Abstract
1. Two processes of Ruthenium Red-insensitive Ca2+ efflux exist in liver and in heart mitochondria: one Na+-independent, and another Na+-dependent. The processes attain maximal rates of 1.4 and 3.0 nmol of Ca2+.min-1.mg-1 for the Na+-dependent and 1.2 and 2.0 nmol of Ca2+.min-1.mg-1 for the Na+-independent, in liver and heart mitochondria, respectively. 2. The Na+-dependent pathway is inhibited, both in heart and in liver mitochondria, by the Ca2+ antagonist diltiazem with a Ki of 4 microM. The Na+-independent pathway is inhibited by diltiazem with a Ki of 250 microM in liver mitochondria, while it behaves as almost insensitive to diltiazem in heart mitochondria. 3. Stretching of the mitochondrial inner membrane in hypo-osmotic media results in activation of the Na+-independent pathway both in liver and in heart mitochondria. 4. Both in heart and liver mitochondria the Na+-independent pathway is insensitive to variations of medium pH around physiological values, while the Na+-dependent pathway is markedly stimulated parallel with acidification of the medium. The pH-activated, Na+-dependent pathway maintains the diltiazem sensitivity. 5. In heart mitochondria, the Na+-dependent pathway is non-competitively inhibited by Mg2+ with a Ki of 0.27 mM, while the Na+-independent pathway is less affected; similarly, in liver mitochondria Mg2+ inhibits the Na+-dependent pathway more than it does the Na+-independent pathway. In the presence of physiological concentrations of Na+, Ca2+ and Mg2+, the Na+-independent and the Na+-dependent pathways operate at rates, respectively, of 0.5 and 1.0 nmol of Ca2+.min-1.mg-1 in heart mitochondria and 0.9 and 0.2 nmol of Ca2+.min-1.mg-1 in liver mitochondria. It is concluded that both heart and liver mitochondria possess two independent pathways for Ca2+ efflux operating at comparable rates.
Full text
PDF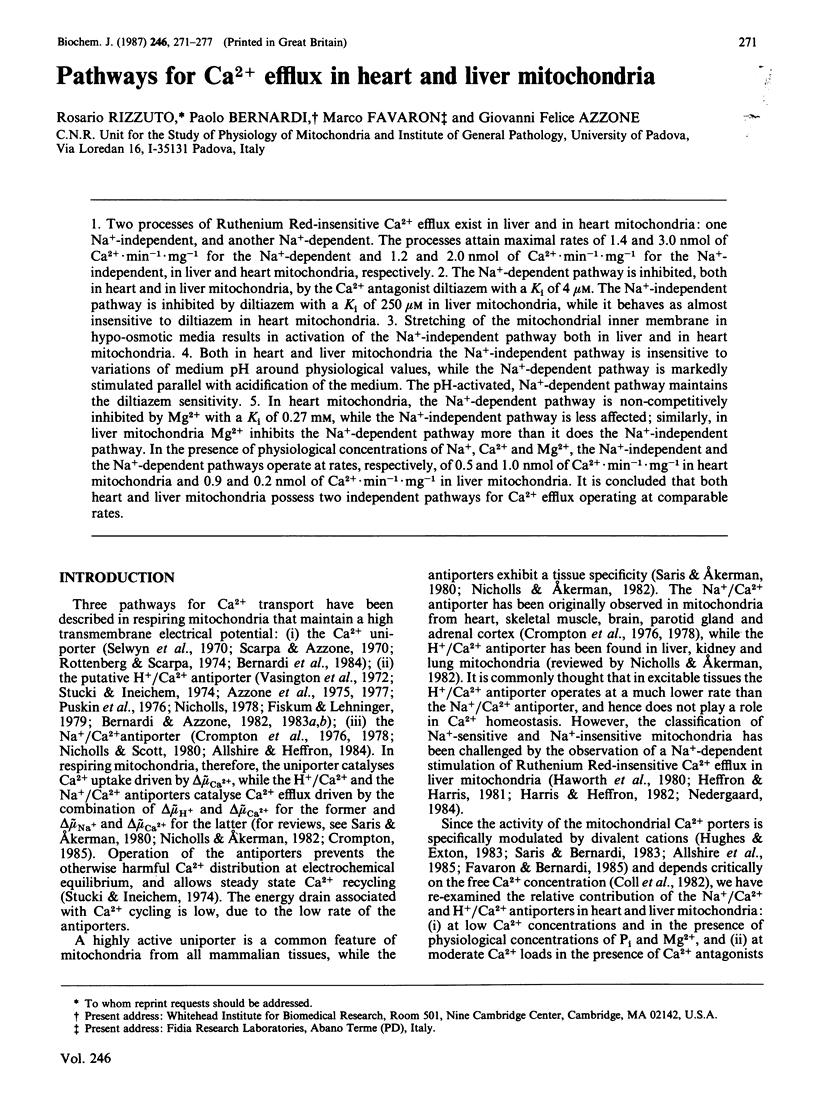
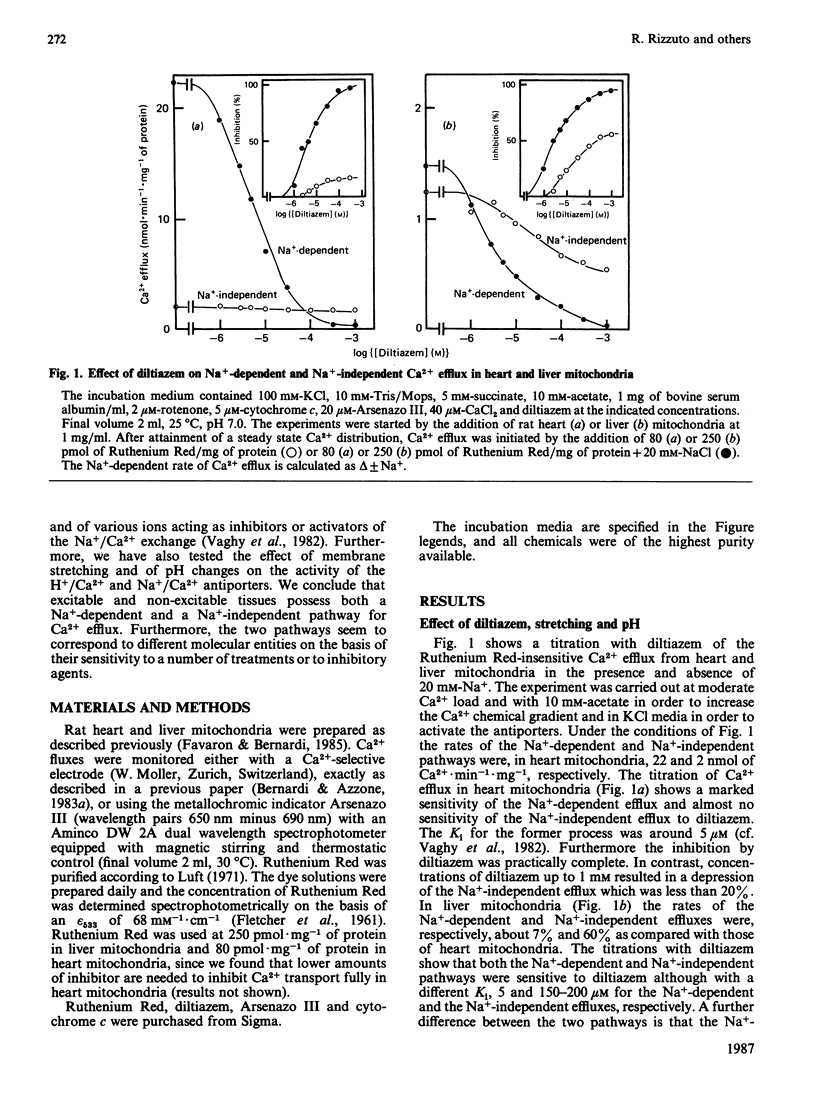
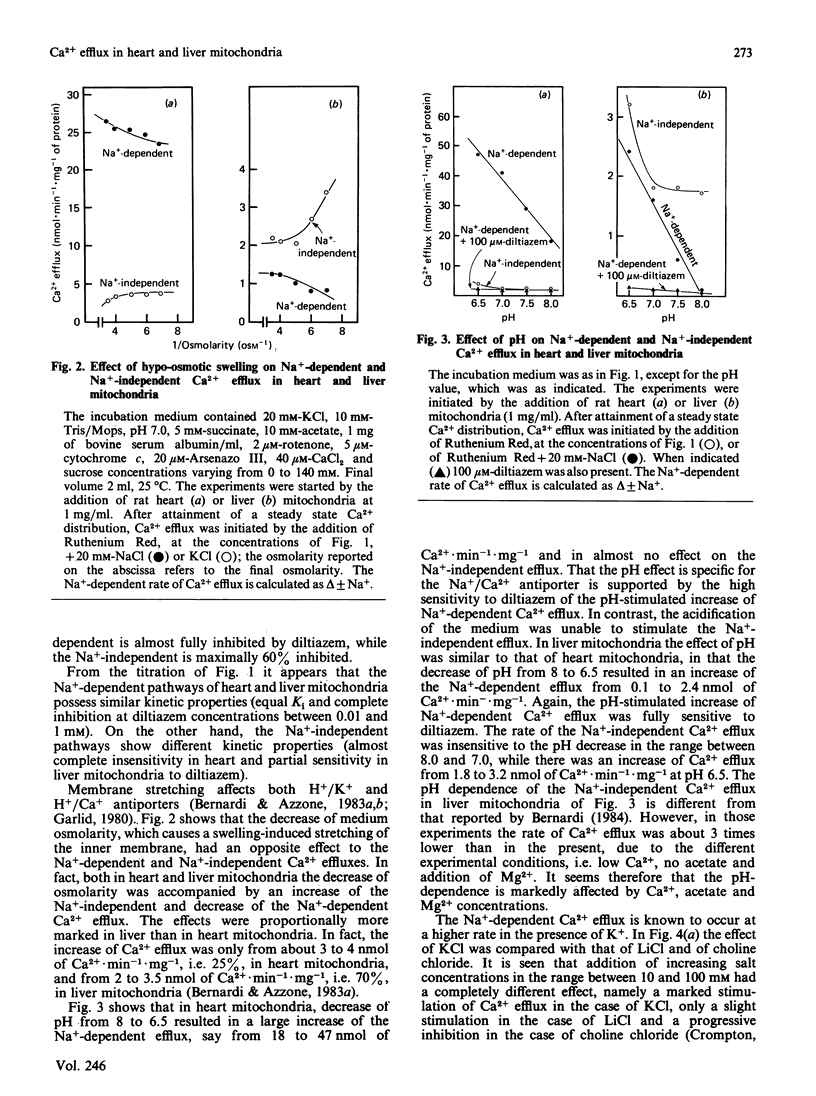
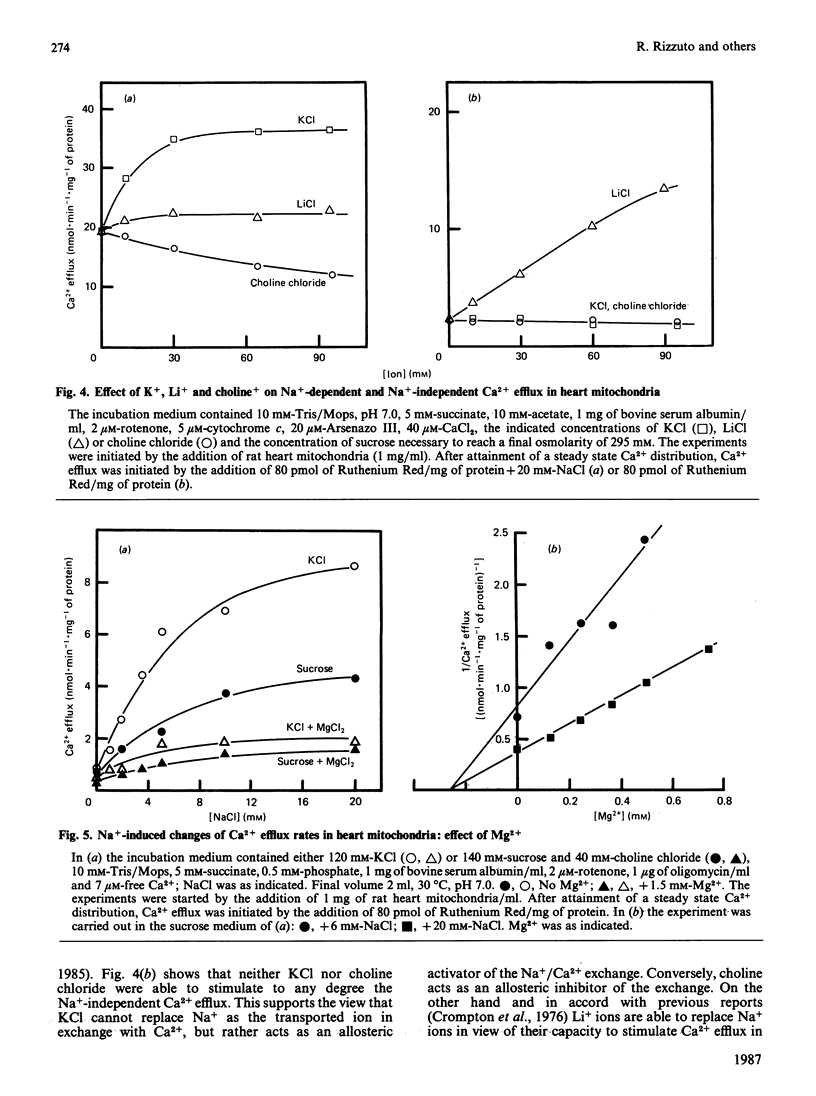
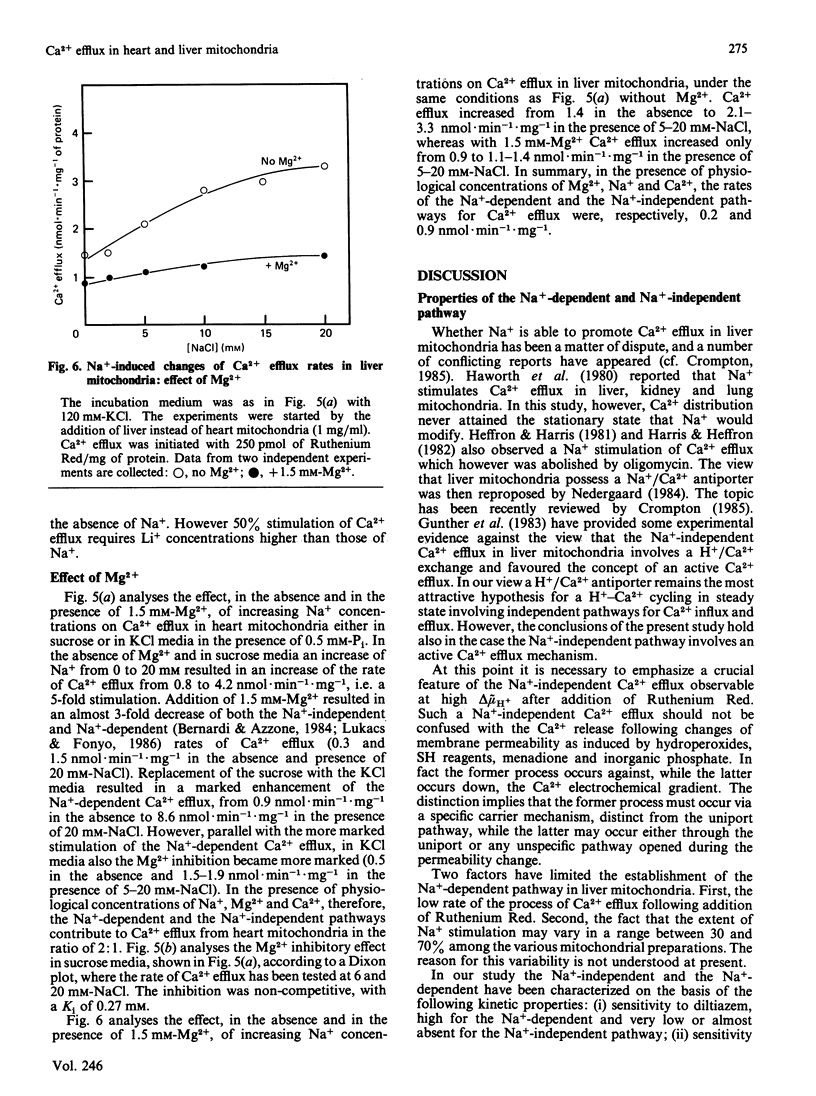
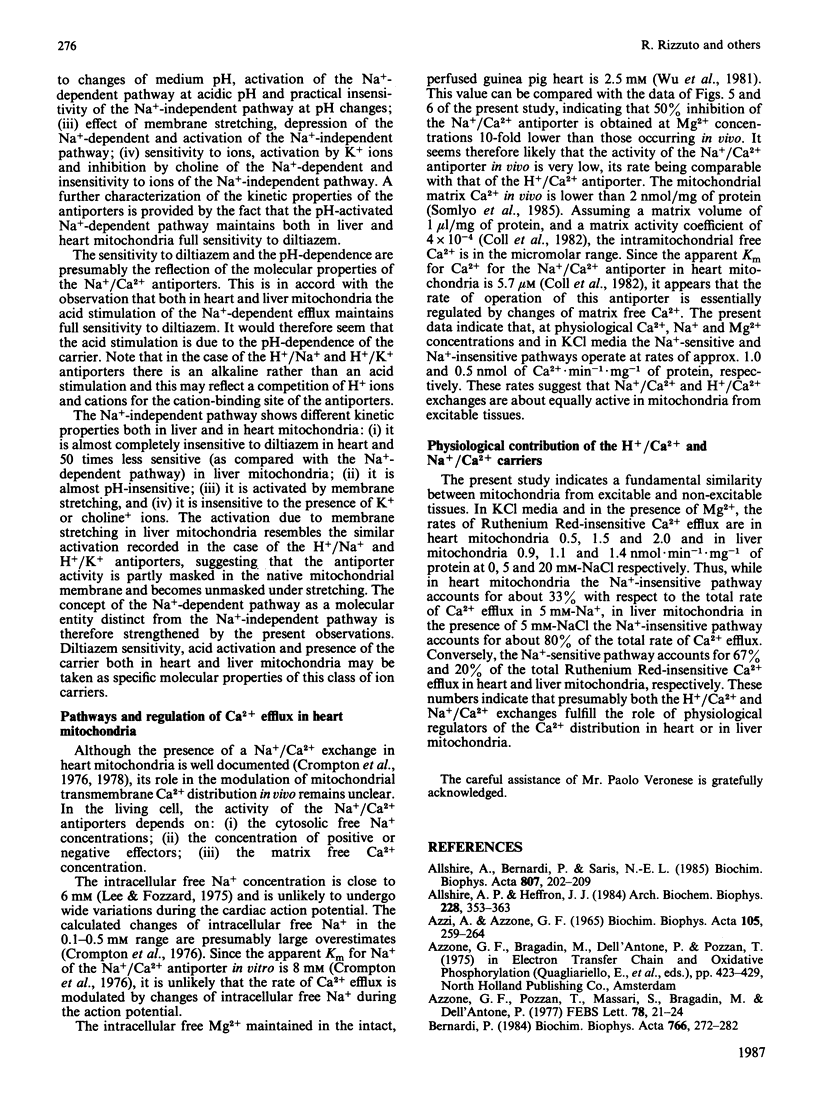
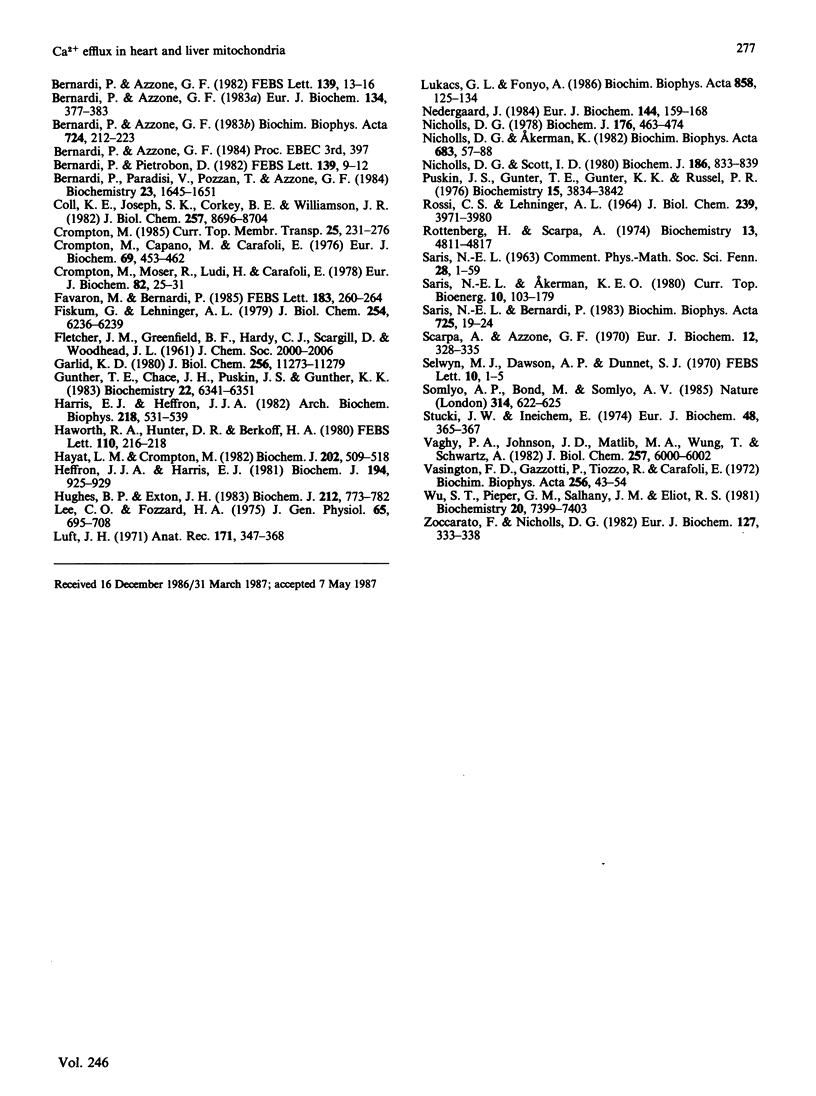
Selected References
These references are in PubMed. This may not be the complete list of references from this article.
