Abstract
The effects of rotenone on the succinate-driven reduction of matrix nicotinamide nucleotides were investigated in Percoll-purified mitochondria from potato (Solanum tuberosum) tubers. Depending on the presence of ADP or ATP, rotenone caused an increase or a decrease in the level of reduction of the matrix nicotinamide nucleotides. The increase in the reduction induced by rotenone in the presence of ADP was linked to the oxidation of the malate resulting from the oxidation of succinate. Depending on the experimental conditions, malic enzyme (at pH 6.6 or in the presence of added CoA) or malate dehydrogenase (at pH 7.9) were involved in this oxidation. At pH 7.9, the oxaloacetate produced progressively inhibited the succinate dehydrogenase. In the presence of ATP the production of oxaloacetate was stopped, and succinate dehydrogenase was protected from inhibition by oxaloacetate. However, previously accumulated oxaloacetate transitorily decreased the level of the reduction of the NAD+ driven by succinate, by causing the reversal of the malate dehydrogenase reaction. Under these conditions (i.e. presence of ATP), rotenone strongly inhibited the reduction of NAD+ by succinate-driven reverse electron flow. No evidence for an active reverse electron transport through a rotenone-insensitive path could be obtained. The inhibitory effect of rotenone was masked if malate had previously accumulated, owing to the malate-oxidizing enzymes which reduced part or all of the matrix NAD+.
Full text
PDF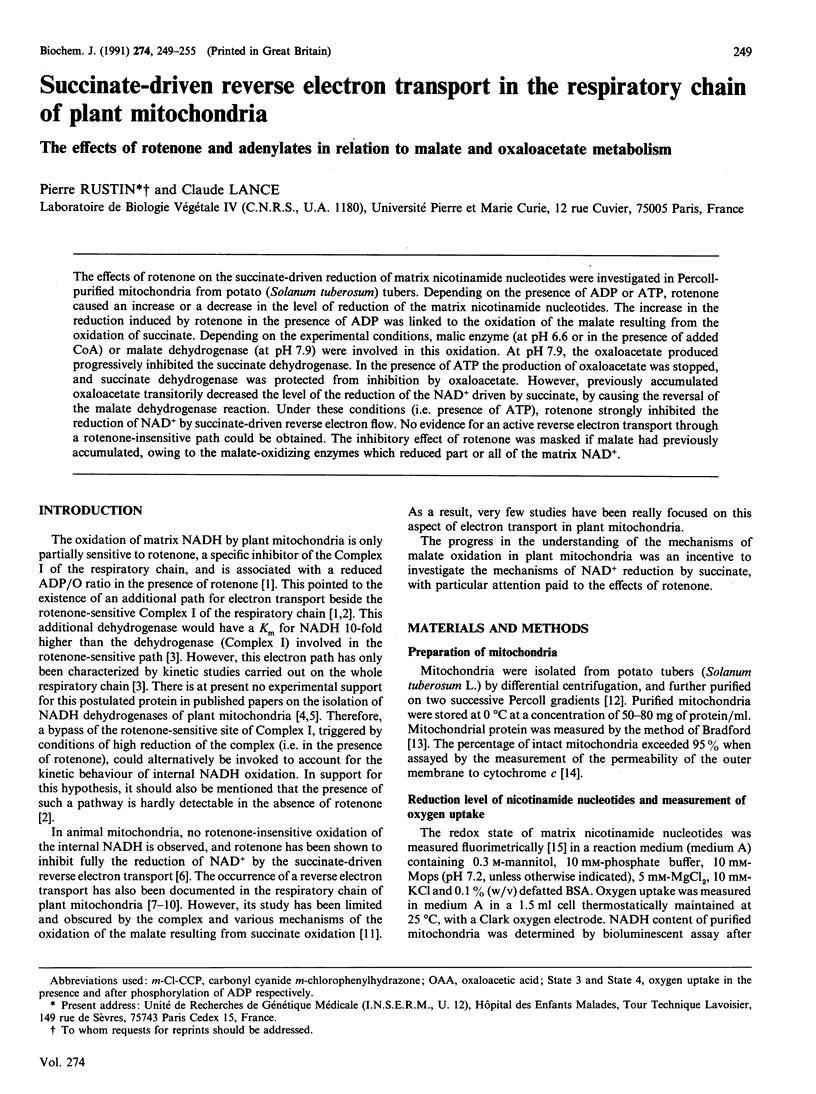
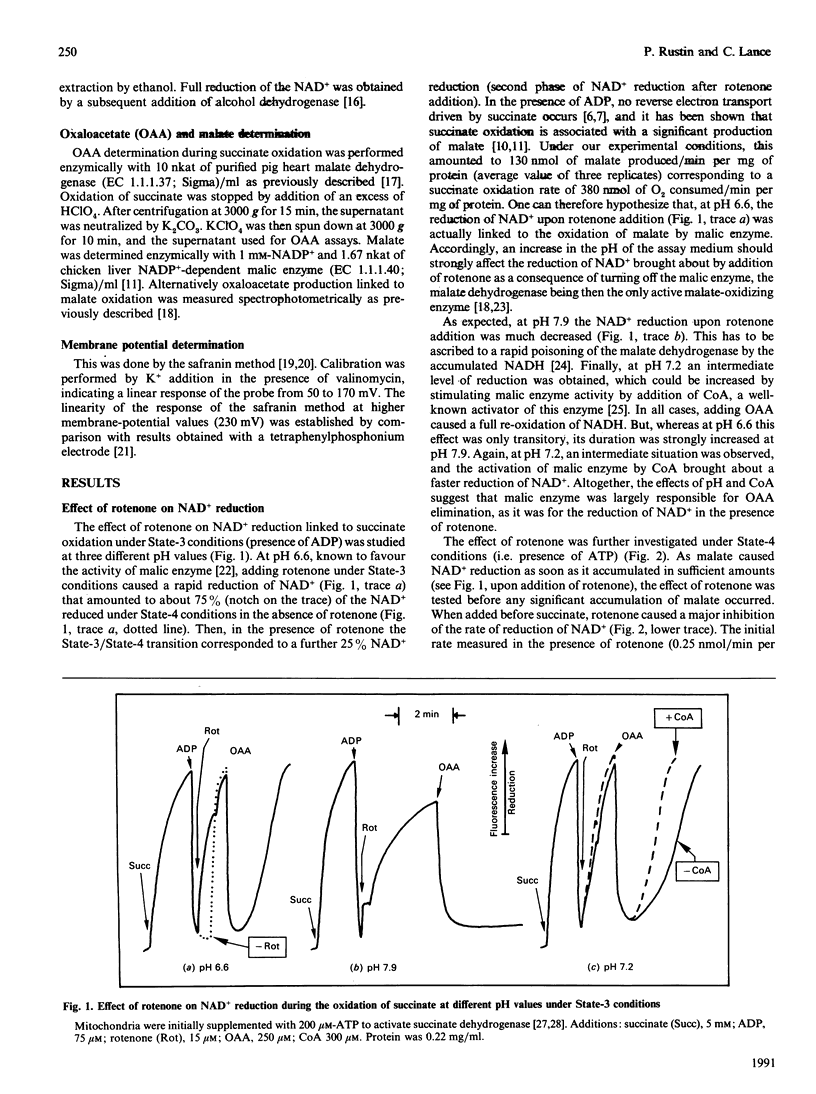
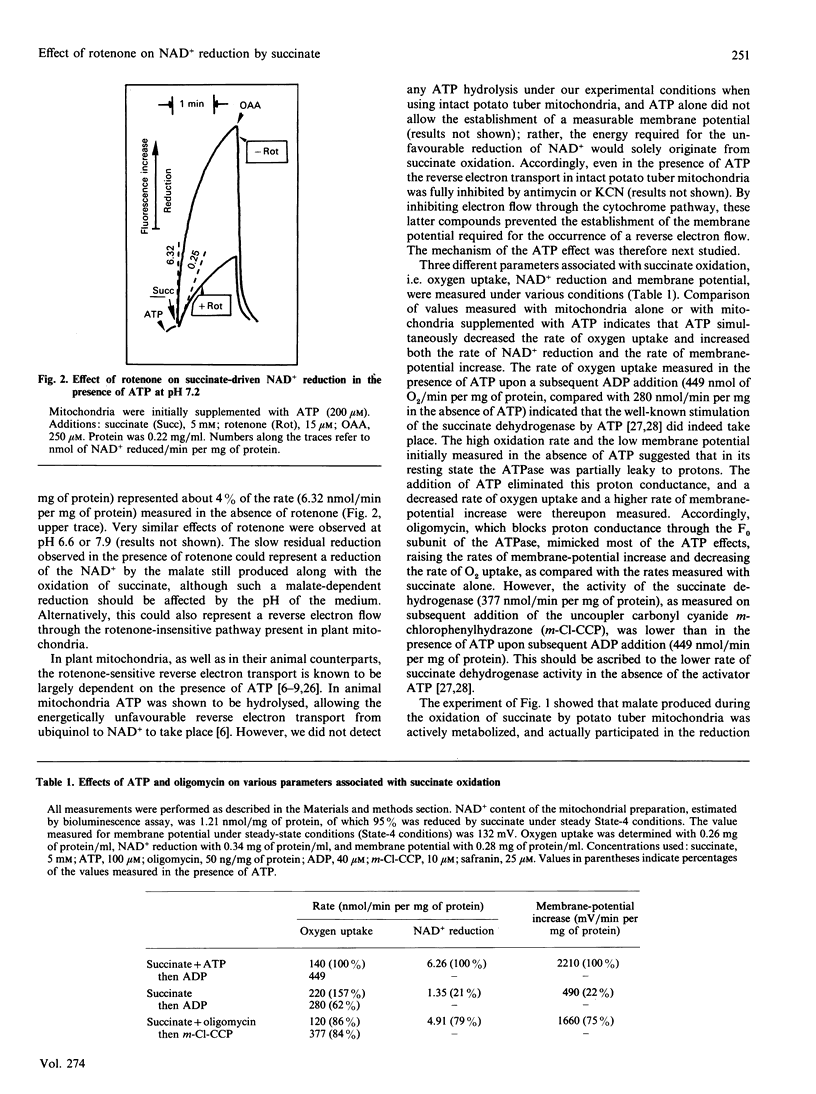
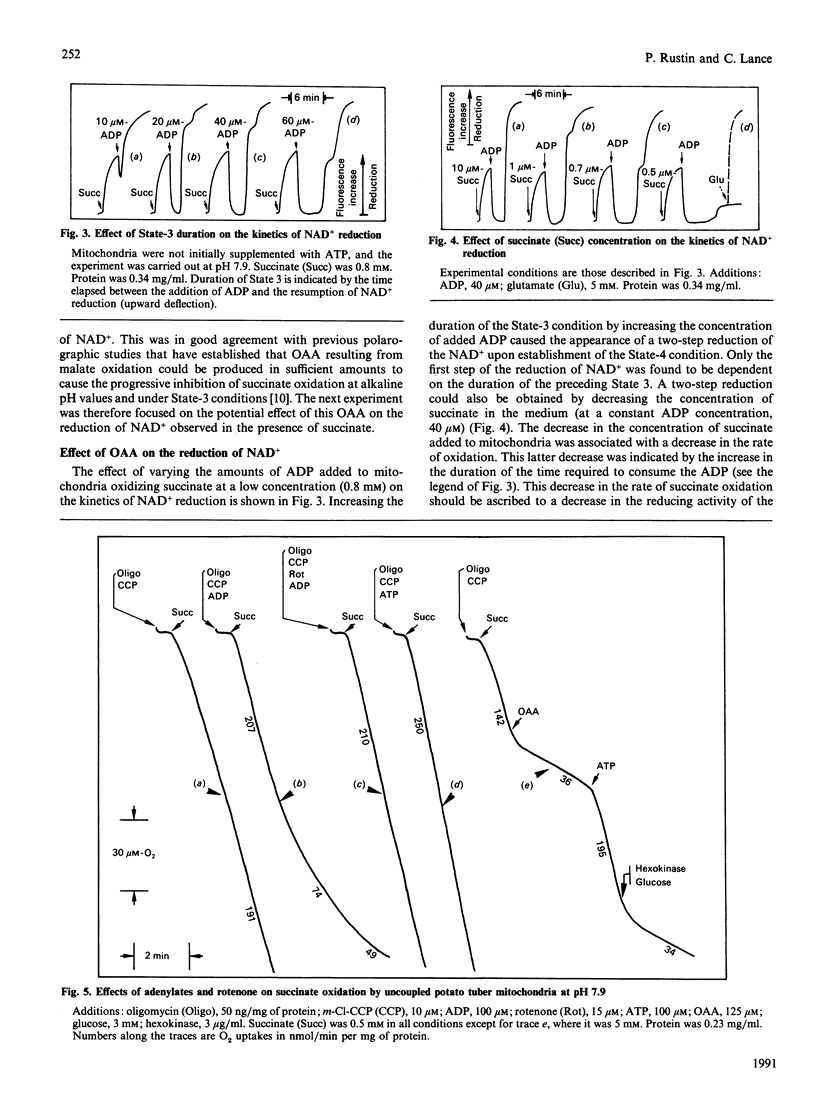
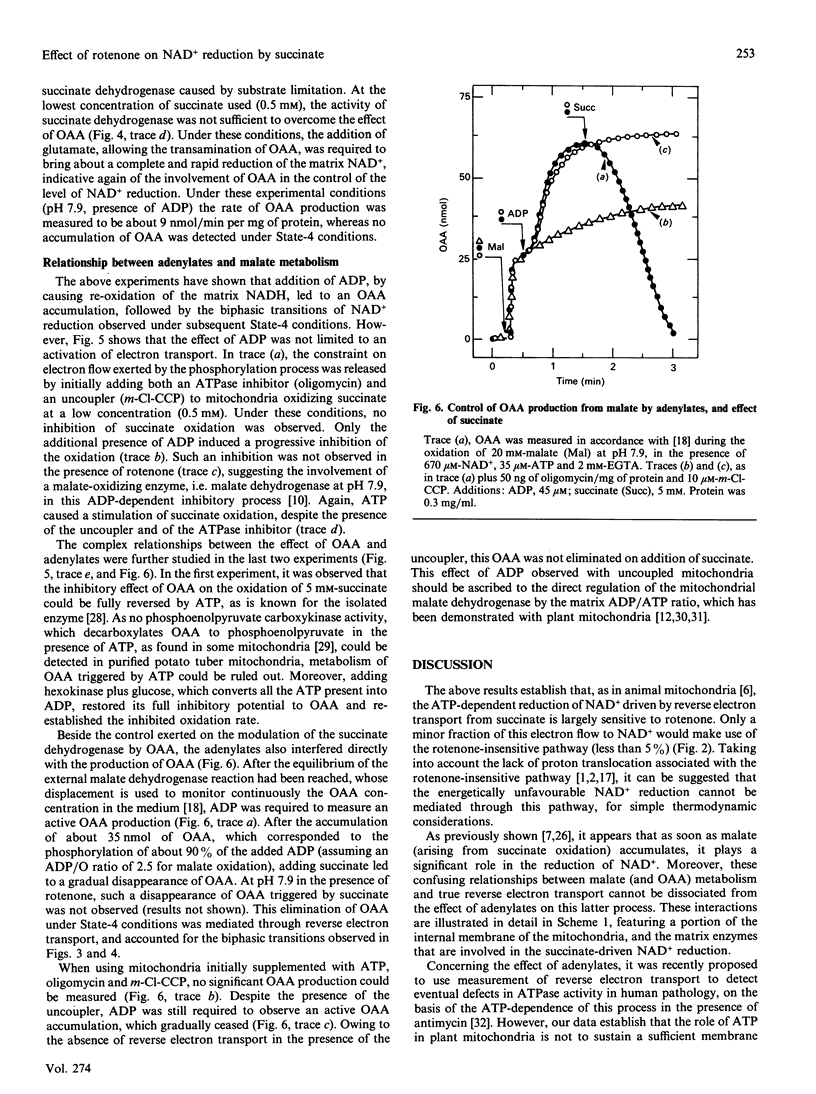
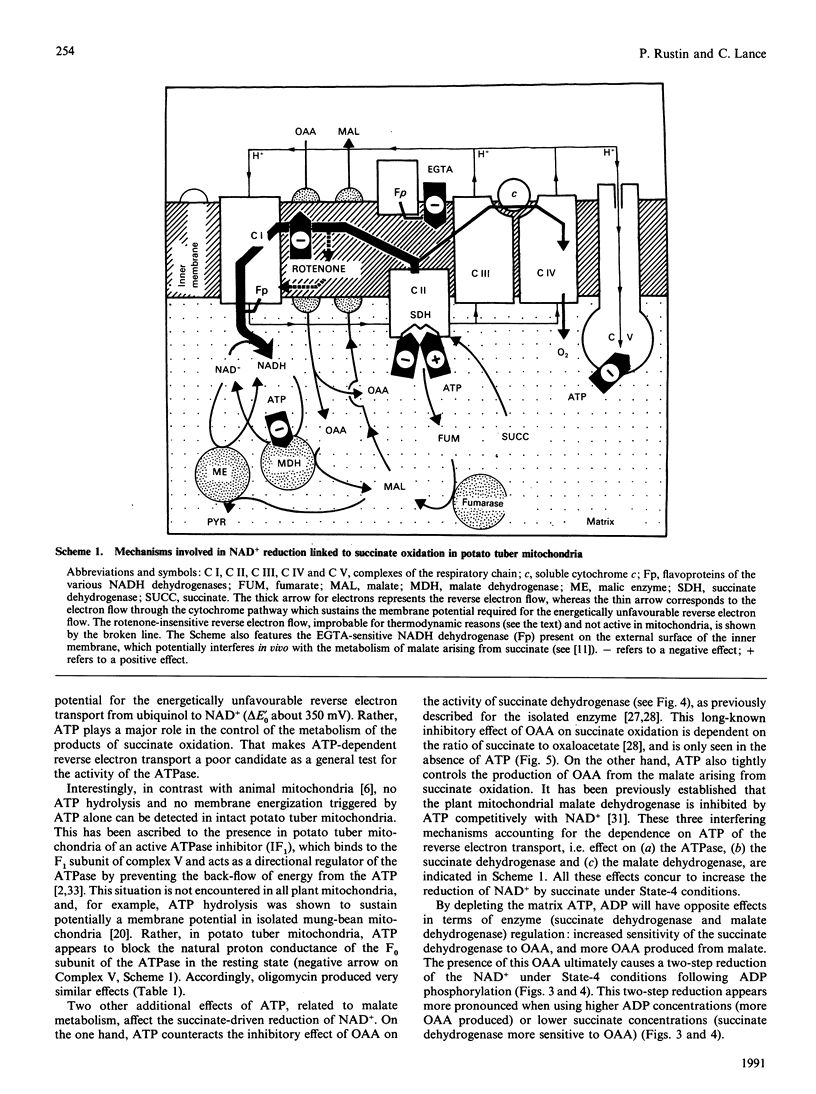
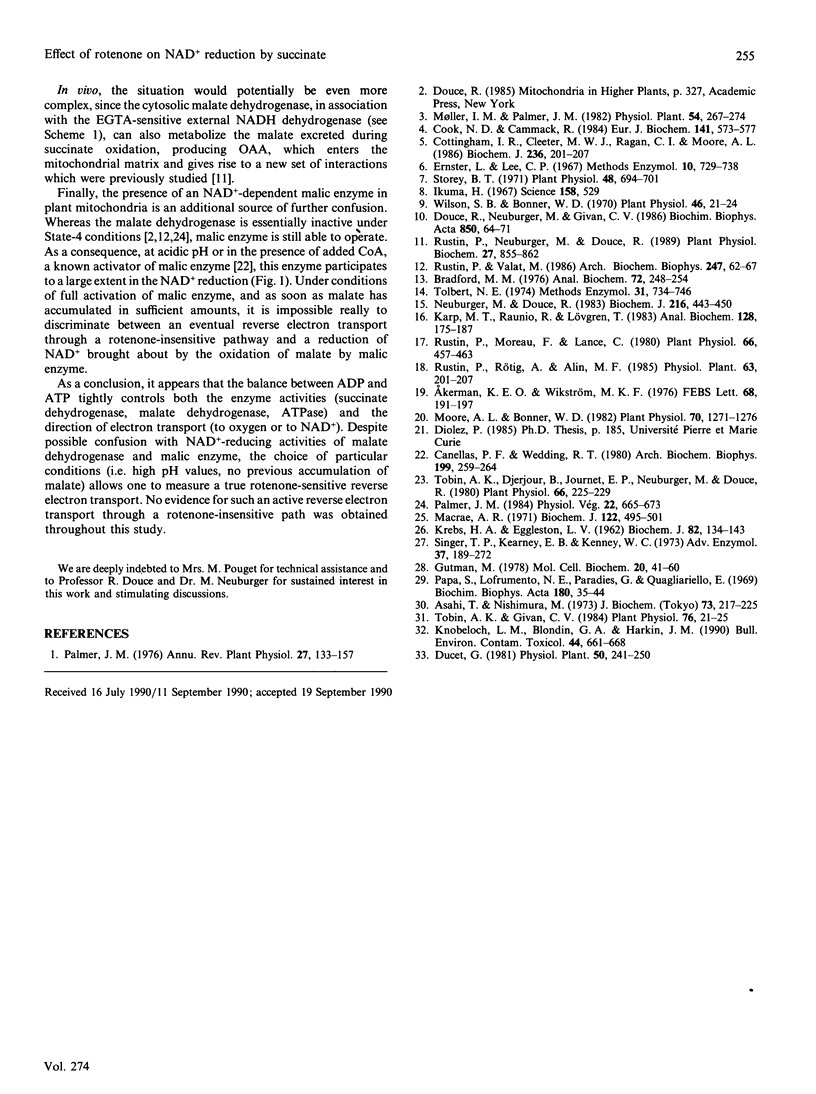
Selected References
These references are in PubMed. This may not be the complete list of references from this article.
- Akerman K. E., Wikström M. K. Safranine as a probe of the mitochondrial membrane potential. FEBS Lett. 1976 Oct 1;68(2):191–197. doi: 10.1016/0014-5793(76)80434-6. [DOI] [PubMed] [Google Scholar]
