Abstract
Thermal inactivation of glyceraldehyde-3-phosphate dehydrogenase appeared to be caused by a conformational mechanism, without involvement of covalent reactions. On the other hand, photodynamic inactivation of the enzyme (induced by illumination in the presence of Photofrin II) was caused by photo-oxidation of the essential thiol group in the active centre. A short photodynamic treatment of the enzyme, leading to only a limited inactivation, caused a pronounced potentiation of subsequent thermal inactivation, as measured over the temperature range 40-50 degrees C. Analysis of the experimental results according to the Arrhenius equation revealed that both the activation energy of thermal inactivation and the frequency factor (the proportionality constant) were significantly decreased by the preceding photodynamic treatment. The experimental results indicate a mechanism in which limited photodynamic treatment induced a conformational change of the protein molecule. This conformational change did not contribute to photodynamic enzyme inhibition, but was responsible for the decreased frequency factor and activation energy of subsequent thermal inactivation of the enzyme. The opposing effects of decreased activation energy and decreased frequency factor resulted in potentiation of thermal inactivation of the enzyme over the temperature range 40-50 degrees C. With other proteins, different results were obtained. With amylase the combined photodynamic and thermal effects were not synergistic, but additive, and photodynamic treatment had no effect on the frequency factor and the activation energy of thermal inactivation. With respect to myoglobin denaturation, the photodynamic and thermal effects were antagonistic over the whole practically applicable temperature range. Limited photodynamic treatment protected the protein against heat-induced precipitation, concomitantly increasing both the frequency factor and the activation energy of the process. These results offer a model for