase. Cell. 1984 Mar;36(3):577–579. doi: 10.1016/0092-8674(84)90336-2. [DOI] [PubMed] [Google Scholar]
- Jacobs G., Cunningham L. W. Creatine kinase. The relationship of trypsin susceptibility to substrate binding. Biochemistry. 1968 Jan;7(1):143–151. doi: 10.1021/bi00841a019. [DOI] [PubMed] [Google Scholar]
- Kahn R. A., Gilman A. G. Purification of a protein cofactor required for ADP-ribosylation of the stimulatory regulatory component of adenylate cyclase by cholera toxin. J Biol Chem. 1984 May 25;259(10):6228–6234. [PubMed] [Google Scholar]
- Kahn R. A., Gilman A. G. The protein cofactor necessary for ADP-ribosylation of Gs by cholera toxin is itself a GTP binding protein. J Biol Chem. 1986 Jun 15;261(17):7906–7911. [PubMed] [Google Scholar]
- Kandel J., Collier R. J., Chung D. W. Interaction of fragment A from diphtheria toxin with nicotinamide adenine dinucleotide. J Biol Chem. 1974 Apr 10;249(7):2088–2097. [PubMed] [Google Scholar]
- Laemmli U. K. Cleavage of structural proteins during the assembly of the head of bacteriophage T4. Nature. 1970 Aug 15;227(5259):680–685. doi: 10.1038/227680a0. [DOI] [PubMed] [Google Scholar]
- Mekalanos J. J., Collier R. J., Romig W. R. Enzymic activity of cholera toxin. I. New method of assay and the mechanism of ADP-ribosyl transfer. J Biol Chem. 1979 Jul 10;254(13):5849–5854. [PubMed] [Google Scholar]
- Mekalanos J. J., Collier R. J., Romig W. R. Enzymic activity of cholera toxin. II. Relationships to proteolytic processing, disulfide bond reduction, and subunit composition. J Biol Chem. 1979 Jul 10;254(13):5855–5861. [PubMed] [Google Scholar]
- Moss J., Stanley S. J., Lin M. C. NAD glycohydrolase and ADP-ribosyltransferase activities are intrinsic to the A1 peptide of choleragen. J Biol Chem. 1979 Dec 10;254(23):11993–11999. [PubMed] [Google Scholar]
- Moss J., Vaughan M. Isolation of an avian erythrocyte protein possessing ADP-ribosyltransferase activity and capable of activating adenylate cyclase. Proc Natl Acad Sci U S A. 1978 Aug;75(8):3621–3624. doi: 10.1073/pnas.75.8.3621. [DOI] [PMC free article] [PubMed] [Google Scholar]
- Moss J., Vaughan M. Mechanism of action of choleragen and E. coli heat-labile enterotoxin: activation of adenylate cyclase by ADP-ribosylation. Mol Cell Biochem. 1981 Jul 7;37(2):75–90. doi: 10.1007/BF02354931. [DOI] [PubMed] [Google Scholar]
- Moss J., Vaughan M. Mechanism of action of choleragen. Evidence for ADP-ribosyltransferase activity with arginine as an acceptor. J Biol Chem. 1977 Apr 10;252(7):2455–2457. [PubMed] [Google Scholar]
- Nimmo I. A., Atkins G. L., Strange R. C., Percy-Robb I. W. An evaluation of ways of using equilibrium dialysis to quantify the binding of ligand to macromolecule. Biochem J. 1977 Jul 1;165(1):107–110. doi: 10.1042/bj1650107. [DOI] [PMC free article] [PubMed] [Google Scholar]
- Osborne J. C., Jr, Stanley S. J., Moss J. Kinetic mechanisms of two NAD:arginine ADP-ribosyltransferases: the soluble, salt-stimulated transferase from turkey erythrocytes and choleragen, a toxin from Vibrio cholerae. Biochemistry. 1985 Sep 10;24(19):5235–5240. doi: 10.1021/bi00340a042. [DOI] [PubMed] [Google Scholar]
- Rifkind D., Frey J. A., Petersen E. A., Dinowitz M. Delayed hypersensitivity to fungal antigens in mice. II. Molecular classes in immunogenic RNA extracts that transfer delayed hypersensitivity. J Infect Dis. 1976 May;133(5):523–532. doi: 10.1093/infdis/133.5.523. [DOI] [PubMed] [Google Scholar]
- Rossmann M. G., Moras D., Olsen K. W. Chemical and biological evolution of nucleotide-binding protein. Nature. 1974 Jul 19;250(463):194–199. doi: 10.1038/250194a0. [DOI] [PubMed] [Google Scholar]
- Soman G., Narayanan J., Martin B. L., Graves D. J. Use of substituted (benzylidineamino)guanidines in the study of guanidino group specific ADP-ribosyltransferase. Biochemistry. 1986 Jul 15;25(14):4113–4119. doi: 10.1021/bi00362a019. [DOI] [PubMed] [Google Scholar]
- TRAYSER K. A., COLOWICK S. P. Properties of crystalline hexokinase from yeast. III. Studies on glucose-enzyme interaction. Arch Biochem Biophys. 1961 Jul;94:169–176. doi: 10.1016/0003-9861(61)90025-x. [DOI] [PubMed] [Google Scholar]
- Tait R. M., Nassau P. M. Artificial low-molecular-mass substrates of cholera toxin. Eur J Biochem. 1984 Aug 15;143(1):213–219. doi: 10.1111/j.1432-1033.1984.tb08361.x. [DOI] [PubMed] [Google Scholar]
- Tait R. M., van Heyningen S. The adenylate cyclase-activating activity of cholera toxin is not associated with a nicotinamide--adenine dinucleotide glycohydrolase activity. Biochem J. 1978 Sep 15;174(3):1059–1062. doi: 10.1042/bj1741059. [DOI] [PMC free article] [PubMed] [Google Scholar]
- Tweten R. K., Barbieri J. T., Collier R. J. Diphtheria toxin. Effect of substituting aspartic acid for glutamic acid 148 on ADP-ribosyltransferase activity. J Biol Chem. 1985 Sep 5;260(19):10392–10394. [PubMed] [Google Scholar]
- Yamamoto T., Tamura T., Yokota T. Primary structure of heat-labile enterotoxin produced by Escherichia coli pathogenic for humans. J Biol Chem. 1984 Apr 25;259(8):5037–5044. [PubMed] [Google Scholar]
- van Heyningen S. Cholera toxin. Biosci Rep. 1982 Mar;2(3):135–146. doi: 10.1007/BF01116376. [DOI] [PubMed] [Google Scholar]