- Asahi T., Nishimura M. Regulatory function of malate dehydrogenase isoenzymes in the cotyledons of mung bean. J Biochem. 1973 Feb;73(2):217–225. [PubMed] [Google Scholar]
- Bradford M. M. A rapid and sensitive method for the quantitation of microgram quantities of protein utilizing the principle of protein-dye binding. Anal Biochem. 1976 May 7;72:248–254. doi: 10.1006/abio.1976.9999. [DOI] [PubMed] [Google Scholar]
- Canellas P. F., Wedding R. T. Substrate and metal ion interactions in the NAD+ malic enzyme from cauliflower. Arch Biochem Biophys. 1980 Jan;199(1):259–264. doi: 10.1016/0003-9861(80)90279-9. [DOI] [PubMed] [Google Scholar]
- Cook N. D., Cammack R. Purification and characterization of the rotenone-insensitive NADH dehydrogenase of mitochondria from Arum maculatum. Eur J Biochem. 1984 Jun 15;141(3):573–577. doi: 10.1111/j.1432-1033.1984.tb08231.x. [DOI] [PubMed] [Google Scholar]
- Cottingham I. R., Cleeter M. W., Ragan C. I., Moore A. L. Immunological analysis of plant mitochondrial NADH dehydrogenases. Biochem J. 1986 May 15;236(1):201–207. doi: 10.1042/bj2360201. [DOI] [PMC free article] [PubMed] [Google Scholar]
- Gutman M. Modulation of mitochondrial succinate dehydrogenase activity, mechanism and function. Mol Cell Biochem. 1978 Jun 15;20(1):41–60. doi: 10.1007/BF00229453. [DOI] [PubMed] [Google Scholar]
- Ikuma H. Pyridine nucleotide in mung bean mitochondria. Science. 1967 Oct 27;158(3800):529–529. doi: 10.1126/science.158.3800.529-b. [DOI] [PubMed] [Google Scholar]
- KREBS H. A., EGGLESTON L. V. The effect of dinitrophenol and amytal on the reduction of acetoacetate in the presence of succinate. Biochem J. 1962 Jan;82:134–143. doi: 10.1042/bj0820134. [DOI] [PMC free article] [PubMed] [Google Scholar]
- Karp M. T., Raunio R. P., Lövgren T. N. Simultaneous extraction and combined bioluminescent assay of NAD+ and NADH. Anal Biochem. 1983 Jan;128(1):175–180. doi: 10.1016/0003-2697(83)90359-7. [DOI] [PubMed] [Google Scholar]