one of the possible mechanisms of synergistic interaction between photodynamic therapy and hyperthermia in cancer treatment.
Full text
PDF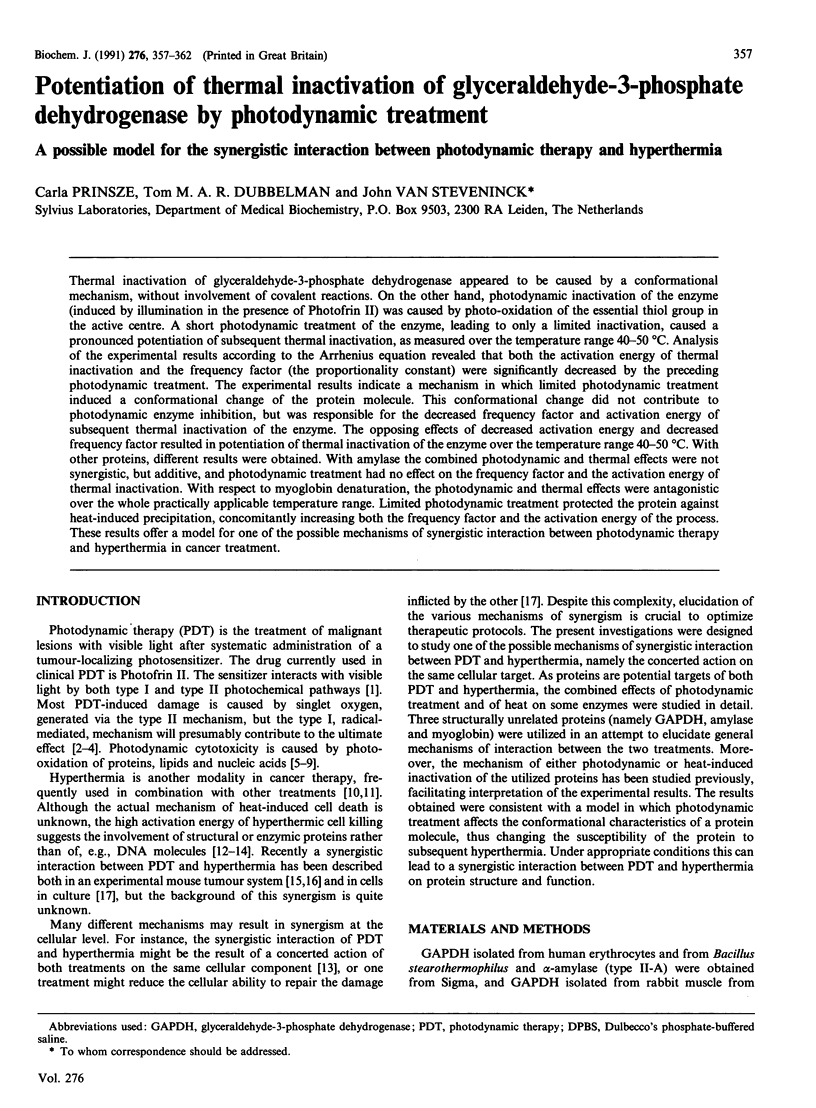
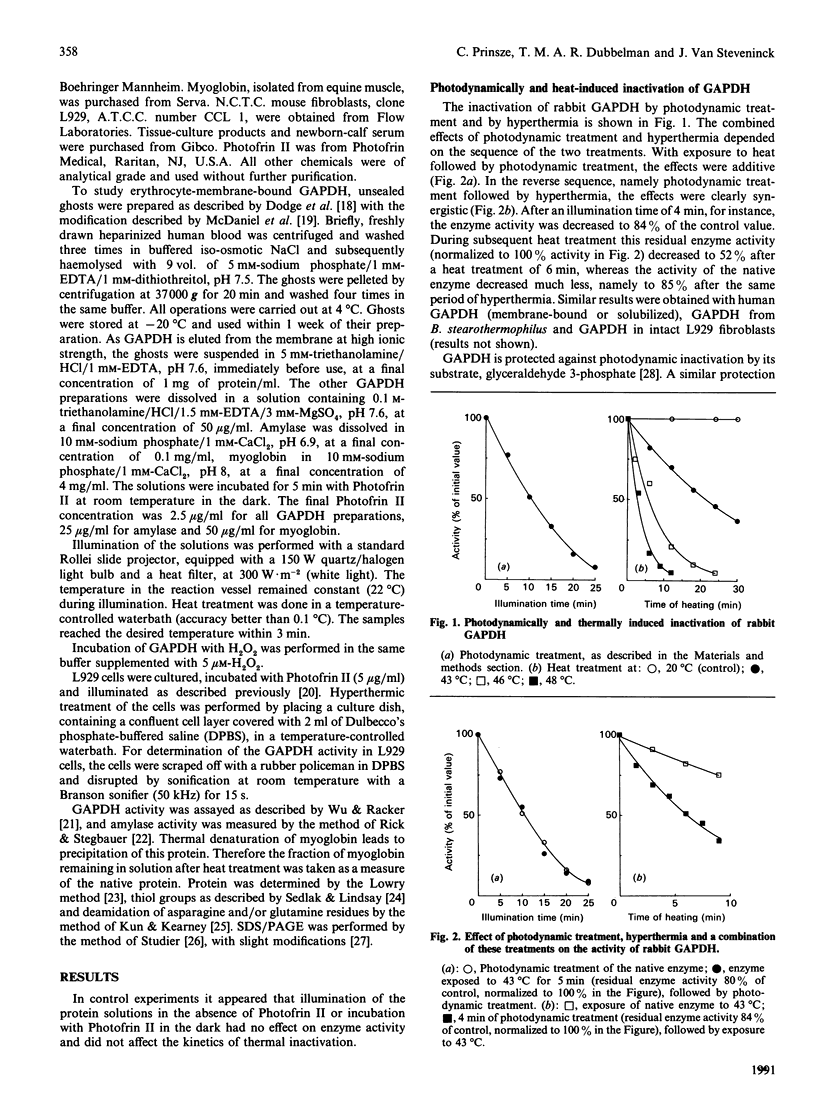
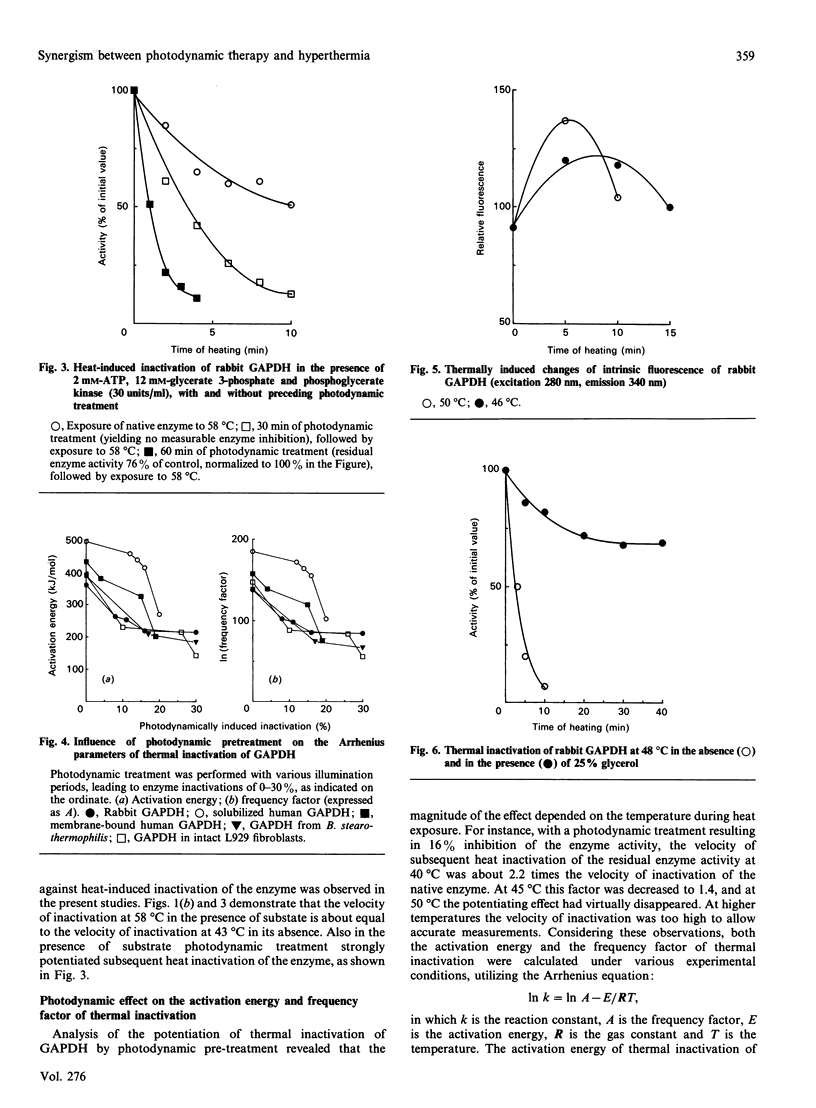
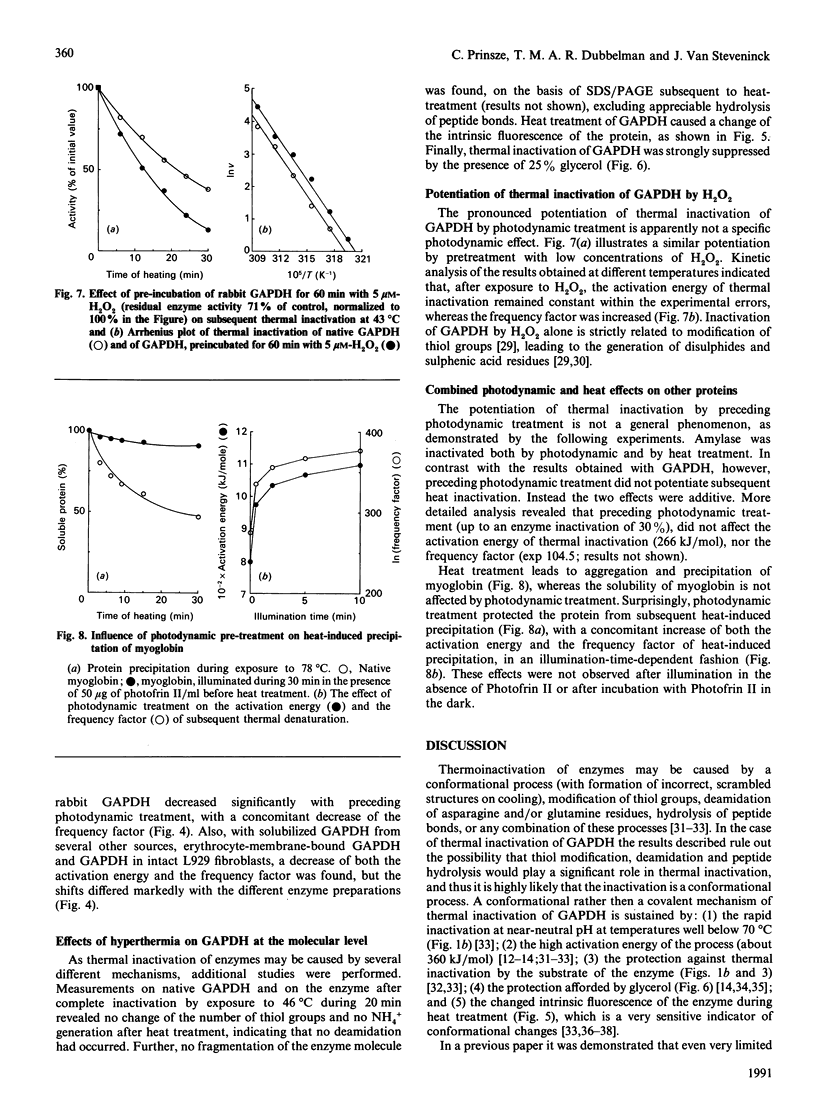
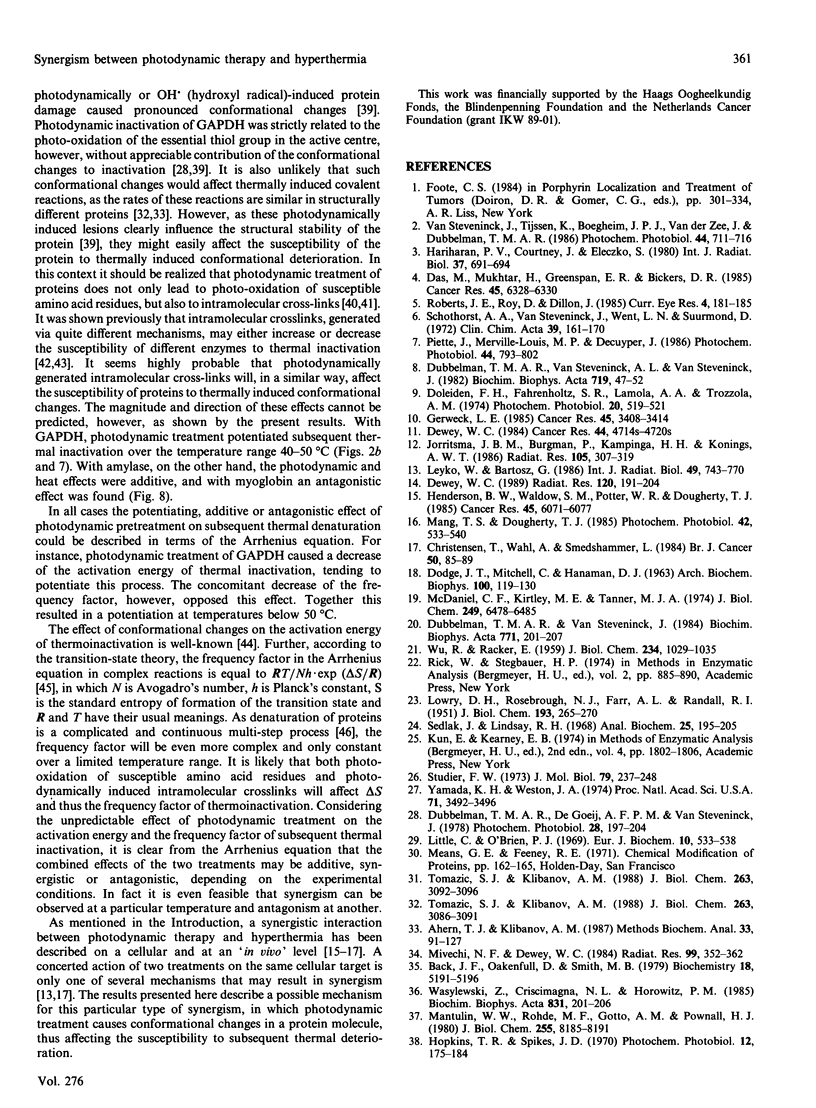
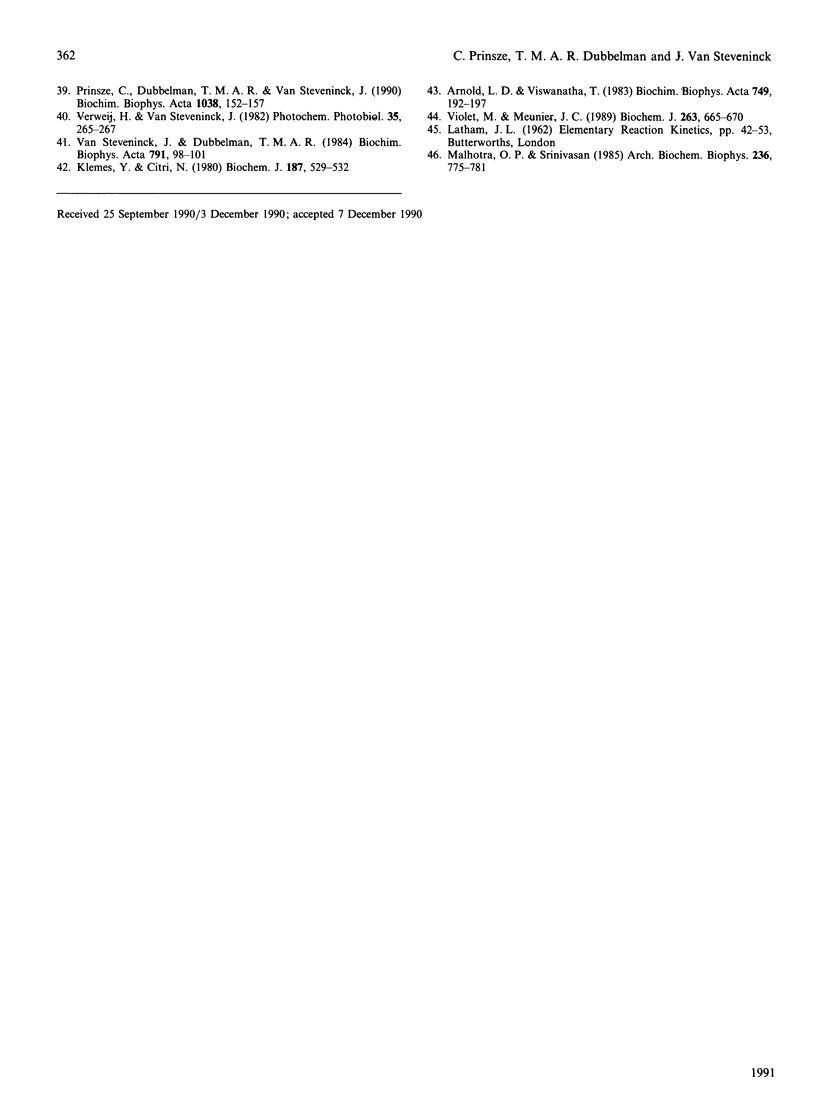
Selected References
These references are in PubMed. This may not be the complete list of references from this article.
