Abstract
The technique of reversible Ca2+-induced permeabilization [Al Nasser & Crompton (1986) Biochem. J. 239, 19-29, 31-40] has been applied to the preparation of heart mitochondria loaded with the Ca2+ indicator arsenazo III (2 nmol of arsenazo III/mg of mitochondrial protein). The loaded mitochondria ('mitosomes') were used to study the control of the Na+-Ca2+ carrier by extramitochondrial Ca2+ mediated by putative regulatory sites. The Vmax. of the Na+-Ca2+ carrier and the degree of regulatory-site-mediated inhibition were similar to normal heart mitochondria. Ca2+ occupation of the sites in mitosomes yields partial inhibition, which is half-maximal with 0.8 microM external free Ca2+. The inhibition consists of a small decrease in Vmax. and a relatively large increase in apparent Km for internal Ca2+. Mg2+ also appears to interact with the sites, but this is largely abolished by ATP and ADP (but not AMP) under conditions in which the free [Mg2+] is maintained constant. The results indicate that the regulatory sites are effective in controlling the Na+-Ca2+ carrier at physiological concentrations of adenine nucleotides, Mg2+, intra- and extra-mitochondrial free Ca2+.
Full text
PDF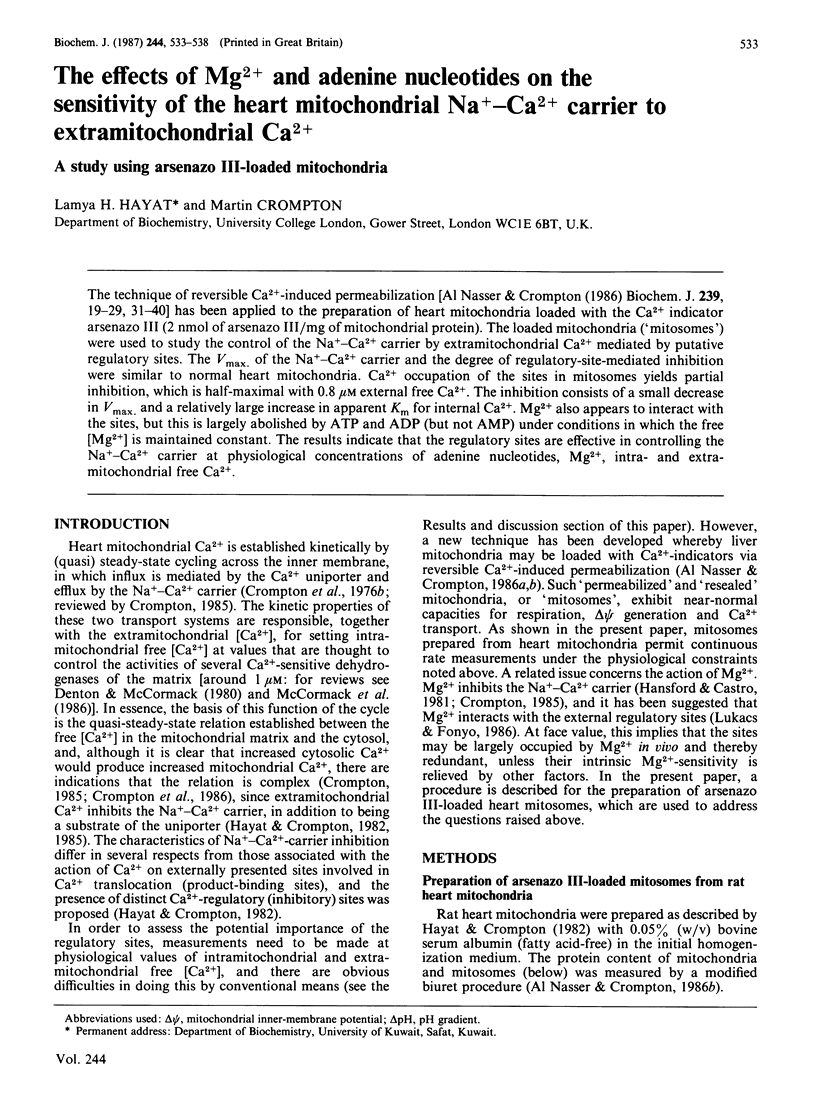