- Knobeloch L. M., Blondin G. A., Harkin J. M. Use of submitochondrial particles for prediction of chemical toxicity in man. Bull Environ Contam Toxicol. 1990 May;44(5):661–668. doi: 10.1007/BF01701785. [DOI] [PubMed] [Google Scholar]
- Macrae A. R. Isolation and properties of a 'malic' enzyme from cauliflower bud mitochondria. Biochem J. 1971 May;122(4):495–501. doi: 10.1042/bj1220495. [DOI] [PMC free article] [PubMed] [Google Scholar]
- Moore A. L., Bonner W. D. Measurements of membrane potentials in plant mitochondria with the safranine method. Plant Physiol. 1982 Nov;70(5):1271–1276. doi: 10.1104/pp.70.5.1271. [DOI] [PMC free article] [PubMed] [Google Scholar]
- Neuburger M., Douce R. Slow passive diffusion of NAD+ between intact isolated plant mitochondria and suspending medium. Biochem J. 1983 Nov 15;216(2):443–450. doi: 10.1042/bj2160443. [DOI] [PMC free article] [PubMed] [Google Scholar]
- Papa S., Lofrumento N. E., Paradies G., Quagliariello E. Mechanism of inhibition by uncouples of succinate oxidation in isolated mitochondria. Biochim Biophys Acta. 1969 May;180(1):35–44. doi: 10.1016/0005-2728(69)90191-1. [DOI] [PubMed] [Google Scholar]
- Rustin P., Moreau F., Lance C. Malate Oxidation in Plant Mitochondria via Malic Enzyme and the Cyanide-insensitive Electron Transport Pathway. Plant Physiol. 1980 Sep;66(3):457–462. doi: 10.1104/pp.66.3.457. [DOI] [PMC free article] [PubMed] [Google Scholar]
- Rustin P., Valat M. The control of malate dehydrogenase activity by adenine nucleotides in purified potato tuber (Solanum tuberosum L.) mitochondria. Arch Biochem Biophys. 1986 May 15;247(1):62–67. doi: 10.1016/0003-9861(86)90533-3. [DOI] [PubMed] [Google Scholar]
- Singer T. P., Kearney E. B., Kenney W. C. Succinate dehydrogenase. Adv Enzymol Relat Areas Mol Biol. 1973;37:189–272. doi: 10.1002/9780470122822.ch4. [DOI] [PubMed] [Google Scholar]
