Abstract
Red cells exposed to t-butyl hydroperoxide undergo lipid peroxidation, haemoglobin degradation and hexose monophosphate-shunt stimulation. By using the lipid-soluble antioxidant 2,6-di-t-butyl-p-cresol, the relative contributions of t-butyl hydroperoxide and membrane lipid hydroperoxides to oxidative haemoglobin changes and hexose monophosphate-shunt stimulation were determined. About 90% of the haemoglobin changes and all of the hexose monophosphate-shunt stimulation were caused by t-butyl hydroperoxide. The remainder of the haemoglobin changes appeared to be due to reactions between haemoglobin and lipid hydroperoxides generated during membrane peroxidation. After exposure of red cells to t-butyl hydroperoxide, no lipid hydroperoxides were detected iodimetrically, whether or not glucose was present in the incubation. Concentrations of 2,6-di-t-butyl-p-cresol, which almost totally suppressed lipid peroxidation, significantly inhibited haemoglobin binding to the membrane but had no significant effect on hexose monophosphate shunt stimulation, suggesting that lipid hydroperoxides had been decomposed by a reaction with haem or haem-protein and not enzymically via glutathione peroxidase. The mechanisms of lipid peroxidation and haemoglobin oxidation and the protective role of glucose were also investigated. In time-course studies of red cells containing oxyhaemoglobin, methaemoglobin or carbonmono-oxyhaemoglobin incubated without glucose and exposed to t-butyl hydroperoxide, haemoglobin oxidation paralleled both lipid peroxidation and t-butyl hydroperoxide consumption. Lipid peroxidation ceased when all t-butyl hydroperoxide was consumed, indicating that it was not autocatalytic and was driven by initiation events followed by rapid propagation and termination of chain reactions and rapid non-enzymic decomposition of lipid hydroperoxides. Carbonmono-oxyhaemoglobin and oxyhaemoglobin were good promoters of peroxidation, whereas methaemoglobin relatively