- Ahern T. J., Klibanov A. M. Analysis of processes causing thermal inactivation of enzymes. Methods Biochem Anal. 1988;33:91–127. doi: 10.1002/9780470110546.ch3. [DOI] [PubMed] [Google Scholar]
- Arnold L. D., Viswanatha T. Thermal denaturation of native and cross-linked Bacillus cereus 569/H beta-lactamase I. Biochim Biophys Acta. 1983 Dec 12;749(2):192–197. doi: 10.1016/0167-4838(83)90252-2. [DOI] [PubMed] [Google Scholar]
- Back J. F., Oakenfull D., Smith M. B. Increased thermal stability of proteins in the presence of sugars and polyols. Biochemistry. 1979 Nov 13;18(23):5191–5196. doi: 10.1021/bi00590a025. [DOI] [PubMed] [Google Scholar]
- Christensen T., Wahl A., Smedshammer L. Effects of haematoporphyrin derivative and light in combination with hyperthermia on cells in culture. Br J Cancer. 1984 Jul;50(1):85–89. doi: 10.1038/bjc.1984.142. [DOI] [PMC free article] [PubMed] [Google Scholar]
- DODGE J. T., MITCHELL C., HANAHAN D. J. The preparation and chemical characteristics of hemoglobin-free ghosts of human erythrocytes. Arch Biochem Biophys. 1963 Jan;100:119–130. doi: 10.1016/0003-9861(63)90042-0. [DOI] [PubMed] [Google Scholar]
- Das M., Mukhtar H., Greenspan E. R., Bickers D. R. Photoenhancement of lipid peroxidation associated with the generation of reactive oxygen species in hepatic microsomes of hematoporphyrin derivative-treated rats. Cancer Res. 1985 Dec;45(12 Pt 1):6328–6330. [PubMed] [Google Scholar]
- Dewey W. C. Failla memorial lecture. The search for critical cellular targets damaged by heat. Radiat Res. 1989 Nov;120(2):191–204. [PubMed] [Google Scholar]
- Dewey W. C. Interaction of heat with radiation and chemotherapy. Cancer Res. 1984 Oct;44(10 Suppl):4714s–4720s. [PubMed] [Google Scholar]
- Doleiden F. H., Fahrenholtz S. R., Lamola A. A., Trozzolo A. M. Reactivity of cholesterol and some fatty acids toward singlet oxygen. Photochem Photobiol. 1974 Dec;20(6):519–521. doi: 10.1111/j.1751-1097.1974.tb06613.x. [DOI] [PubMed] [Google Scholar]
- Dubbelman T. M., Van Steveninck J. Photodynamic effects of hematoporphyrin-derivative on transmembrane transport systems of murine L929 fibroblasts. Biochim Biophys Acta. 1984 Apr 11;771(2):201–207. doi: 10.1016/0005-2736(84)90534-0. [DOI] [PubMed] [Google Scholar]
- Dubbelman T. M., de Goeij A. F., van Steveninck J. Protoporphyrin-sensitized photodynamic modification of proteins in isolated human red blood cell membranes. Photochem Photobiol. 1978 Aug;28(2):197–204. doi: 10.1111/j.1751-1097.1978.tb07695.x. [DOI] [PubMed] [Google Scholar]
- Gerweck L. E. Hyperthermia in cancer therapy: the biological basis and unresolved questions. Cancer Res. 1985 Aug;45(8):3408–3414. [PubMed] [Google Scholar]
- Hariharan P. V., Courtney J., Eleczko S. Production of hydroxyl radicals in cell systems exposed to haematoporphyrin and red light. Int J Radiat Biol Relat Stud Phys Chem Med. 1980 Jun;37(6):691–694. [PubMed] [Google Scholar]