- Storey B. T. The Respiratory Chain of Plant Mitochondria: XI. Electron Transport from Succinate to Endogenous Pyridine Nucleotide in Mung Bean Mitochondria. Plant Physiol. 1971 Dec;48(6):694–701. doi: 10.1104/pp.48.6.694. [DOI] [PMC free article] [PubMed] [Google Scholar]
- Tobin A. K., Givan C. V. Adenine nucleotide regulation of malate oxidation in isolated mung bean hypocotyl mitochondria. Plant Physiol. 1984 Sep;76(1):21–25. doi: 10.1104/pp.76.1.21. [DOI] [PMC free article] [PubMed] [Google Scholar]
- Tobin A., Djerdjour B., Journet E., Neuburger M., Douce R. Effect of NAD on Malate Oxidation in Intact Plant Mitochondria. Plant Physiol. 1980 Aug;66(2):225–229. doi: 10.1104/pp.66.2.225. [DOI] [PMC free article] [PubMed] [Google Scholar]
- Tolbert N. E. Isolation of subcellular organelles of metabolism on isopycnic sucrose gradients. Methods Enzymol. 1974;31:734–746. doi: 10.1016/0076-6879(74)31077-4. [DOI] [PubMed] [Google Scholar]
- Wilson S. B., Bonner W. D. Effects of guanidine inhibitors on mung bean mitochondria. Plant Physiol. 1970 Jul;46(1):21–24. doi: 10.1104/pp.46.1.21. [DOI] [PMC free article] [PubMed] [Google Scholar]