- Allshire A. P., Heffron J. J. Uptake, retention, and efflux of Ca2+ by mitochondrial preparations from skeletal muscle. Arch Biochem Biophys. 1984 Jan;228(1):353–363. doi: 10.1016/0003-9861(84)90076-6. [DOI] [PubMed] [Google Scholar]
- Allshire A., Bernardi P., Saris N. E. Manganese stimulates calcium flux through the mitochondrial uniporter. Biochim Biophys Acta. 1985 May 3;807(2):202–209. doi: 10.1016/0005-2728(85)90123-9. [DOI] [PubMed] [Google Scholar]
- Azzi A., Azzone G. F. Swelling and shrinkage phenomena in liver mitochondria. I. Large amplitude swelling induced by inorganic phosphate and by ATP. Biochim Biophys Acta. 1965 Aug 24;105(2):253–264. doi: 10.1016/s0926-6593(65)80150-3. [DOI] [PubMed] [Google Scholar]
- Azzone G. F., Pozzan T., Massari S., Bragadin M., Dell'Antone P. H+/site ratio and steady state distribution of divalent cations in mitochondria. FEBS Lett. 1977;78(1):21–24. doi: 10.1016/0014-5793(77)80264-0. [DOI] [PubMed] [Google Scholar]
- Bernardi P., Azzone G. F. A membrane potential-modulated pathway for Ca2+ efflux in rat liver mitochondria. FEBS Lett. 1982 Mar 8;139(1):13–16. doi: 10.1016/0014-5793(82)80476-6. [DOI] [PubMed] [Google Scholar]
- Bernardi P., Azzone G. F. Electroneutral H+-K+ exchange in liver mitochondria. Regulation by membrane potential. Biochim Biophys Acta. 1983 Aug 31;724(2):212–223. doi: 10.1016/0005-2728(83)90140-8. [DOI] [PubMed] [Google Scholar]
- Bernardi P., Azzone G. F. Regulation of Ca2+ efflux in rat liver mitochondria. Role of membrane potential. Eur J Biochem. 1983 Aug 1;134(2):377–383. doi: 10.1111/j.1432-1033.1983.tb07578.x. [DOI] [PubMed] [Google Scholar]
- Bernardi P. Modulation of Ca2+ efflux and rebounding Ca2+ transport in rat liver mitochondria. Biochim Biophys Acta. 1984 Aug 31;766(2):277–282. doi: 10.1016/0005-2728(84)90242-1. [DOI] [PubMed] [Google Scholar]
- Bernardi P., Paradisi V., Pozzan T., Azzone G. F. Pathway for uncoupler-induced calcium efflux in rat liver mitochondria: inhibition by ruthenium red. Biochemistry. 1984 Apr 10;23(8):1645–1651. doi: 10.1021/bi00303a010. [DOI] [PubMed] [Google Scholar]
- Bernardi P., Pietrobon D. On the nature of Pi-induced, Mg2+-prevented Ca2+ release in rat liver mitochondria. FEBS Lett. 1982 Mar 8;139(1):9–12. doi: 10.1016/0014-5793(82)80475-4. [DOI] [PubMed] [Google Scholar]
- Coll K. E., Joseph S. K., Corkey B. E., Williamson J. R. Determination of the matrix free Ca2+ concentration and kinetics of Ca2+ efflux in liver and heart mitochondria. J Biol Chem. 1982 Aug 10;257(15):8696–8704. [PubMed] [Google Scholar]
- Crompton M., Moser R., Lüdi H., Carafoli E. The interrelations between the transport of sodium and calcium in mitochondria of various mammalian tissues. Eur J Biochem. 1978 Jan 2;82(1):25–31. doi: 10.1111/j.1432-1033.1978.tb11993.x. [DOI] [PubMed] [Google Scholar]
- Favaron M., Bernardi P. Tissue-specific modulation of the mitochondrial calcium uniporter by magnesium ions. FEBS Lett. 1985 Apr 22;183(2):260–264. doi: 10.1016/0014-5793(85)80789-4. [DOI] [PubMed] [Google Scholar]