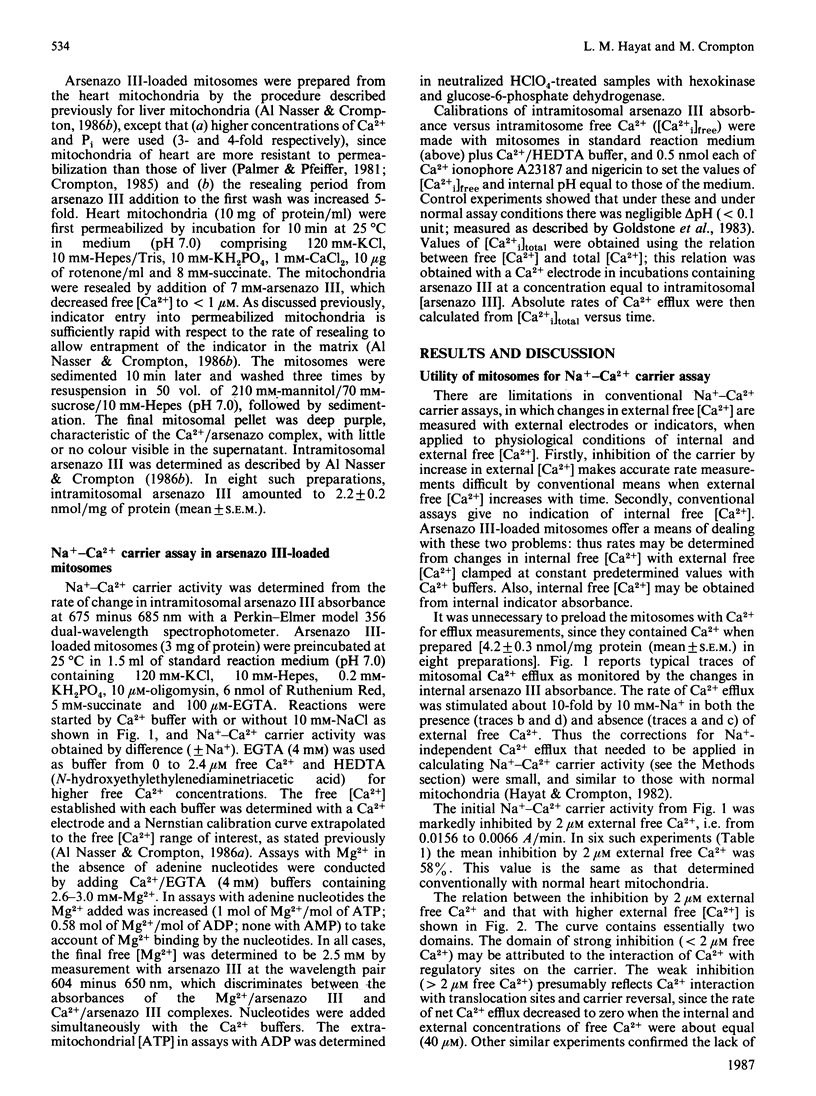
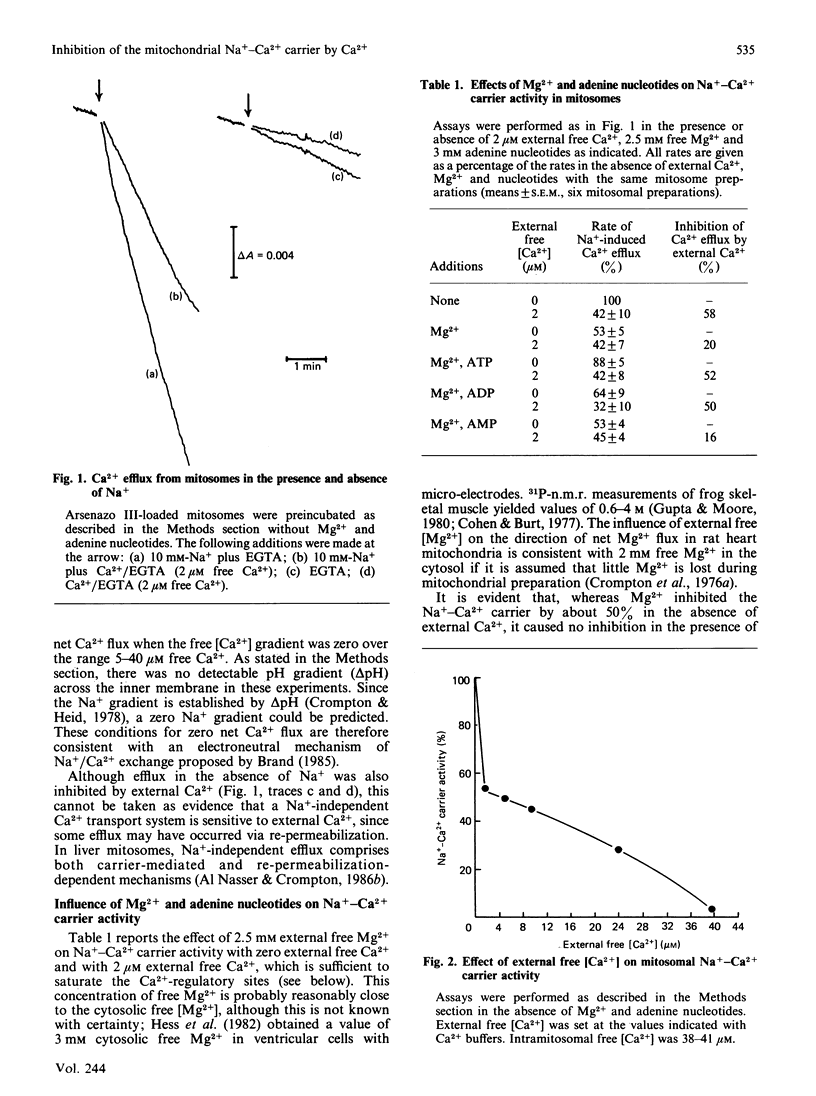
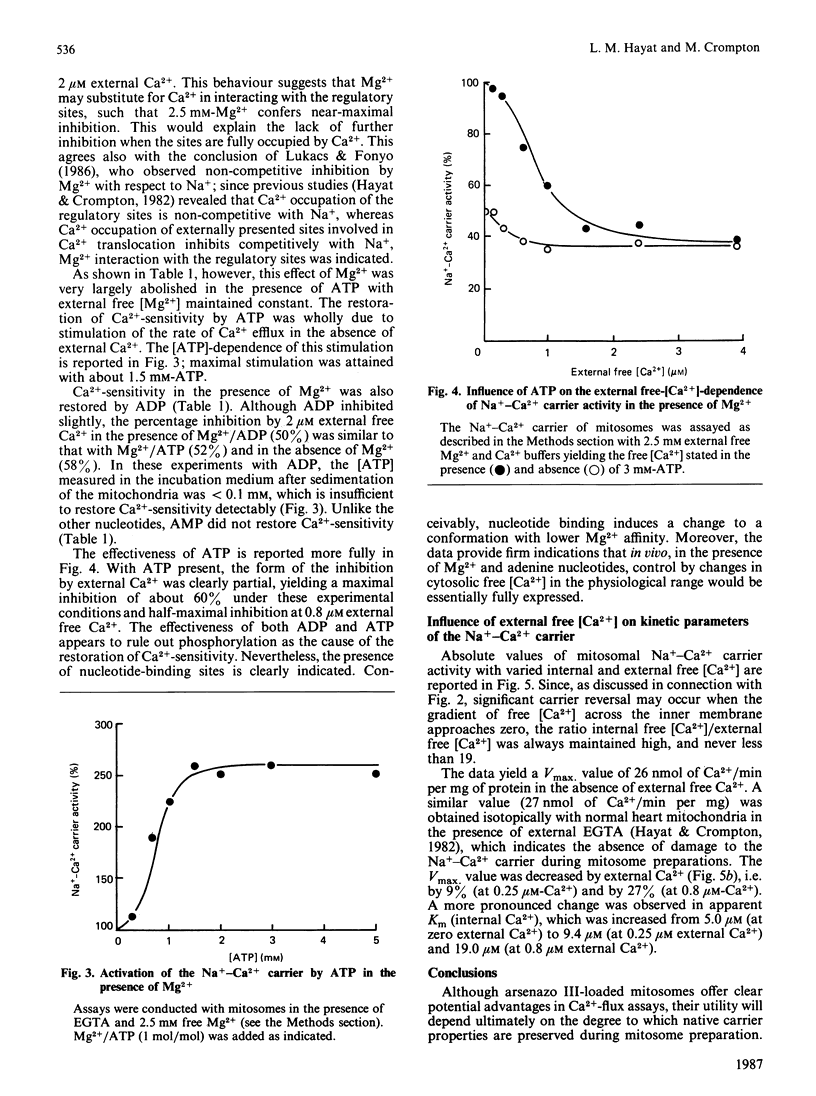
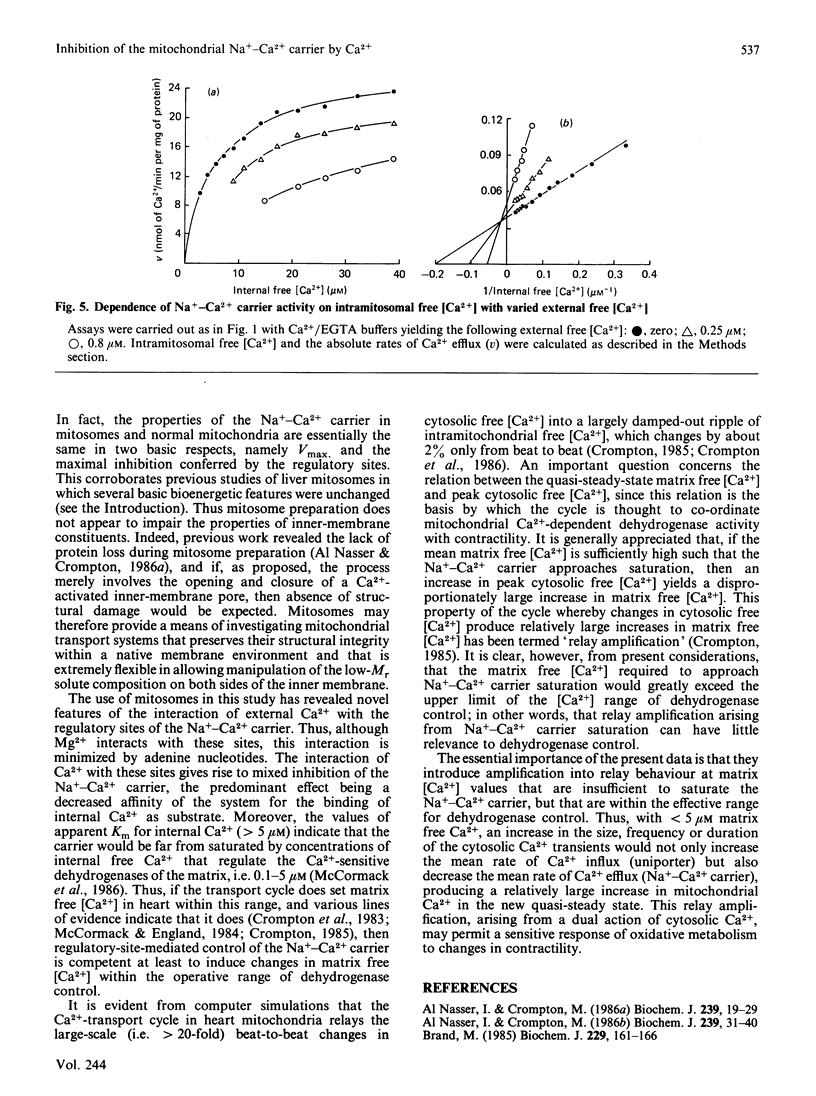
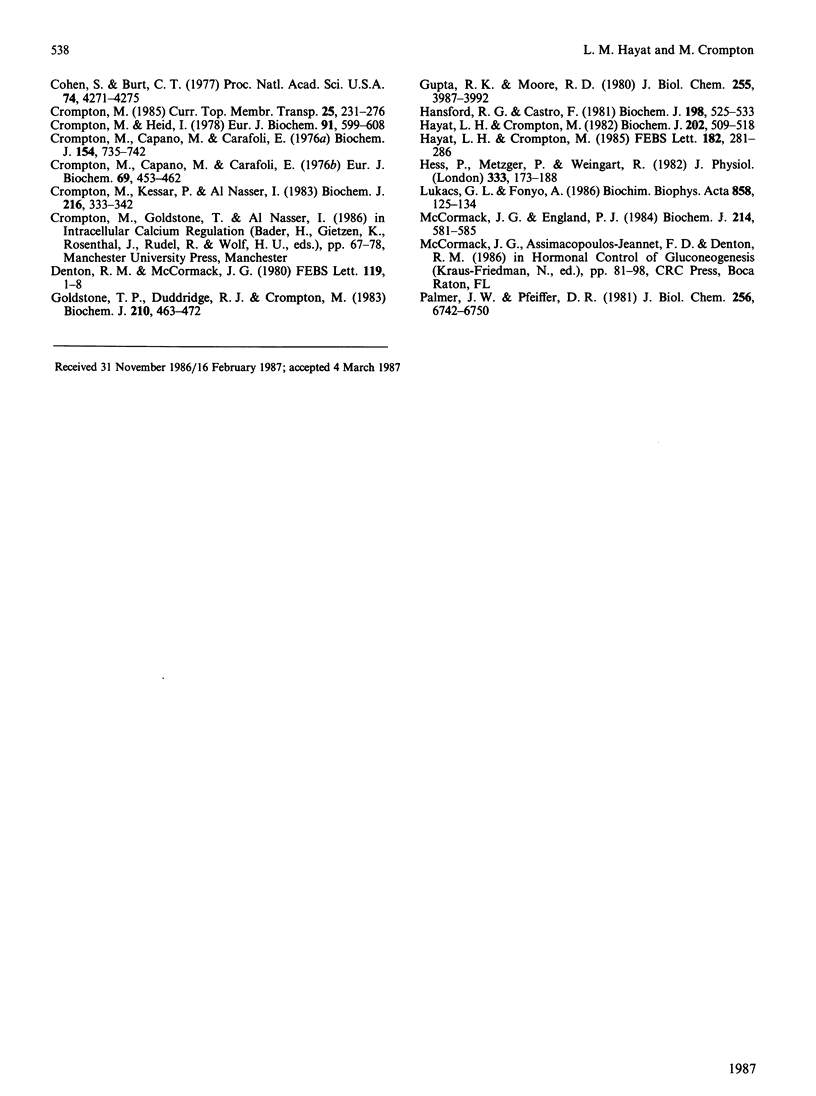
Selected References
These references are in PubMed. This may not be the complete list of references from this article.