spared the membrane from peroxidation. The protective influence of glucose metabolism on the time course of t-butyl hydroperoxide-induced changes was greatest in carbonmono-oxyhaemoglobin-containing red cells followed in order by oxyhaemoglobin- and methaemoglobin-containing red cells. This is the reverse order of the reactivity of the hydroperoxide with haemoglobin, which is greatest with methaemoglobin. In studies exposing red cells to a wide range of t-butyl hydroperoxide concentrations, haemoglobin oxidation and lipid peroxidation did not occur until the cellular glutathione had been oxidized. The amount of lipid peroxidation per increment in added t-butyl hydroperoxide was greatest in red cells containing carbonmono-oxyhaemoglobin, followed in order by oxyhaemoglobin and methaemoglobin. Red cells containing oxyhaemoglobin and carbonmono-oxyhaemoglobin and exposed to increasing concentrations of t-butyl hydroperoxide became increasingly resistant to lipid peroxidation as methaemoglobin accumulated, supporting a relatively protective role for methaemoglobin. In the presence of glucose, higher levels of t-butyl hydroperoxide were required to induce lipid peroxidation and haemoglobin oxidation compared with incubations without glucose. Carbonmono-oxyhaemoglobin-containing red cells exposed to the highest levels of t-butyl hydroperoxide underwent haemolysis after a critical level of lipid peroxidation was reached. Inhibition of lipid peroxidation by 2,6-di-t-butyl-p-cresol below this critical level prevented haemolysis. Oxidative membrane damage appeared to be a more important determinant of haemolysis in vitro than haemoglobin degradation. The effects of various antioxidants and free-radical scavengers on lipid peroxidation in red cells or in ghosts plus methaemoglobin exposed to t-butyl hydroperoxide suggested that red-cell haemoglobin decomposed the hydroperoxide by a homolytic scission mechanism to t-butoxyl radicals.
Full text
PDF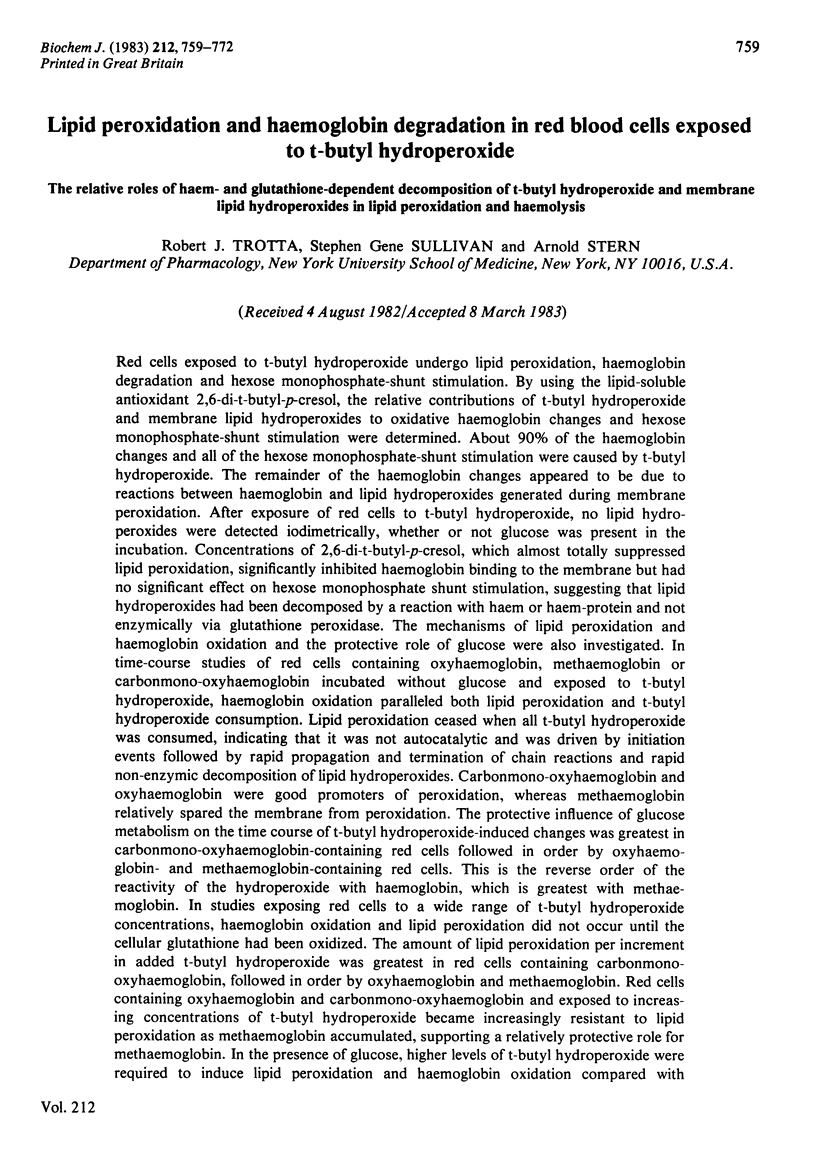