- Fiskum G., Lehninger A. L. Regulated release of Ca2+ from respiring mitochondria by Ca2+/2H+ antiport. J Biol Chem. 1979 Jul 25;254(14):6236–6239. [PubMed] [Google Scholar]
- Garlid K. D. On the mechanism of regulation of the mitochondrial K+/H+ exchanger. J Biol Chem. 1980 Dec 10;255(23):11273–11279. [PubMed] [Google Scholar]
- Gunter T. E., Chace J. H., Puskin J. S., Gunter K. K. Mechanism of sodium independent calcium efflux from rat liver mitochondria. Biochemistry. 1983 Dec 20;22(26):6341–6351. doi: 10.1021/bi00295a046. [DOI] [PubMed] [Google Scholar]
- Harris E. J., Heffron J. J. The stimulation of the release of Ca2+ from mitochondria by sodium ions and its inhibition. Arch Biochem Biophys. 1982 Oct 15;218(2):531–539. doi: 10.1016/0003-9861(82)90377-0. [DOI] [PubMed] [Google Scholar]
- Haworth R. A., Hunter D. R., Berkoff H. A. Na+ releases Ca2+ from liver, kidney and lung mitochondria. FEBS Lett. 1980 Feb 11;110(2):216–218. doi: 10.1016/0014-5793(80)80076-7. [DOI] [PubMed] [Google Scholar]
- Hayat L. H., Crompton M. Evidence for the existence of regulatory sites for Ca2+ on the Na+/Ca2+ carrier of cardiac mitochondria. Biochem J. 1982 Feb 15;202(2):509–518. doi: 10.1042/bj2020509. [DOI] [PMC free article] [PubMed] [Google Scholar]
- Heffron J. J., Harris E. J. Stimulation of calcium-ion efflux from liver mitochondria by sodium ions and its response to ADP and energy state. Biochem J. 1981 Mar 15;194(3):925–929. doi: 10.1042/bj1940925. [DOI] [PMC free article] [PubMed] [Google Scholar]
- Hughes B. P., Exton J. H. Effect of micromolar concentrations of manganese ions on calcium-ion cycling in rat liver mitochondria. Biochem J. 1983 Jun 15;212(3):773–782. doi: 10.1042/bj2120773. [DOI] [PMC free article] [PubMed] [Google Scholar]
- Lee C. O., Fozzard H. A. Activities of potassium and sodium ions in rabbit heart muscle. J Gen Physiol. 1975 Jun;65(6):695–708. doi: 10.1085/jgp.65.6.695. [DOI] [PMC free article] [PubMed] [Google Scholar]
- Luft J. H. Ruthenium red and violet. I. Chemistry, purification, methods of use for electron microscopy and mechanism of action. Anat Rec. 1971 Nov;171(3):347–368. doi: 10.1002/ar.1091710302. [DOI] [PubMed] [Google Scholar]
- Lukács G. L., Fonyó A. The Ba2+ sensitivity of the Na+-induced Ca2+ efflux in heart mitochondria: the site of inhibitory action. Biochim Biophys Acta. 1986 Jun 13;858(1):125–134. doi: 10.1016/0005-2736(86)90298-1. [DOI] [PubMed] [Google Scholar]
- Nedergaard J. Na+-dependent regulation of extramitochondrial Ca2+ by rat-liver mitochondria. Eur J Biochem. 1984 Oct 1;144(1):159–168. doi: 10.1111/j.1432-1033.1984.tb08444.x. [DOI] [PubMed] [Google Scholar]
- Nicholls D. G., Scott I. D. The regulation of brain mitochondrial calcium-ion transport. The role of ATP in the discrimination between kinetic and membrane-potential-dependent calcium-ion efflux mechanisms. Biochem J. 1980 Mar 15;186(3):833–839. doi: 10.1042/bj1860833. [DOI] [PMC free article] [PubMed] [Google Scholar]
- Nicholls D. G. The regulation of extramitochondrial free calcium ion concentration by rat liver mitochondria. Biochem J. 1978 Nov 15;176(2):463–474. doi: 10.1042/bj1760463. [DOI] [PMC free article] [PubMed] [Google Scholar]