- Henderson B. W., Waldow S. M., Potter W. R., Dougherty T. J. Interaction of photodynamic therapy and hyperthermia: tumor response and cell survival studies after treatment of mice in vivo. Cancer Res. 1985 Dec;45(12 Pt 1):6071–6077. [PubMed] [Google Scholar]
- Hopkins T. R., Spikes J. D. Conformational changes of lysozyme during photodynamic inactivation. Photochem Photobiol. 1970 Sep;12(3):175–184. doi: 10.1111/j.1751-1097.1970.tb06049.x. [DOI] [PubMed] [Google Scholar]
- Jorritsma J. B., Burgman P., Kampinga H. H., Konings A. W. DNA polymerase activity in heat killing and hyperthermic radiosensitization of mammalian cells as observed after fractionated heat treatments. Radiat Res. 1986 Mar;105(3):307–319. [PubMed] [Google Scholar]
- Klemes Y., Citri N. Cross-linking preserves conformational changes induced in penicillinase by its substrates. Biochem J. 1980 May 1;187(2):529–532. doi: 10.1042/bj1870529. [DOI] [PMC free article] [PubMed] [Google Scholar]
- LOWRY O. H., ROSEBROUGH N. J., FARR A. L., RANDALL R. J. Protein measurement with the Folin phenol reagent. J Biol Chem. 1951 Nov;193(1):265–275. [PubMed] [Google Scholar]
- Leyko W., Bartosz G. Membrane effects of ionizing radiation and hyperthermia. Int J Radiat Biol Relat Stud Phys Chem Med. 1986 May;49(5):743–770. doi: 10.1080/09553008514552971. [DOI] [PubMed] [Google Scholar]
- Little C., O'Brien P. J. Mechanism of peroxide-inactivation of the sulphydryl enzyme glyceraldehyde-3-phosphate dehydrogenase. Eur J Biochem. 1969 Oct;10(3):533–538. doi: 10.1111/j.1432-1033.1969.tb00721.x. [DOI] [PubMed] [Google Scholar]
- Malhotra O. P., Srinivasan pH-dependent conformational transformation in mung bean glyceraldehyde-3-phosphate dehydrogenase. Arch Biochem Biophys. 1985 Feb 1;236(2):775–781. doi: 10.1016/0003-9861(85)90683-6. [DOI] [PubMed] [Google Scholar]
- Mang T. S., Dougherty T. J. Time and sequence dependent influence of in vitro photodynamic therapy (PDT) survival by hyperthermia. Photochem Photobiol. 1985 Nov;42(5):533–540. doi: 10.1111/j.1751-1097.1985.tb01606.x. [DOI] [PubMed] [Google Scholar]
- Mantulin W. W., Rohde M. F., Gotto A. M., Jr, Pownall H. J. The conformational properties of human plasma apolipoprotein C-II. A spectroscopic study. J Biol Chem. 1980 Sep 10;255(17):8185–8191. [PubMed] [Google Scholar]
- McDaniel C. F., Kirtley M. E., Tanner M. J. The interaction of glyceraldehyde 3-phosphate dehydrogenase with human erythrocyte membranes. J Biol Chem. 1974 Oct 25;249(20):6478–6485. [PubMed] [Google Scholar]
- Mivechi N. F., Dewey W. C. Effect of glycerol and low pH on heat-induced cell killing and loss of cellular DNA polymerase activities in Chinese hamster ovary cells. Radiat Res. 1984 Aug;99(2):352–362. [PubMed] [Google Scholar]
- Piette J., Merville-Louis M. P., Decuyper J. Damages induced in nucleic acids by photosensitization. Photochem Photobiol. 1986 Dec;44(6):793–802. doi: 10.1111/j.1751-1097.1986.tb05539.x. [DOI] [PubMed] [Google Scholar]
