Abstract
The relative significance of the flux through the glutamine aminotransferase (glutaminase II) pathway to renal ammoniagenesis is poorly understood. A basic and unresolved question is whether 2-oxoglutaramate (2-OGM), a product of the glutaminase II reaction, is deamidated to yield 2-oxoglutarate and NH3, or whether 2-OGM accumulates as an unreactive lactam, depending on the environmental pH. In the current studies we utilized 13C n.m.r. as well as 15N n.m.r. as well as 15N n.m.r. to demonstrate that 2-OGM occurs as a lactam, i.e. 5-hydroxypyroglutamate, regardless of the environmental pH. Our additional aims were to determine whether human kidney cells (HK cells) in culture can produce 2-OGM and to ascertain a pH-dependent relationship between NH3 and 2-OGM production from glutamine. We therefore developed an isotope dilution assay for 2-OGM utilizing 5-hydroxy[4-13C,1-15N]pyroglutamate as the labelled species. Incubations of HK cells in minimal essential medium supplemented with 1 mM-[2-15N]glutamine demonstrated significantly higher production of 2-OGM at pH 6.8 and lower production at pH 7.6 compared with pH 7.4. Similarly both 15NH3 and [15N]alanine formation were significantly higher in acute acidosis (pH 6.8) and lower in acute alkalosis (pH 