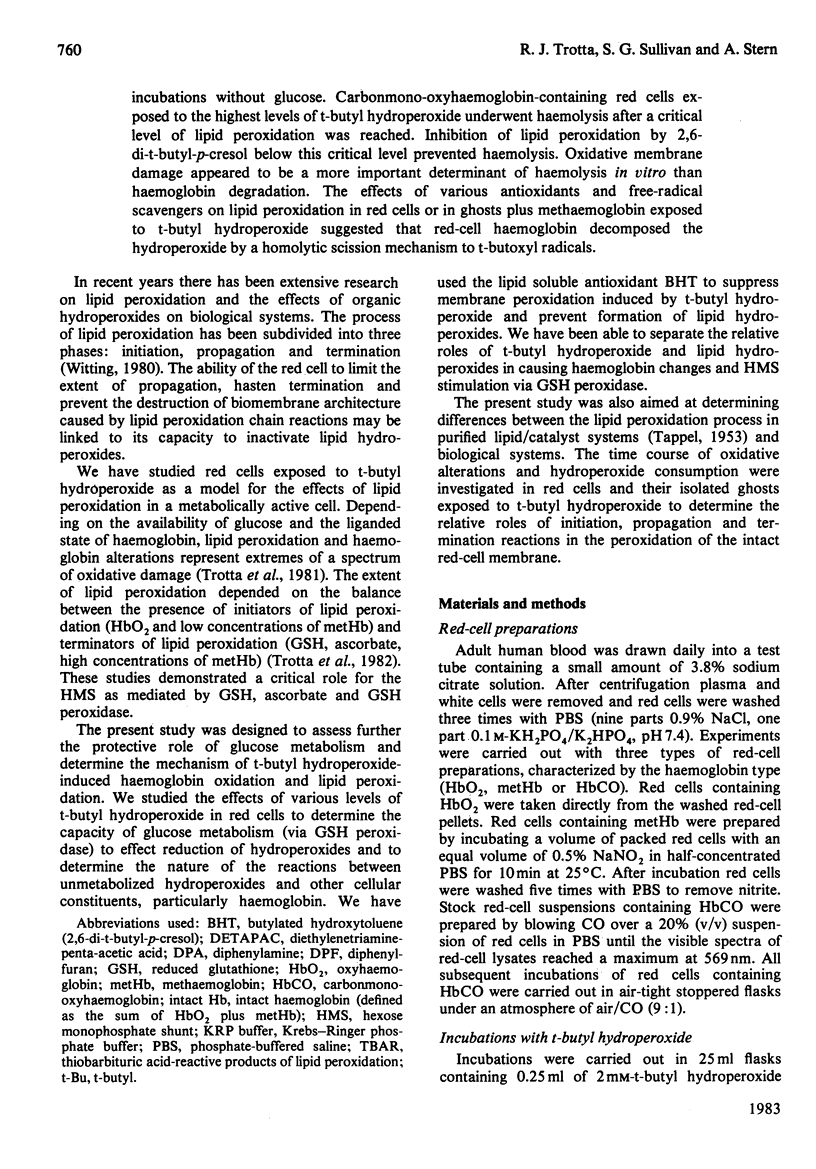
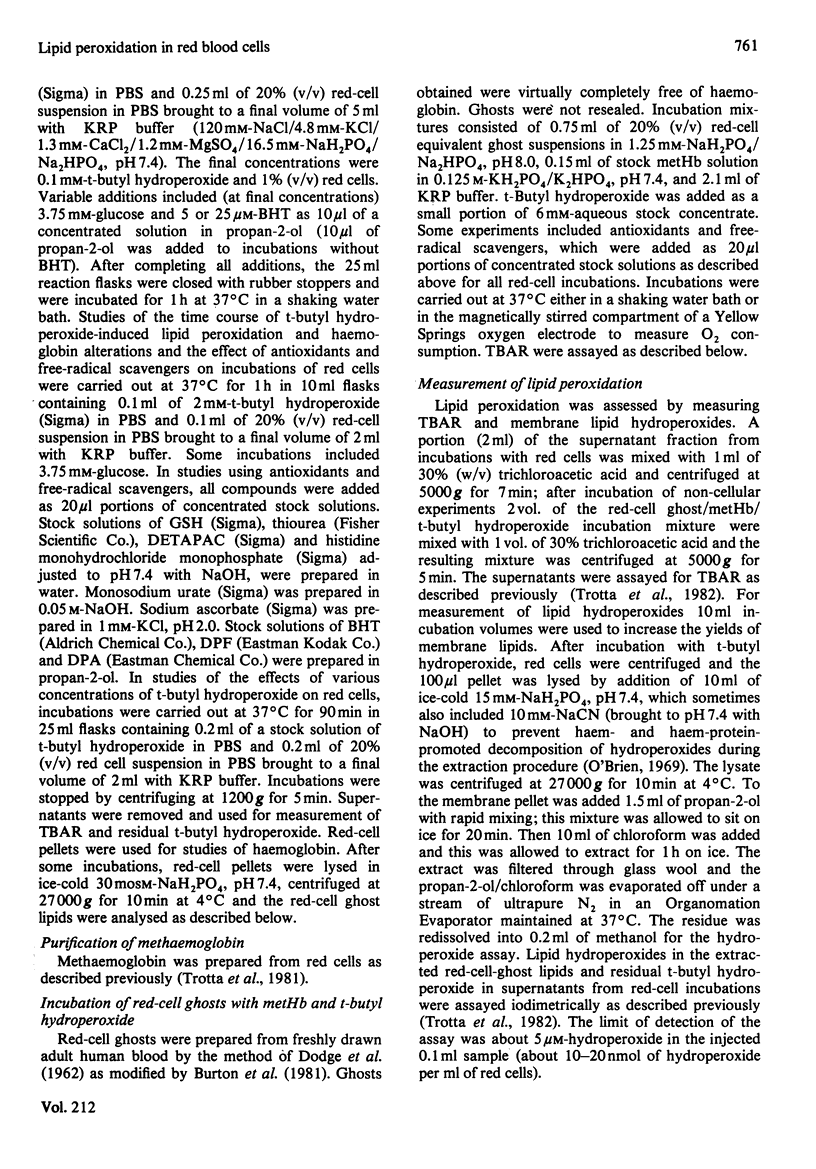
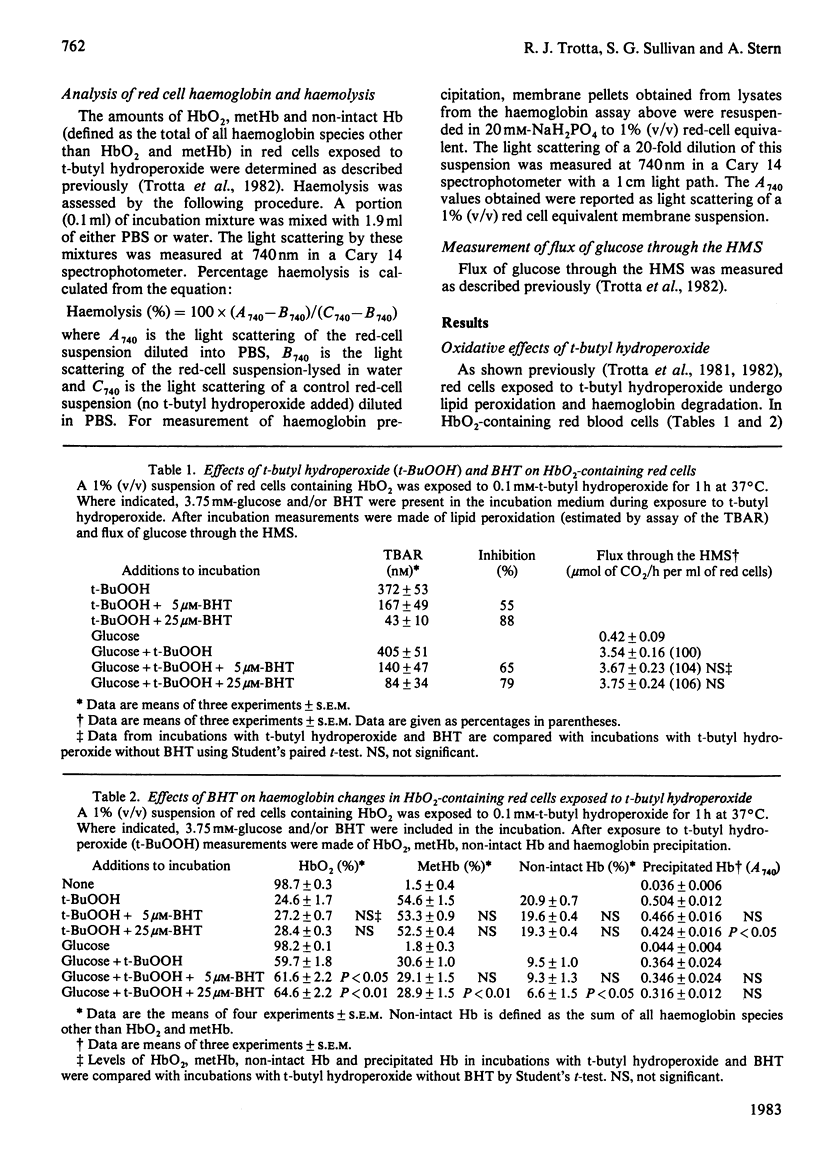
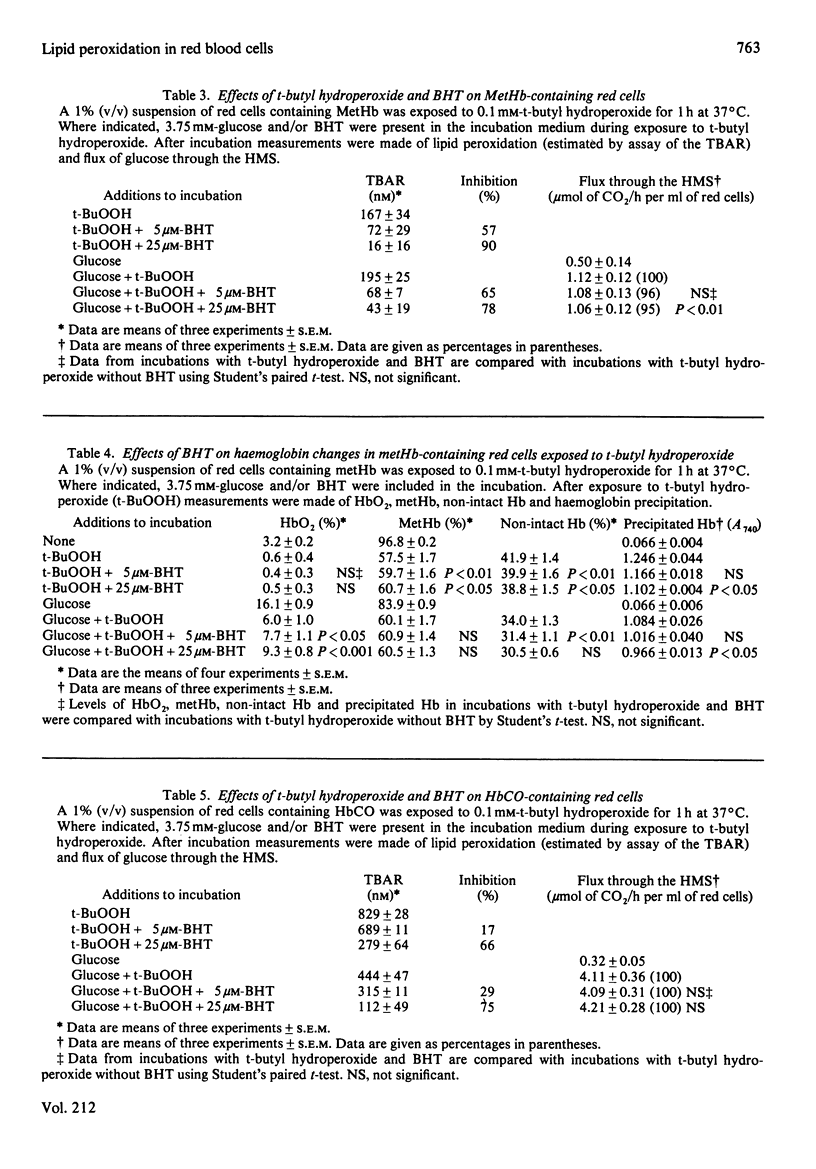
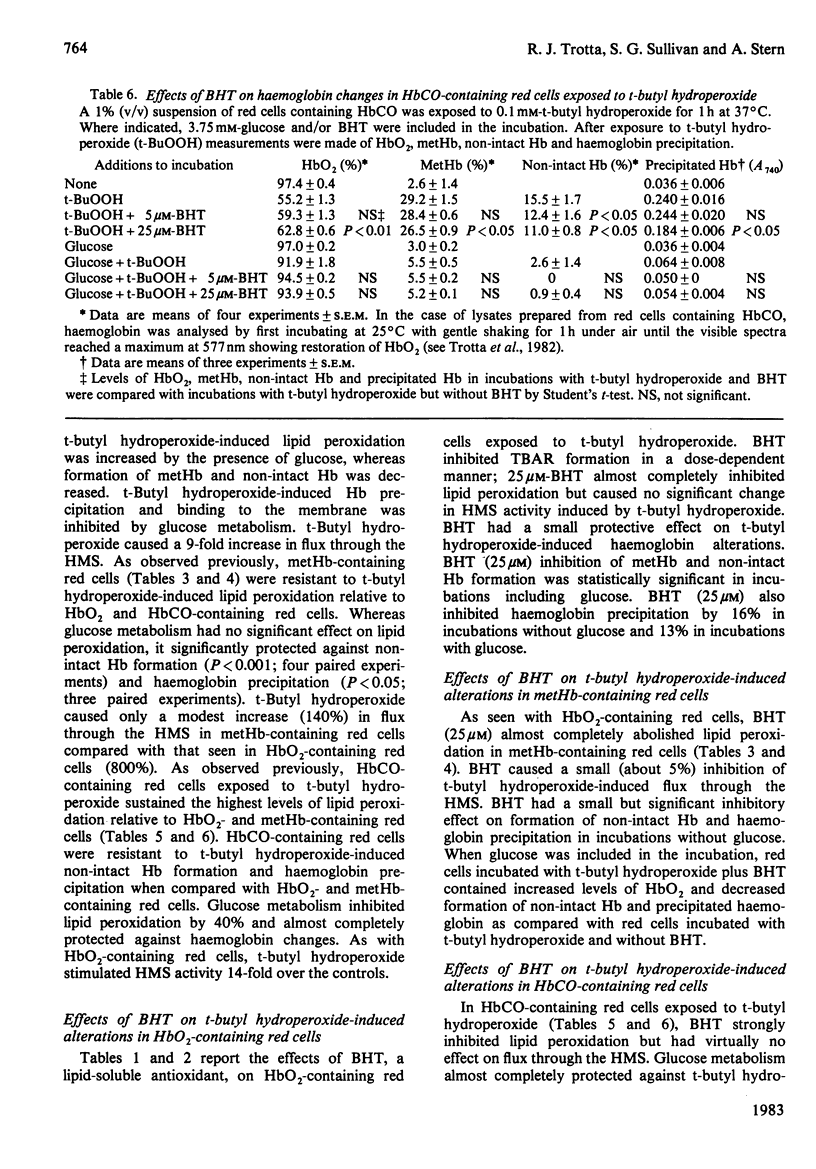
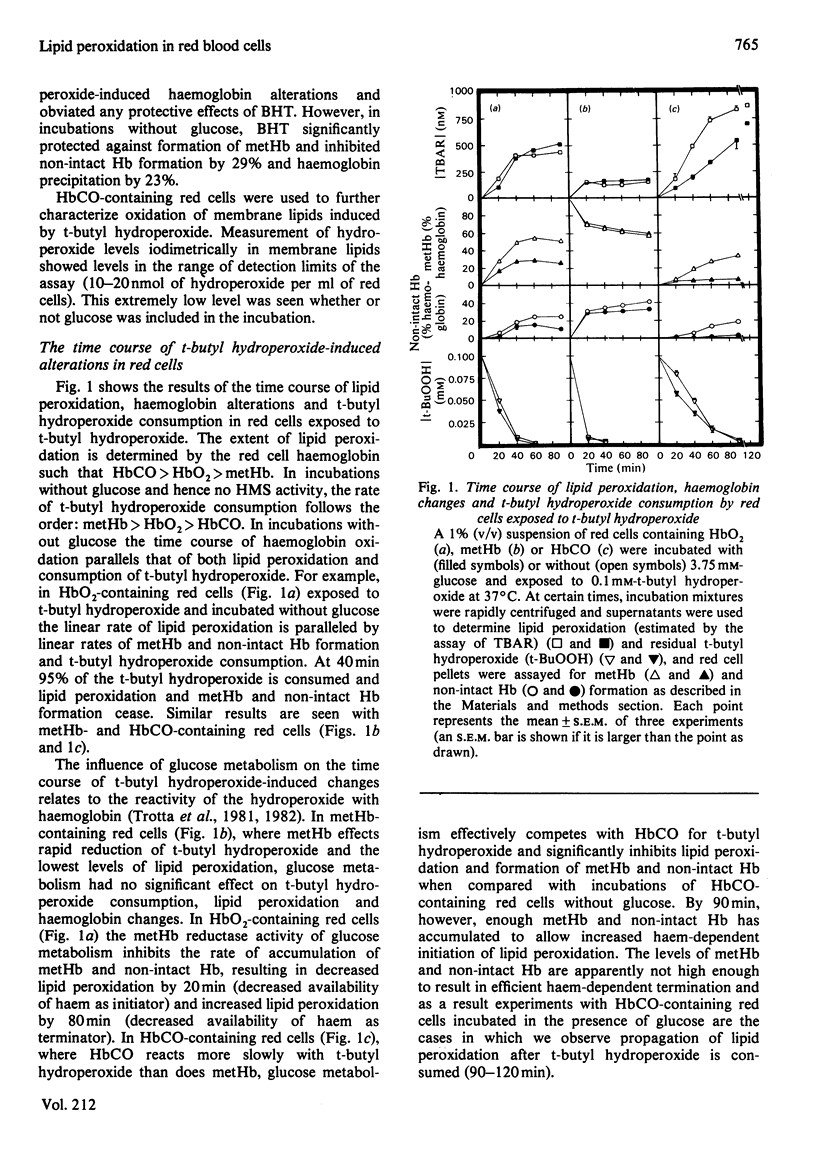
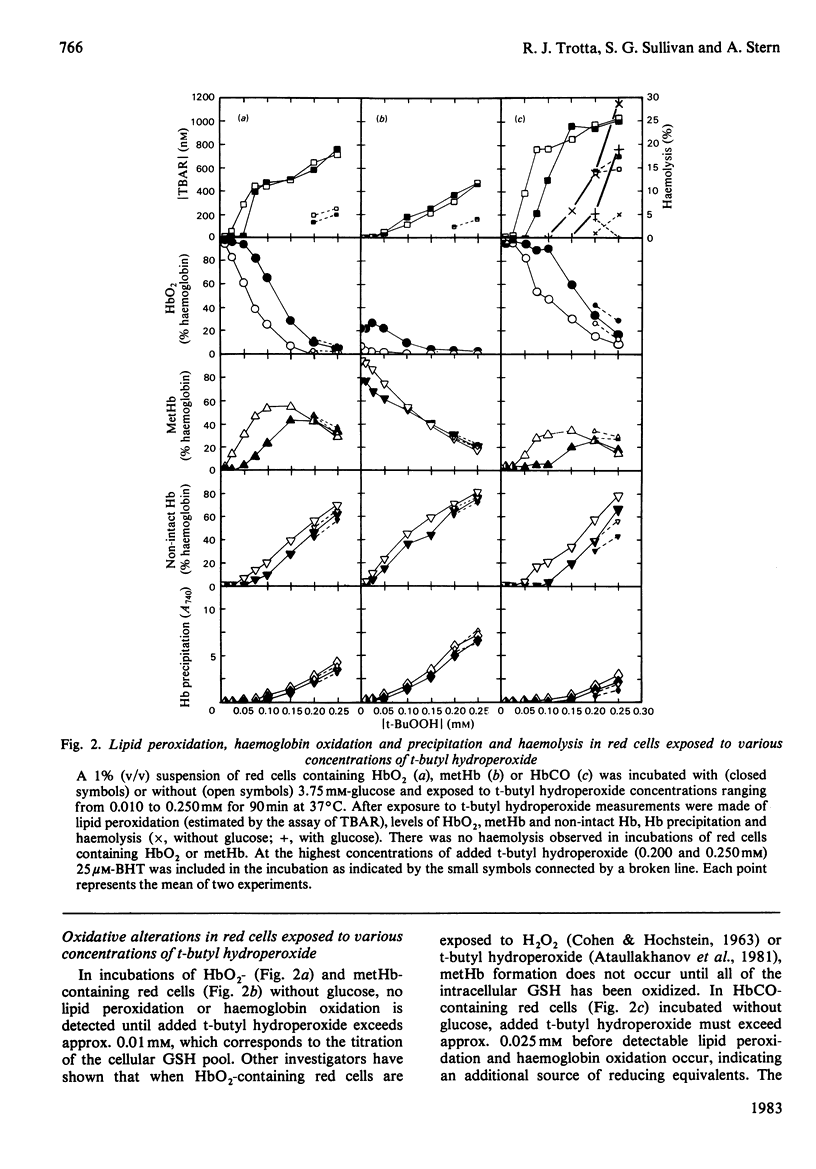
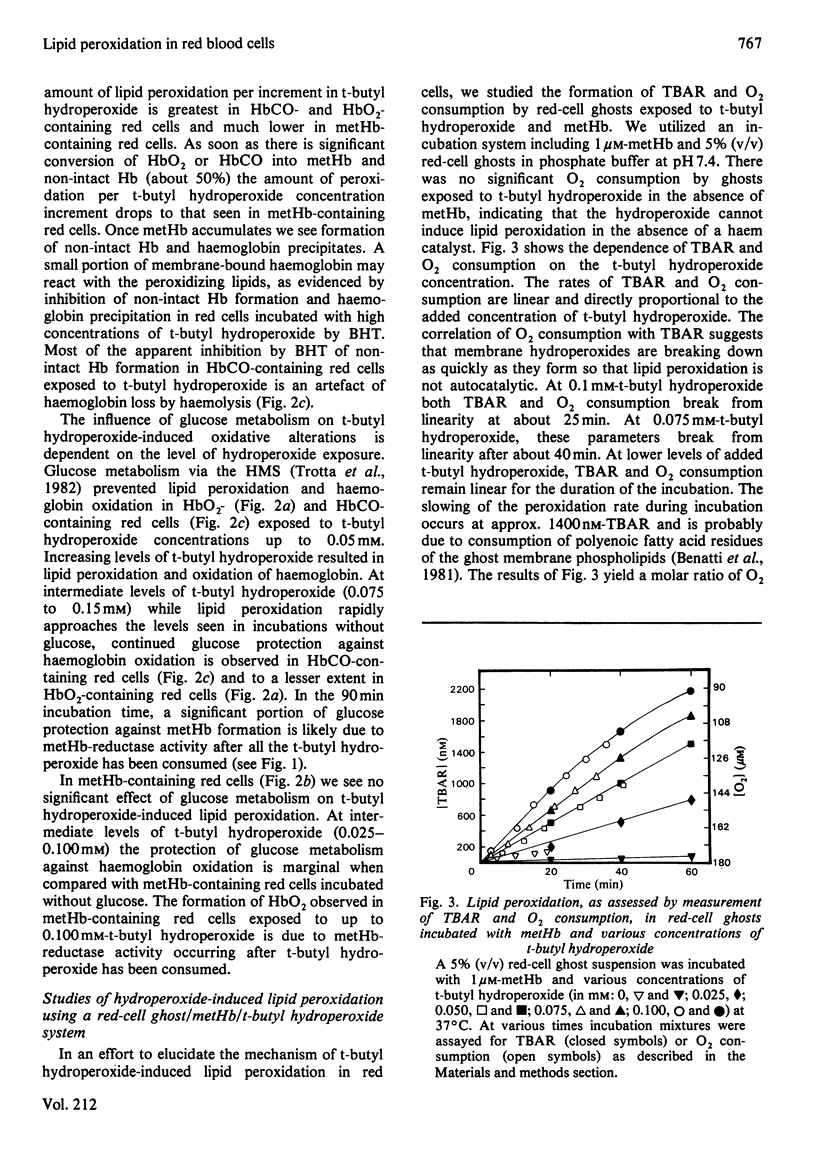
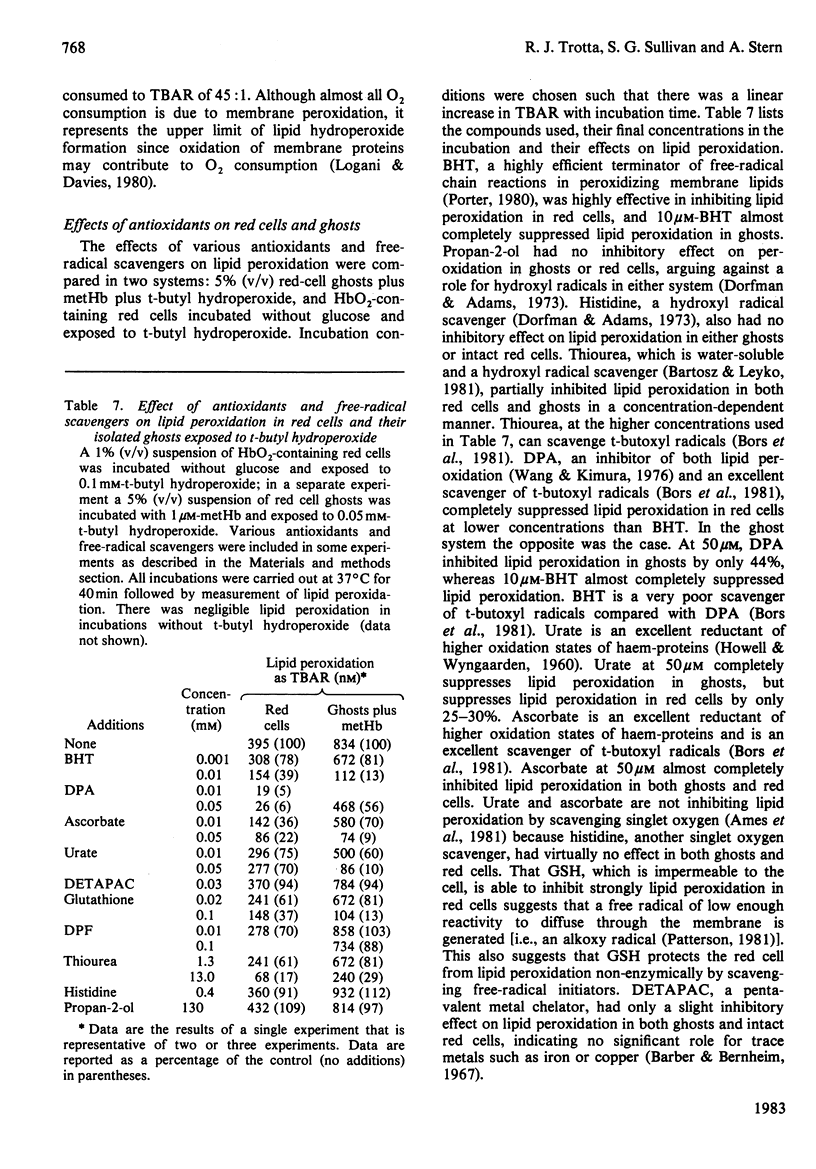
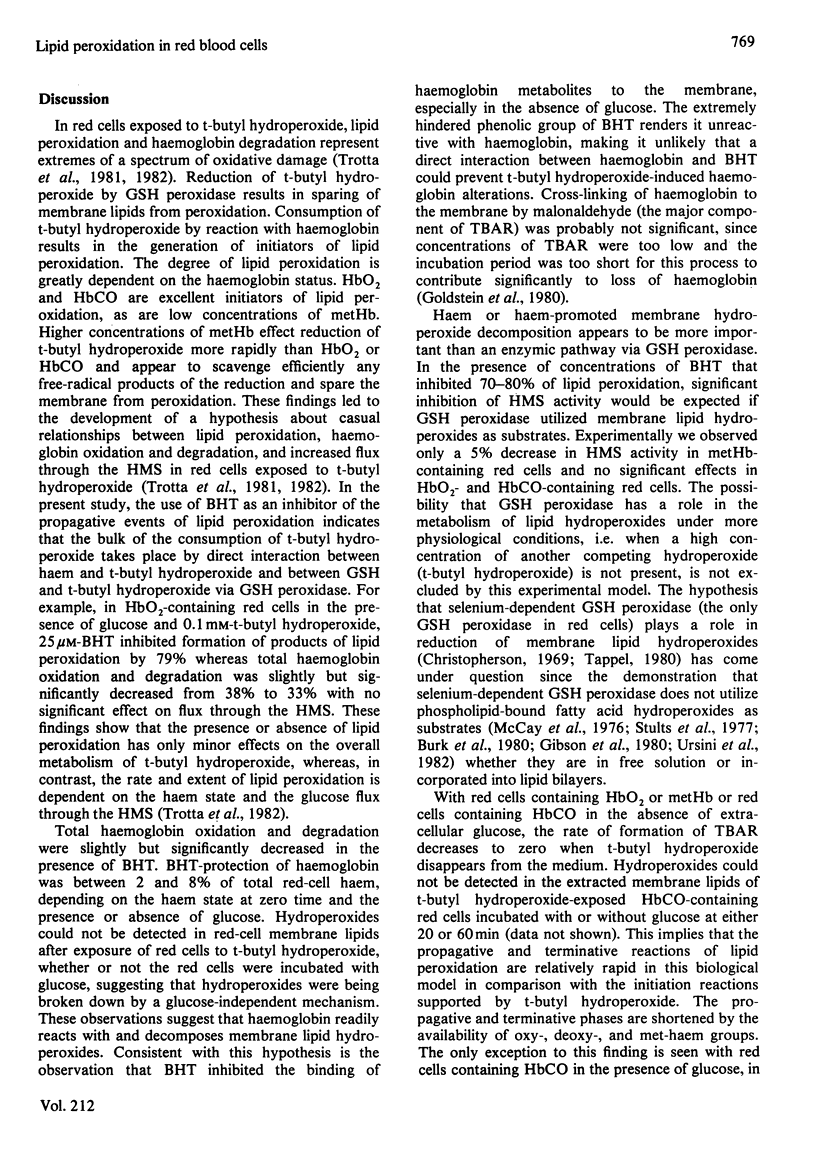
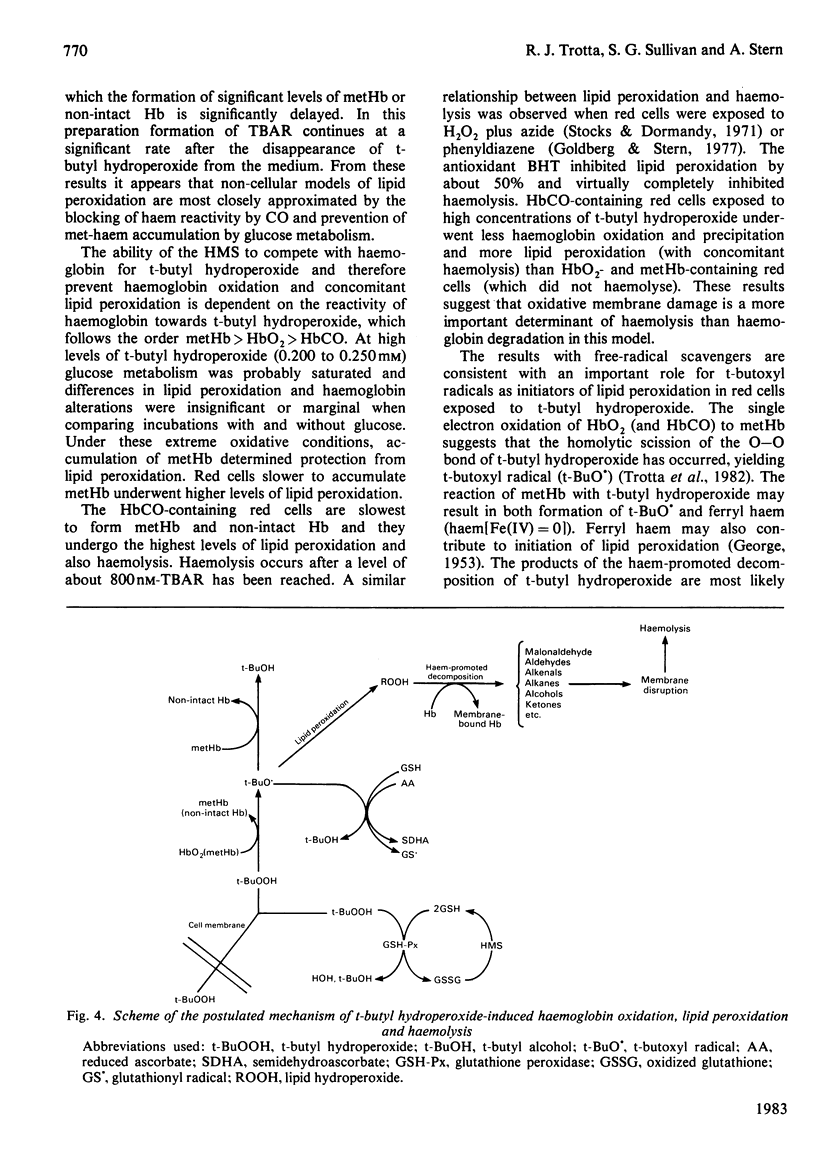
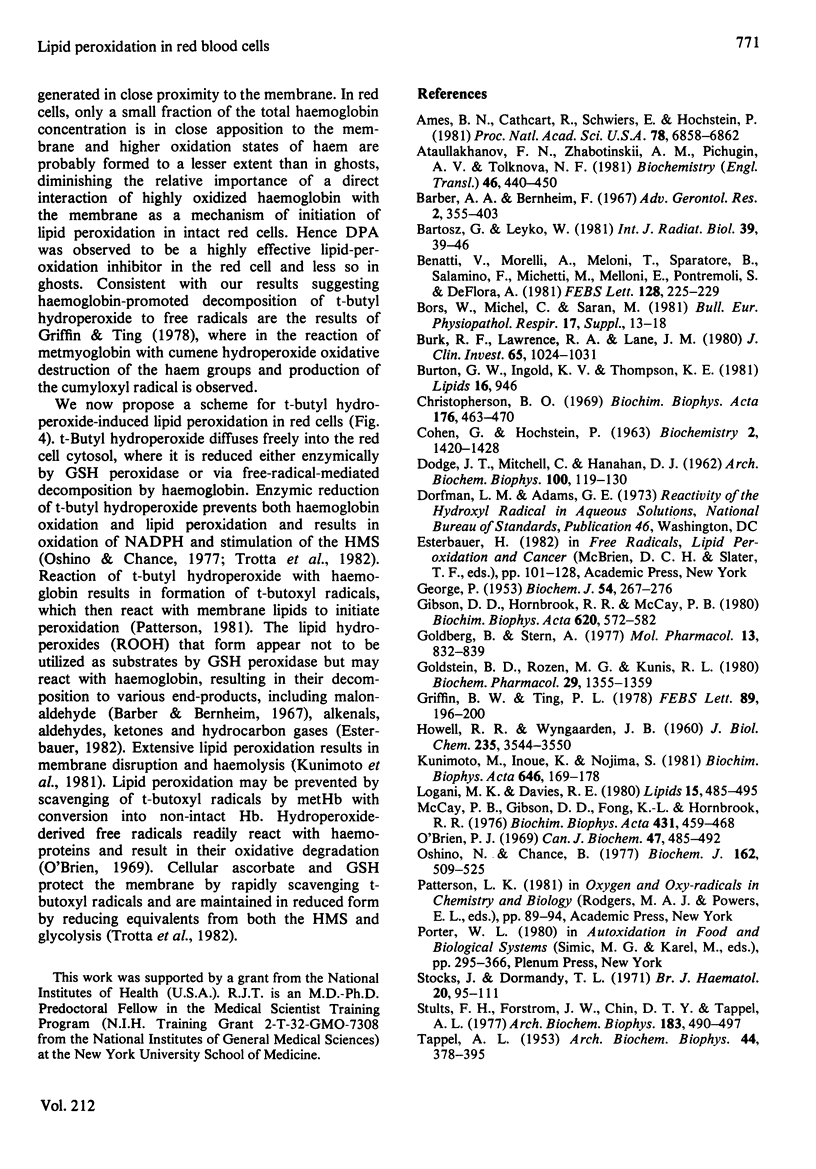
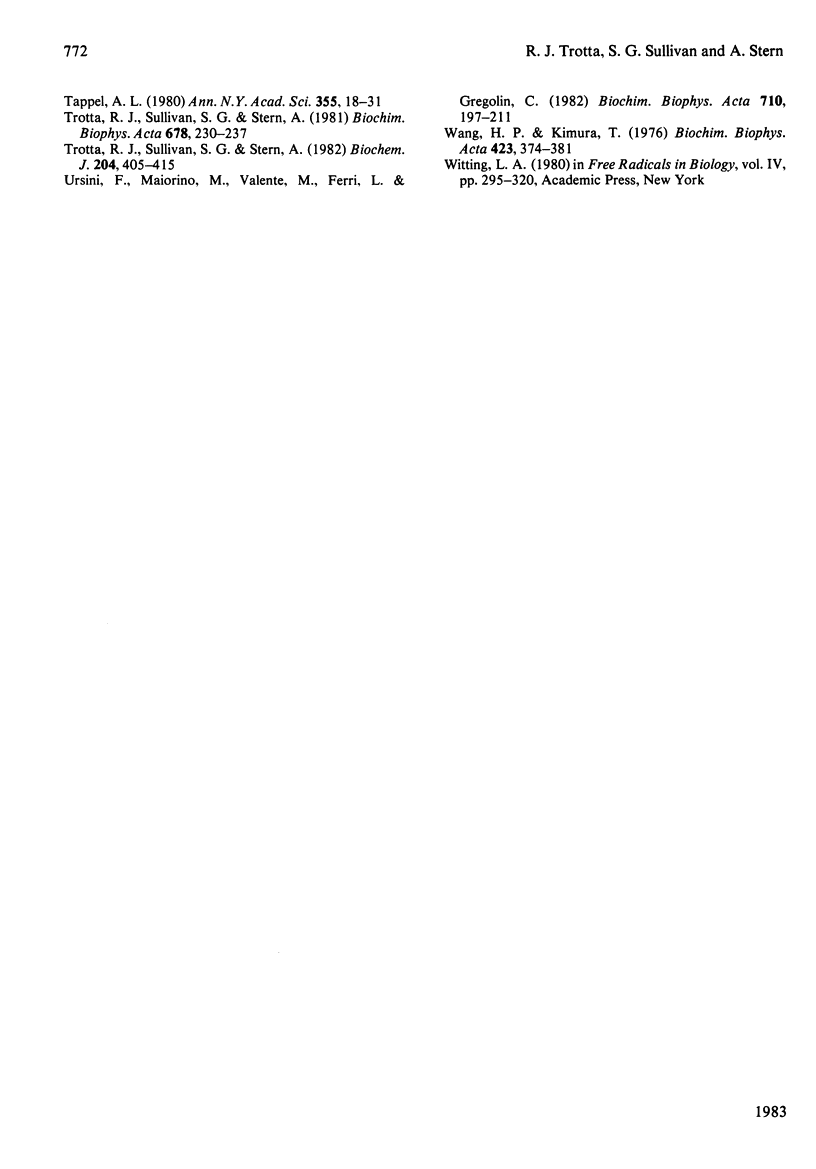
Selected References
These references are in PubMed. This may not be the complete list of references from this article.