- Al-Nasser I., Crompton M. The entrapment of the Ca2+ indicator arsenazo III in the matrix space of rat liver mitochondria by permeabilization and resealing. Na+-dependent and -independent effluxes of Ca2+ in arsenazo III-loaded mitochondria. Biochem J. 1986 Oct 1;239(1):31–40. doi: 10.1042/bj2390031. [DOI] [PMC free article] [PubMed] [Google Scholar]
- Brand M. D. The stoichiometry of the exchange catalysed by the mitochondrial calcium/sodium antiporter. Biochem J. 1985 Jul 1;229(1):161–166. doi: 10.1042/bj2290161. [DOI] [PMC free article] [PubMed] [Google Scholar]
- Cohen S. M., Burt C. T. 31P nuclear magnetic relaxation studies of phosphocreatine in intact muscle: determination of intracellular free magnesium. Proc Natl Acad Sci U S A. 1977 Oct;74(10):4271–4275. doi: 10.1073/pnas.74.10.4271. [DOI] [PMC free article] [PubMed] [Google Scholar]
- Crompton M., Capano M., Carafoli E. Respiration-dependent efflux of magnesium ions from heart mitochondria. Biochem J. 1976 Mar 15;154(3):735–742. doi: 10.1042/bj1540735. [DOI] [PMC free article] [PubMed] [Google Scholar]
- Crompton M., Heid I. The cycling of calcium, sodium, and protons across the inner membrane of cardiac mitochondria. Eur J Biochem. 1978 Nov 15;91(2):599–608. doi: 10.1111/j.1432-1033.1978.tb12713.x. [DOI] [PubMed] [Google Scholar]
- Crompton M., Kessar P., Al-Nasser I. The alpha-adrenergic-mediated activation of the cardiac mitochondrial Ca2+ uniporter and its role in the control of intramitochondrial Ca2+ in vivo. Biochem J. 1983 Nov 15;216(2):333–342. doi: 10.1042/bj2160333. [DOI] [PMC free article] [PubMed] [Google Scholar]
- Denton R. M., McCormack J. G. On the role of the calcium transport cycle in heart and other mammalian mitochondria. FEBS Lett. 1980 Sep 22;119(1):1–8. doi: 10.1016/0014-5793(80)80986-0. [DOI] [PubMed] [Google Scholar]
- Goldstone T. P., Duddridge R. J., Crompton M. The activation of Na+-dependent efflux of Ca2+ from liver mitochondria by glucagon and beta-adrenergic agonists. Biochem J. 1983 Feb 15;210(2):463–472. doi: 10.1042/bj2100463. [DOI] [PMC free article] [PubMed] [Google Scholar]
- Gupta R. K., Moore R. D. 31P NMR studies of intracellular free Mg2+ in intact frog skeletal muscle. J Biol Chem. 1980 May 10;255(9):3987–3993. [PubMed] [Google Scholar]
- Hansford R. G., Castro F. Effects of micromolar concentrations of free calcium ions on the reduction of heart mitochondrial NAD(P) by 2-oxoglutarate. Biochem J. 1981 Sep 15;198(3):525–533. doi: 10.1042/bj1980525. [DOI] [PMC free article] [PubMed] [Google Scholar]
- Hayat L. H., Crompton M. Ca2+-dependent inhibition by trifluoperazine of the Na+-Ca2+ carrier in mitoplasts derived from heart mitochondria. FEBS Lett. 1985 Mar 25;182(2):281–286. doi: 10.1016/0014-5793(85)80316-1. [DOI] [PubMed] [Google Scholar]
- Hayat L. H., Crompton M. Evidence for the existence of regulatory sites for Ca2+ on the Na+/Ca2+ carrier of cardiac mitochondria. Biochem J. 1982 Feb 15;202(2):509–518. doi: 10.1042/bj2020509. [DOI] [PMC free article] [PubMed] [Google Scholar]
- Hess P., Metzger P., Weingart R. Free magnesium in sheep, ferret and frog striated muscle at rest measured with ion-selective micro-electrodes. J Physiol. 1982 Dec;333:173–188. doi: 10.1113/jphysiol.1982.sp014447. [DOI] [PMC free article] [PubMed] [Google Scholar]
- Lukács G. L., Fonyó A. The Ba2+ sensitivity of the Na+-induced Ca2+ efflux in heart mitochondria: the site of inhibitory action. Biochim Biophys Acta. 1986 Jun 13;858(1):125–134. doi: 10.1016/0005-2736(86)90298-1. [DOI] [PubMed] [Google Scholar]
- McCormack J. G., England P. J. Ruthenium Red inhibits the activation of pyruvate dehydrogenase caused by positive inotropic agents in the perfused rat heart. Biochem J. 1983 Aug 15;214(2):581–585. doi: 10.1042/bj2140581. [DOI] [PMC free article] [PubMed] [Google Scholar]
- Palmer J. W., Pfeiffer D. R. The control of Ca2+ release from heart mitochondria. J Biol Chem. 1981 Jul 10;256(13):6742–6750. [PubMed] [Google Scholar]