- Prinsze C., Dubbelman T. M., Van Steveninck J. Protein damage, induced by small amounts of photodynamically generated singlet oxygen or hydroxyl radicals. Biochim Biophys Acta. 1990 Apr 19;1038(2):152–157. doi: 10.1016/0167-4838(90)90198-o. [DOI] [PubMed] [Google Scholar]
- Roberts J. E., Roy D., Dillon J. The photosensitized oxidation of the calf lens main intrinsic protein (MP26) with hematoporphyrin. Curr Eye Res. 1985 Mar;4(3):181–185. doi: 10.3109/02713688509000848. [DOI] [PubMed] [Google Scholar]
- Schothorst A. A., van Steveninck J., Went L. N., Suurmond D. Photodynamic damage of the erythrocyte membrane caused by protoporphyrin in protoporphyria and in normal red blood cells. Clin Chim Acta. 1972 Jun;39(1):161–170. doi: 10.1016/0009-8981(72)90312-9. [DOI] [PubMed] [Google Scholar]
- Sedlak J., Lindsay R. H. Estimation of total, protein-bound, and nonprotein sulfhydryl groups in tissue with Ellman's reagent. Anal Biochem. 1968 Oct 24;25(1):192–205. doi: 10.1016/0003-2697(68)90092-4. [DOI] [PubMed] [Google Scholar]
- Studier F. W. Analysis of bacteriophage T7 early RNAs and proteins on slab gels. J Mol Biol. 1973 Sep 15;79(2):237–248. doi: 10.1016/0022-2836(73)90003-x. [DOI] [PubMed] [Google Scholar]
- Tomazic S. J., Klibanov A. M. Mechanisms of irreversible thermal inactivation of Bacillus alpha-amylases. J Biol Chem. 1988 Mar 5;263(7):3086–3091. [PubMed] [Google Scholar]
- Tomazic S. J., Klibanov A. M. Why is one Bacillus alpha-amylase more resistant against irreversible thermoinactivation than another? J Biol Chem. 1988 Mar 5;263(7):3092–3096. [PubMed] [Google Scholar]
- Van Steveninck J., Tijssen K., Boegheim J. P., Van der Zee J., Dubbelman T. M. Photodynamic generation of hydroxyl radicals by hematoporphyrin derivative and light. Photochem Photobiol. 1986 Dec;44(6):711–716. doi: 10.1111/j.1751-1097.1986.tb05528.x. [DOI] [PubMed] [Google Scholar]
- Violet M., Meunier J. C. Kinetic study of the irreversible thermal denaturation of Bacillus licheniformis alpha-amylase. Biochem J. 1989 Nov 1;263(3):665–670. doi: 10.1042/bj2630665. [DOI] [PMC free article] [PubMed] [Google Scholar]
- WU R., RACKER E. Regulatory mechanisms in carbohydrate metabolism. III. Limiting factors in glycolysis of ascites tumor cells. J Biol Chem. 1959 May;234(5):1029–1035. [PubMed] [Google Scholar]
- Wasylewski Z., Criscimagna N. L., Horowitz P. M. A fluorescence study of thermally induced conformational changes in yeast hexokinase. Biochim Biophys Acta. 1985 Oct 4;831(2):201–206. doi: 10.1016/0167-4838(85)90036-6. [DOI] [PubMed] [Google Scholar]
- Yamada K. M., Weston J. A. Isolation of a major cell surface glycoprotein from fibroblasts. Proc Natl Acad Sci U S A. 1974 Sep;71(9):3492–3496. doi: 10.1073/pnas.71.9.3492. [DOI] [PMC free article] [PubMed] [Google Scholar]