- Ames B. N., Cathcart R., Schwiers E., Hochstein P. Uric acid provides an antioxidant defense in humans against oxidant- and radical-caused aging and cancer: a hypothesis. Proc Natl Acad Sci U S A. 1981 Nov;78(11):6858–6862. doi: 10.1073/pnas.78.11.6858. [DOI] [PMC free article] [PubMed] [Google Scholar]
- Barber A. A., Bernheim F. Lipid peroxidation: its measurement, occurrence, and significance in animal tissues. Adv Gerontol Res. 1967;2:355–403. [PubMed] [Google Scholar]
- Bartosz G., Leyko W. Radioprotection of bovine erythrocytes to haemolysis. Int J Radiat Biol Relat Stud Phys Chem Med. 1981 Jan;39(1):39–46. doi: 10.1080/09553008114550051. [DOI] [PubMed] [Google Scholar]
- Benatti U., Morelli A., Meloni T., Sparatore B., Salamino F., Michetti M., Melloni E., Pontremoli S., de Flora A. Comparative patterns of 'in vitro' oxidative hemolysis of normal and glucose 6-phosphate dehydrogenase (G6PD)-deficient erythrocytes. FEBS Lett. 1981 Jun 15;128(2):225–229. doi: 10.1016/0014-5793(81)80086-5. [DOI] [PubMed] [Google Scholar]
- Burk R. F., Lawrence R. A., Lane J. M. Liver necrosis and lipid peroxidation in the rat as the result of paraquat and diquat administration. Effect of selenium deficiency. J Clin Invest. 1980 May;65(5):1024–1031. doi: 10.1172/JCI109754. [DOI] [PMC free article] [PubMed] [Google Scholar]
- Burton G. W., Ingold K. U., Thompson K. E. An improved procedure for the isolation of ghost membranes from human red blood cells. Lipids. 1981 Dec;16(12):946–946. doi: 10.1007/BF02535005. [DOI] [PubMed] [Google Scholar]
- COHEN G., HOCHSTEIN P. GLUTATHIONE PEROXIDASE: THE PRIMARY AGENT FOR THE ELIMINATION OF HYDROGEN PEROXIDE IN ERYTHROCYTES. Biochemistry. 1963 Nov-Dec;2:1420–1428. doi: 10.1021/bi00906a038. [DOI] [PubMed] [Google Scholar]
- Christophersen B. O. Reduction of linolenic acid hydroperoxide by a glutathione peroxidase. Biochim Biophys Acta. 1969 Apr 29;176(3):463–470. doi: 10.1016/0005-2760(69)90213-6. [DOI] [PubMed] [Google Scholar]
- DODGE J. T., MITCHELL C., HANAHAN D. J. The preparation and chemical characteristics of hemoglobin-free ghosts of human erythrocytes. Arch Biochem Biophys. 1963 Jan;100:119–130. doi: 10.1016/0003-9861(63)90042-0. [DOI] [PubMed] [Google Scholar]
- GEORGE P. The chemical nature of the second hydrogen peroxide compound formed by cytochrome c peroxidase and horseradish peroxidase. I. Titration with reducing agents. Biochem J. 1953 May;54(2):267–276. doi: 10.1042/bj0540267. [DOI] [PMC free article] [PubMed] [Google Scholar]
- Gibson D. D., Hornbrook K. R., McCay P. B. Glutathione-dependent inhibition of lipid peroxidation by a soluble, heat-labile factor in animal tissues. Biochim Biophys Acta. 1980 Dec 5;620(3):572–582. doi: 10.1016/0005-2760(80)90149-6. [DOI] [PubMed] [Google Scholar]
- Goldberg B., Stern A. The mechanism of oxidative hemolysis produced by phenylhydrazine. Mol Pharmacol. 1977 Sep;13(5):832–839. [PubMed] [Google Scholar]
- Goldstein B. D., Rozen M. G., Kunis R. L. Role of red cell membrane lipid peroxidation in hemolysis due to phenylhydrazine. Biochem Pharmacol. 1980 May 15;29(10):1355–1359. doi: 10.1016/0006-2952(80)90430-x. [DOI] [PubMed] [Google Scholar]
- Griffin B. W., Ting P. L. Spin trapping evidence for free radical oxidants of aminopyrine in the metmyoglobin-cumene hydroperoxide system. FEBS Lett. 1978 May 15;89(2):196–200. doi: 10.1016/0014-5793(78)80216-6. [DOI] [PubMed] [Google Scholar]
- HOWELL R. R., WYNGAARDEN J. B. On the mechanism of peroxidation of uric acids by hemoproteins. J Biol Chem. 1960 Dec;235:3544–3550. [PubMed] [Google Scholar]
- Kunimoto M., Inoue K., Nojima S. Effect of ferrous ion and ascorbate-induced lipid peroxidation on liposomal membranes. Biochim Biophys Acta. 1981 Aug 6;646(1):169–178. doi: 10.1016/0005-2736(81)90284-4. [DOI] [PubMed] [Google Scholar]
- Logani M. K., Davies R. E. Lipid oxidation: biologic effects and antioxidants--a review. Lipids. 1980 Jun;15(6):485–495. doi: 10.1007/BF02534079. [DOI] [PubMed] [Google Scholar]
- McCray P. B., Gibson D. D., Fong K. L., Hornbrook K. R. Effect of glutathione peroxidase activity on lipid peroxidation in biological membranes. Biochim Biophys Acta. 1976 Jun 22;431(3):459–468. [PubMed] [Google Scholar]
- O'Brien P. J. Intracellular mechanisms for the decomposition of a lipid peroxide. I. Decomposition of a lipid peroxide by metal ions, heme compounds, and nucleophiles. Can J Biochem. 1969 May;47(5):485–492. doi: 10.1139/o69-076. [DOI] [PubMed] [Google Scholar]
- Oshino N., Chance B. Properties of glutathione release observed during reduction of organic hydroperoxide, demethylation of aminopyrine and oxidation of some substances in perfused rat liver, and their implications for the physiological function of catalase. Biochem J. 1977 Mar 15;162(3):509–525. doi: 10.1042/bj1620509. [DOI] [PMC free article] [PubMed] [Google Scholar]
- Stocks J., Dormandy T. L. The autoxidation of human red cell lipids induced by hydrogen peroxide. Br J Haematol. 1971 Jan;20(1):95–111. doi: 10.1111/j.1365-2141.1971.tb00790.x. [DOI] [PubMed] [Google Scholar]
- Stults F. H., Forstrom J. W., Chiu D. T., Tappel A. L. Rat liver glutathione peroxidase: purification and study of multiple forms. Arch Biochem Biophys. 1977 Oct;183(2):490–497. doi: 10.1016/0003-9861(77)90384-8. [DOI] [PubMed] [Google Scholar]
- TAPPEL A. L. The mechanism of the oxidation of unsaturated fatty acids catalyzed by hematin compounds. Arch Biochem Biophys. 1953 Jun;44(2):378–395. doi: 10.1016/0003-9861(53)90056-3. [DOI] [PubMed] [Google Scholar]
- Tappel A. L. Vitamin E and selenium protection from in vivo lipid peroxidation. Ann N Y Acad Sci. 1980;355:18–31. doi: 10.1111/j.1749-6632.1980.tb21324.x. [DOI] [PubMed] [Google Scholar]
- Trotta R. J., Sullivan S. G., Stern A. Lipid peroxidation and haemoglobin degradation in red blood cells exposed to t-butyl hydroperoxide. Effects of the hexose monophosphate shunt as mediated by glutathione and ascorbate. Biochem J. 1982 May 15;204(2):405–415. doi: 10.1042/bj2040405. [DOI] [PMC free article] [PubMed] [Google Scholar]
- Trotta R. J., Sullivan S. G., Stern A. Lipid peroxidation and hemoglobin degradation in red blood cells exposed to t-butyl hydroperoxide. Dependence on glucose metabolism and hemoglobin status. Biochim Biophys Acta. 1981 Dec 4;678(2):230–237. doi: 10.1016/0304-4165(81)90211-7. [DOI] [PubMed] [Google Scholar]
- Ursini F., Maiorino M., Valente M., Ferri L., Gregolin C. Purification from pig liver of a protein which protects liposomes and biomembranes from peroxidative degradation and exhibits glutathione peroxidase activity on phosphatidylcholine hydroperoxides. Biochim Biophys Acta. 1982 Feb 15;710(2):197–211. doi: 10.1016/0005-2760(82)90150-3. [DOI] [PubMed] [Google Scholar]
- Wang H. P., Kimura T. Ferrous ion-mediated cytochrome P-450 degradation and lipid peroxidation in adrenal cortex mitochondria. Biochim Biophys Acta. 1976 Mar 12;423(3):374–381. doi: 10.1016/0005-2728(76)90194-8. [DOI] [PubMed] [Google Scholar]