7.6) compared with that at physiological pH. Addition of 1 mM-amino-oxyacetate to the incubation medium at pH 7.4 significantly diminished [15N]alanine and 2-OGM production, but the production of 15NH3 via the glutamate dehydrogenase pathway was significantly stimulated. The current observations indicate that the glutaminase II pathway plays a minor role and that flux through glutamate dehydrogenase is the predominant site for regulation of ammoniagenesis in human kidney.
Full text
PDF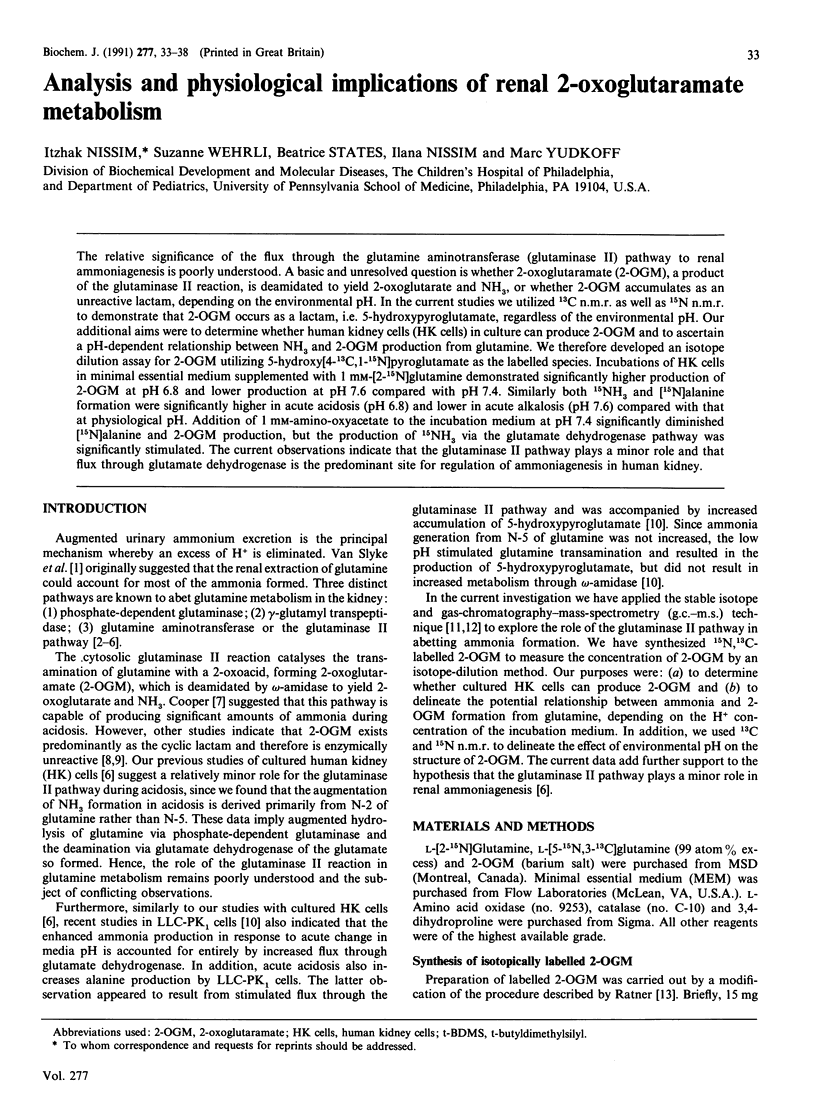
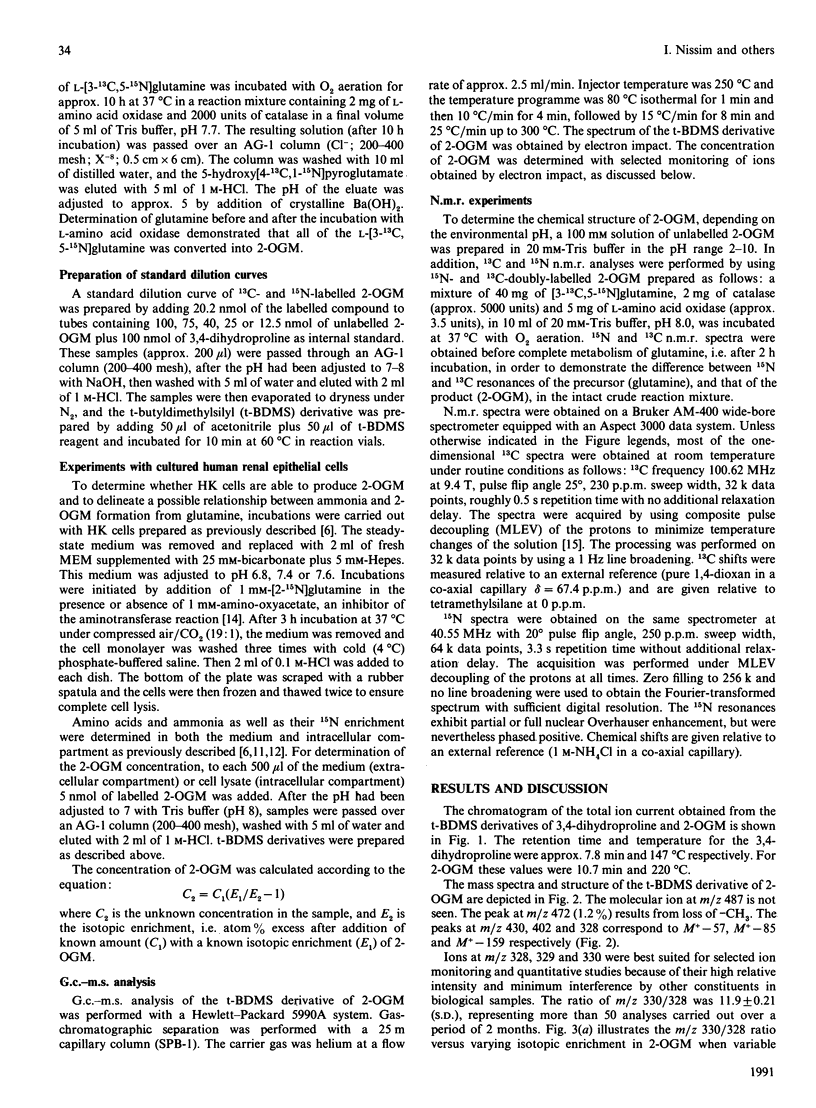
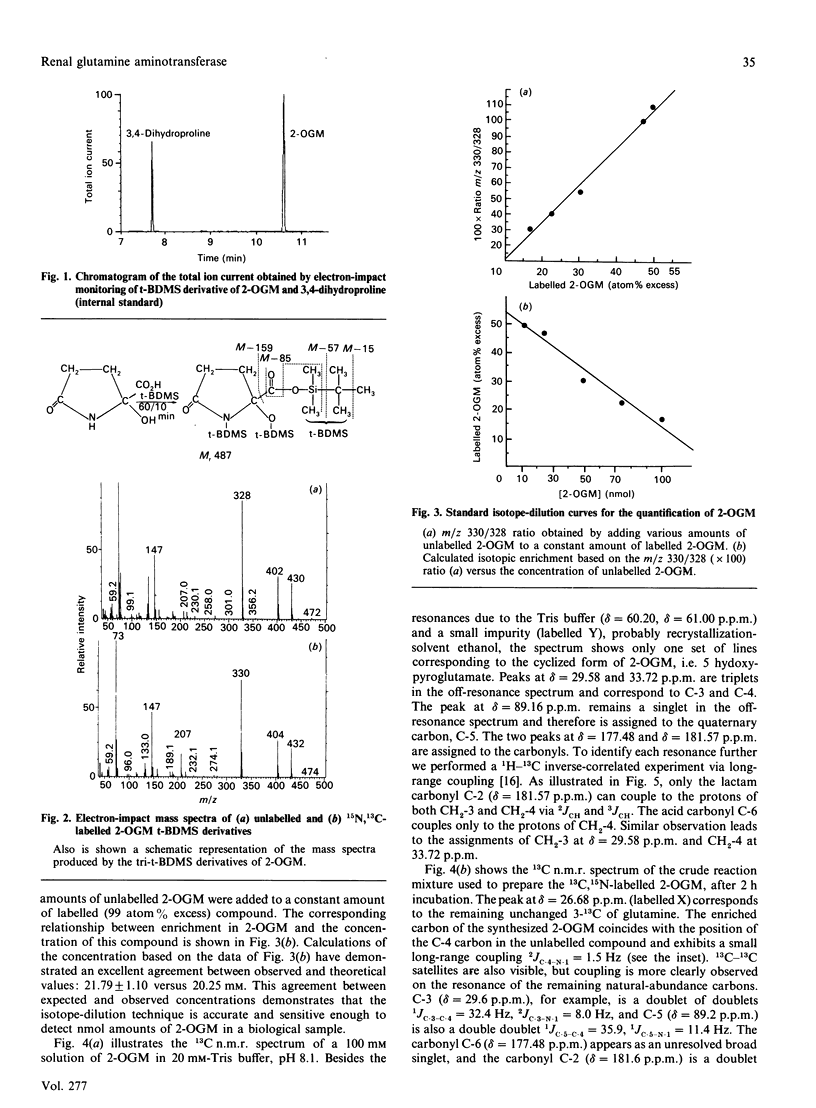
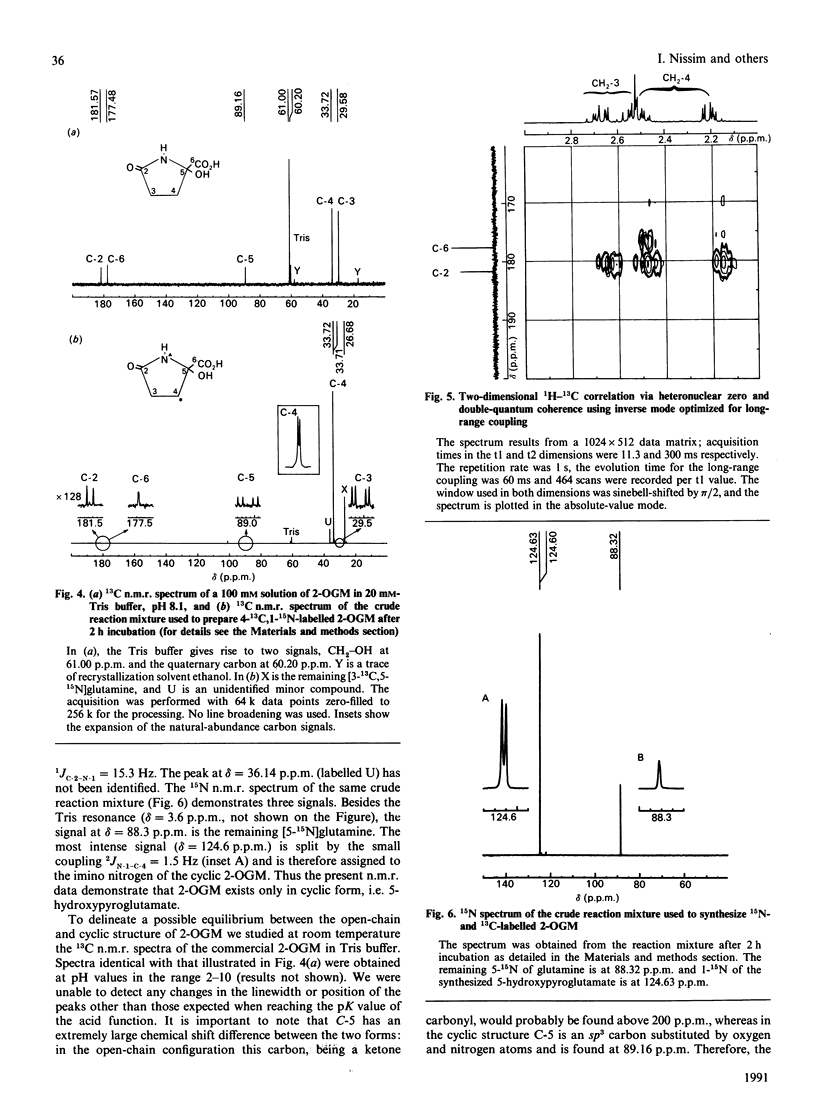
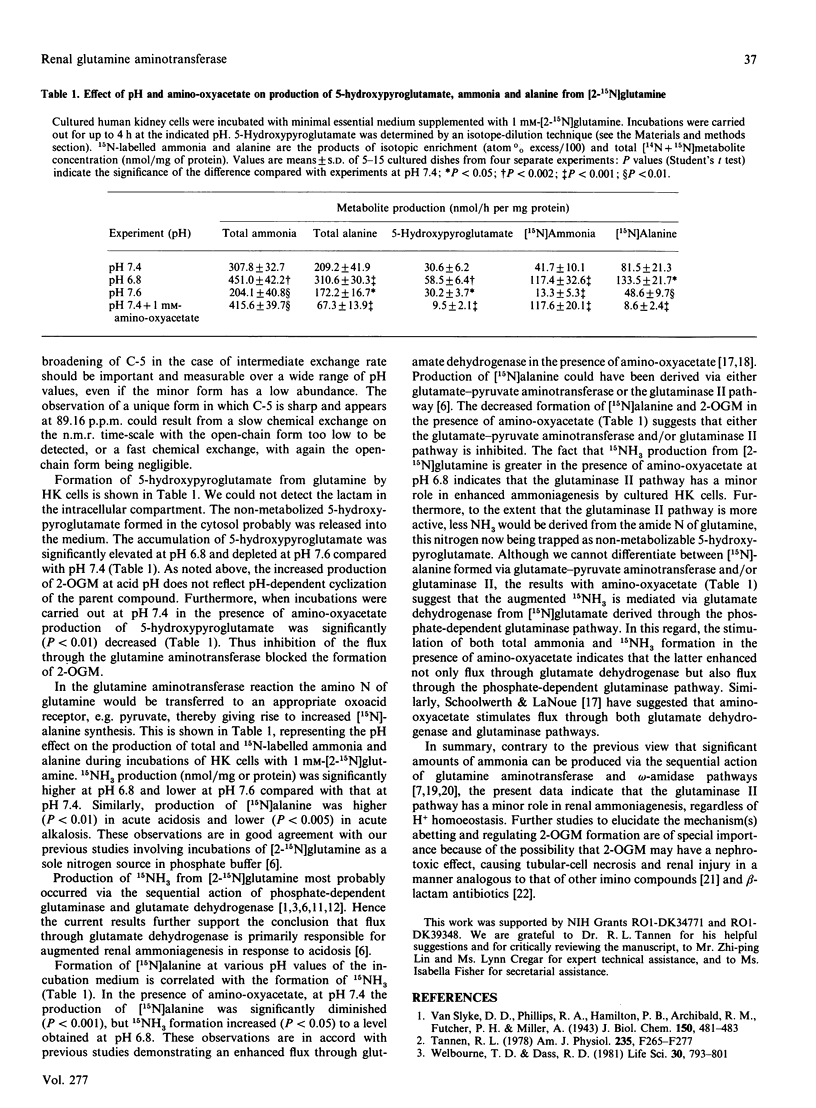
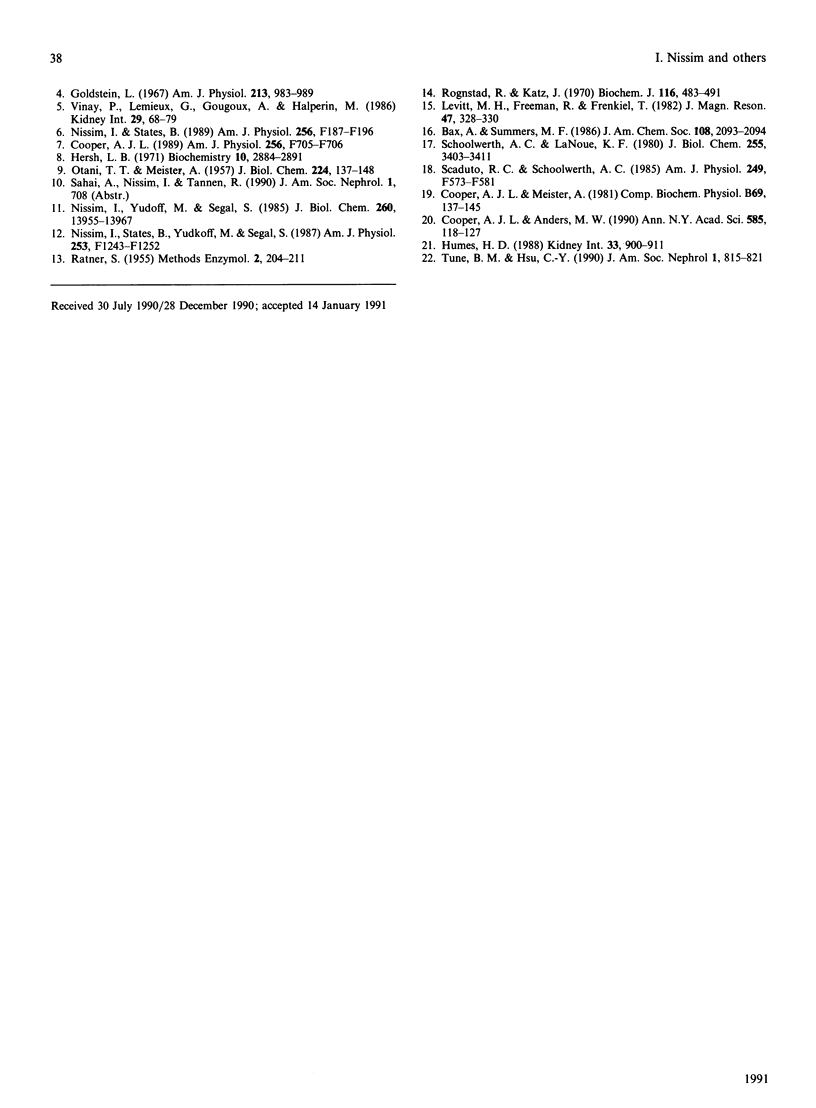
Selected References
These references are in PubMed. This may not be the complete list of references from this article.