- Nicholls D., Akerman K. Mitochondrial calcium transport. Biochim Biophys Acta. 1982 Sep 1;683(1):57–88. doi: 10.1016/0304-4173(82)90013-1. [DOI] [PubMed] [Google Scholar]
- Puskin J. S., Gunter T. E., Gunter K. K., Russell P. R. Evidence for more than one Ca2+ transport mechanism in mitochondria. Biochemistry. 1976 Aug 24;15(17):3834–3842. doi: 10.1021/bi00662a029. [DOI] [PubMed] [Google Scholar]
- ROSSI C. S., LEHNINGER A. L. STOICHIOMETRY OF RESPIRATORY STIMULATION, ACCUMULATION OF CA++ AND PHOSPHATE, AND OXIDATIVE PHOSPHORYLATION IN RAT LIVER MITOCHONDRIA. J Biol Chem. 1964 Nov;239:3971–3980. [PubMed] [Google Scholar]
- Rottenberg H., Scarpa A. Calcium uptake and membrane potential in mitochondria. Biochemistry. 1974 Nov 5;13(23):4811–4817. doi: 10.1021/bi00720a020. [DOI] [PubMed] [Google Scholar]
- Saris N. E., Bernardi P. Inhibition by Sr2+ of specific mitochondrial Ca2+-efflux pathways. Biochim Biophys Acta. 1983 Oct 31;725(1):19–24. doi: 10.1016/0005-2728(83)90219-0. [DOI] [PubMed] [Google Scholar]
- Scarpa A., Azzone G. F. The mechanism of ion translocation in mitochondria. 4. Coupling of K+ efflux with Ca2+ uptake. Eur J Biochem. 1970 Feb;12(2):328–335. doi: 10.1111/j.1432-1033.1970.tb00854.x. [DOI] [PubMed] [Google Scholar]
- Selwyn M. J., Dawson A. P., Dunnett S. J. Calcium transport in mitochondria. FEBS Lett. 1970 Sep 18;10(1):1–5. doi: 10.1016/0014-5793(70)80402-1. [DOI] [PubMed] [Google Scholar]
- Somlyo A. P., Bond M., Somlyo A. V. Calcium content of mitochondria and endoplasmic reticulum in liver frozen rapidly in vivo. Nature. 1985 Apr 18;314(6012):622–625. doi: 10.1038/314622a0. [DOI] [PubMed] [Google Scholar]
- Stucki J. W., Ineichen E. A. Energy dissipation by calcium recycling and the efficiency of calcium transport in rat-liver mitochondria. Eur J Biochem. 1974 Oct 2;48(2):365–375. doi: 10.1111/j.1432-1033.1974.tb03778.x. [DOI] [PubMed] [Google Scholar]
- Vasington F. D., Gazzotti P., Tiozzo R., Carafoli E. The effect of ruthenium red on Ca 2+ transport and respiration in rat liver mitochondria. Biochim Biophys Acta. 1972 Jan 21;256(1):43–54. doi: 10.1016/0005-2728(72)90161-2. [DOI] [PubMed] [Google Scholar]
- Vághy P. L., Johnson J. D., Matlib M. A., Wang T., Schwartz A. Selective inhibition of Na+-induced Ca2+ release from heart mitochondria by diltiazem and certain other Ca2+ antagonist drugs. J Biol Chem. 1982 Jun 10;257(11):6000–6002. [PubMed] [Google Scholar]
- Wu S. T., Pieper G. M., Salhany J. M., Eliot R. S. Measurement of free magnesium in perfused and ischemic arrested heart muscle. A quantitative phosphorus-31 nuclear magnetic resonance and multiequilibria analysis. Biochemistry. 1981 Dec 22;20(26):7399–7403. doi: 10.1021/bi00529a012. [DOI] [PubMed] [Google Scholar]
- Zoccarato F., Nicholls D. The role of phosphate in the regulation of the independent calcium-efflux pathway of liver mitochondria. Eur J Biochem. 1982 Oct;127(2):333–338. doi: 10.1111/j.1432-1033.1982.tb06875.x. [DOI] [PubMed] [Google Scholar]