- Cooper A. J. Ammoniagenesis in cultured human renal cortical epithelial cells. Am J Physiol. 1989 Oct;257(4 Pt 2):F705–F706. doi: 10.1152/ajprenal.1989.257.4.F705. [DOI] [PubMed] [Google Scholar]
- Cooper A. J., Anders M. W. Glutamine transaminase K and cysteine conjugate beta-lyase. Ann N Y Acad Sci. 1990;585:118–127. doi: 10.1111/j.1749-6632.1990.tb28048.x. [DOI] [PubMed] [Google Scholar]
- Goldstein L. Pathways of glutamine deamination and their control in the rat kidney. Am J Physiol. 1967 Oct;213(4):983–989. doi: 10.1152/ajplegacy.1967.213.4.983. [DOI] [PubMed] [Google Scholar]
- Hersh L. B. Rat liver omega-amidase. Purification and properties. Biochemistry. 1971 Jul 20;10(15):2884–2891. doi: 10.1021/bi00791a014. [DOI] [PubMed] [Google Scholar]
- Humes H. D. Aminoglycoside nephrotoxicity. Kidney Int. 1988 Apr;33(4):900–911. doi: 10.1038/ki.1988.83. [DOI] [PubMed] [Google Scholar]
- MEISTER A., OTANI T. T. omega-Amide and omega-amino acid derivatives of alpha-ketoglutaric and oxalacetic acids. J Biol Chem. 1957 Jan;224(1):137–148. [PubMed] [Google Scholar]
- Nissim I., States B. Ammoniagenesis by cultured human renal cortical epithelial cells: study with 15N. Am J Physiol. 1989 Jan;256(1 Pt 2):F187–F196. doi: 10.1152/ajprenal.1989.256.1.F187. [DOI] [PubMed] [Google Scholar]
- Nissim I., States B., Yudkoff M., Segal S. Characterization of amino acid metabolism by cultured rat kidney cells: study with 15N. Am J Physiol. 1987 Dec;253(6 Pt 2):F1243–F1252. doi: 10.1152/ajprenal.1987.253.6.F1243. [DOI] [PubMed] [Google Scholar]
- Nissim I., Yudkoff M., Segal S. Metabolism of glutamine and glutamate by rat renal tubules. Study with 15N and gas chromatography-mass spectrometry. J Biol Chem. 1985 Nov 15;260(26):13955–13967. [PubMed] [Google Scholar]
- Rognstad R., Katz J. Gluconeogenesis in the kidney cortex. Effects of D-malate and amino-oxyacetate. Biochem J. 1970 Feb;116(3):483–491. doi: 10.1042/bj1160483. [DOI] [PMC free article] [PubMed] [Google Scholar]
- Scaduto R. C., Jr, Schoolwerth A. C. Effect of bicarbonate on glutamine and glutamate metabolism by rat kidney cortex mitochondria. Am J Physiol. 1985 Oct;249(4 Pt 2):F573–F581. doi: 10.1152/ajprenal.1985.249.4.F573. [DOI] [PubMed] [Google Scholar]
- Schoolwerth A. C., LaNoue K. F. The role of microcompartmentation in the regulation of glutamate metabolism by rat kidney mitochondria. J Biol Chem. 1980 Apr 25;255(8):3403–3411. [PubMed] [Google Scholar]
- Tannen R. L. Ammonia metabolism. Am J Physiol. 1978 Oct;235(4):F265–F277. doi: 10.1152/ajprenal.1978.235.4.F265. [DOI] [PubMed] [Google Scholar]
- Tune B. M., Hsu C. Y. The renal mitochondrial toxicity of beta-lactam antibiotics: in vitro effects of cephaloglycin and imipenem. J Am Soc Nephrol. 1990 Nov;1(5):815–821. doi: 10.1681/ASN.V15815. [DOI] [PubMed] [Google Scholar]
- Vinay P., Lemieux G., Gougoux A., Halperin M. Regulation of glutamine metabolism in dog kidney in vivo. Kidney Int. 1986 Jan;29(1):68–79. doi: 10.1038/ki.1986.9. [DOI] [PubMed] [Google Scholar]
- Welbourne T. C., Dass P. D. Function of renal gamma-glutamyltransferase: significance of glutathione and glutamine interactions. Life Sci. 1982 Mar 8;30(10):793–801. doi: 10.1016/0024-3205(82)90591-4. [DOI] [PubMed] [Google Scholar]