Abstract
1. Isolated, fast-twitch, low-oxidative muscle fibres from the iliofibularis muscle of Xenopus laevis were fatigued by intermittent tetanic stimulation at 20 degrees C in different Ringer solutions and the amount of lactate released was determined. 2. The rate of lactate efflux was constant during 10 min of intermittent stimulation while lactate in the fibres accumulated, and lactate efflux was not hampered by an unstirred layer surrounding the isolated muscle fibre. 3. The rate of lactate efflux at extracellular pH 7.2 was the same as that at pH 7.8, but depended on the type of buffer used; the highest efflux rate (mean +/- S.E.M., 7.4 +/- 2.2 mumol min-1 (g dry weight)-1, n = 8) was observed in bicarbonate-buffered Ringer solution. This rate was about 2.5 times higher than the rate in phosphate-buffered Ringer solution (2.9 +/- 1.3 mumol min-1 (g dry weight)-1, n = 8), indicating that lactate-bicarbonate exchange is the most important route for lactate extrusion in vivo. 4. The highest rate of lactate efflux corresponds to a rate of glycolytic ATP production which is only about 30% of the oxidative rate of ATP production (calculated from the maximum rate of oxygen consumption determined previously). 5. In the presence of 5 mM alpha-cyano-4-hydroxycinnamate (CHC) the lowest lactate efflux rate (1.5 +/- 0.6 mumol min-1 (g dry weight)-1, n = 16) was found. This rate was independent of the composition of the Ringer solution. Assuming that 5 mM CHC completely inhibits lactate transporters in the sarcolemma, the rate of lactate efflux in the presence of 5 mM CHC can be explained by passive diffusion, but only if most lactate is extruded via the T-tubules.
Full text
PDF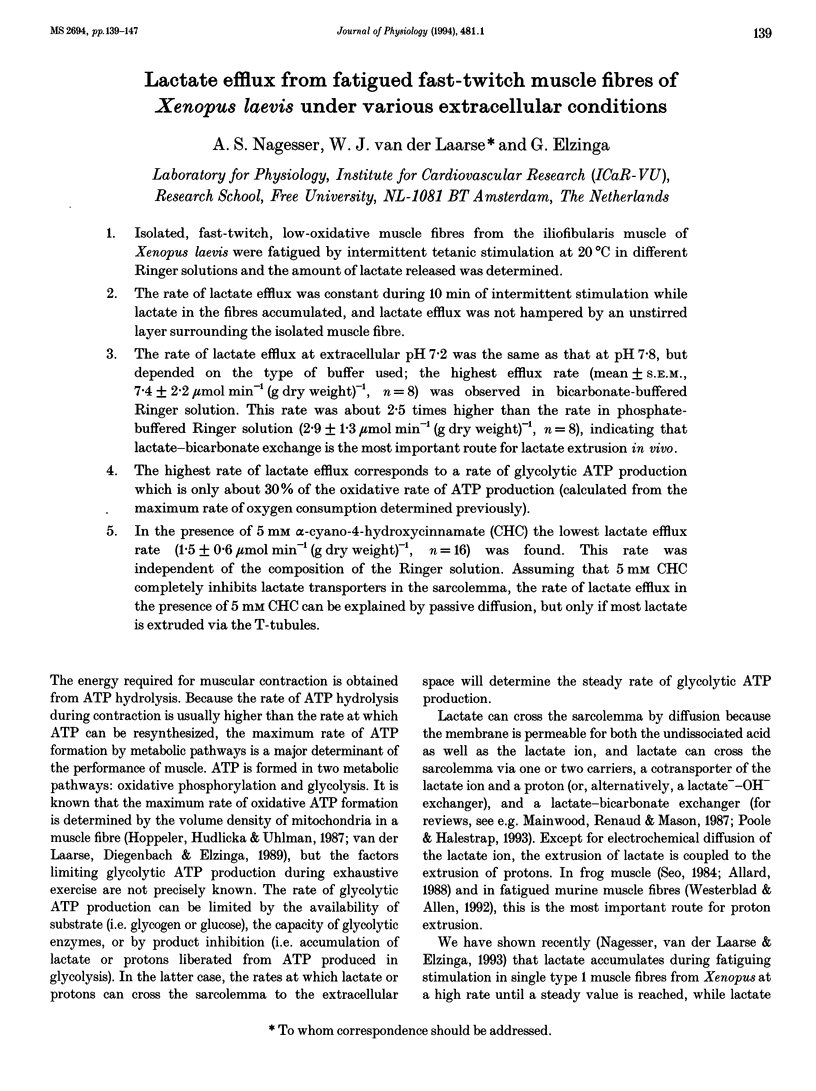
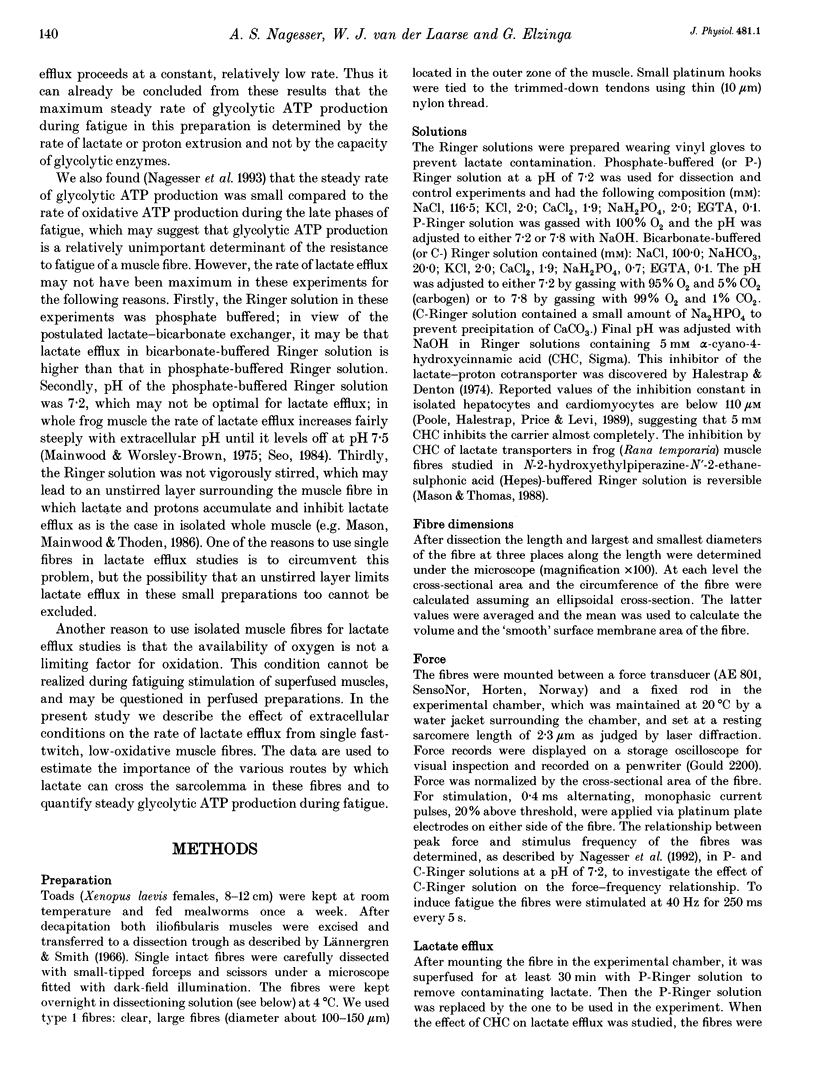
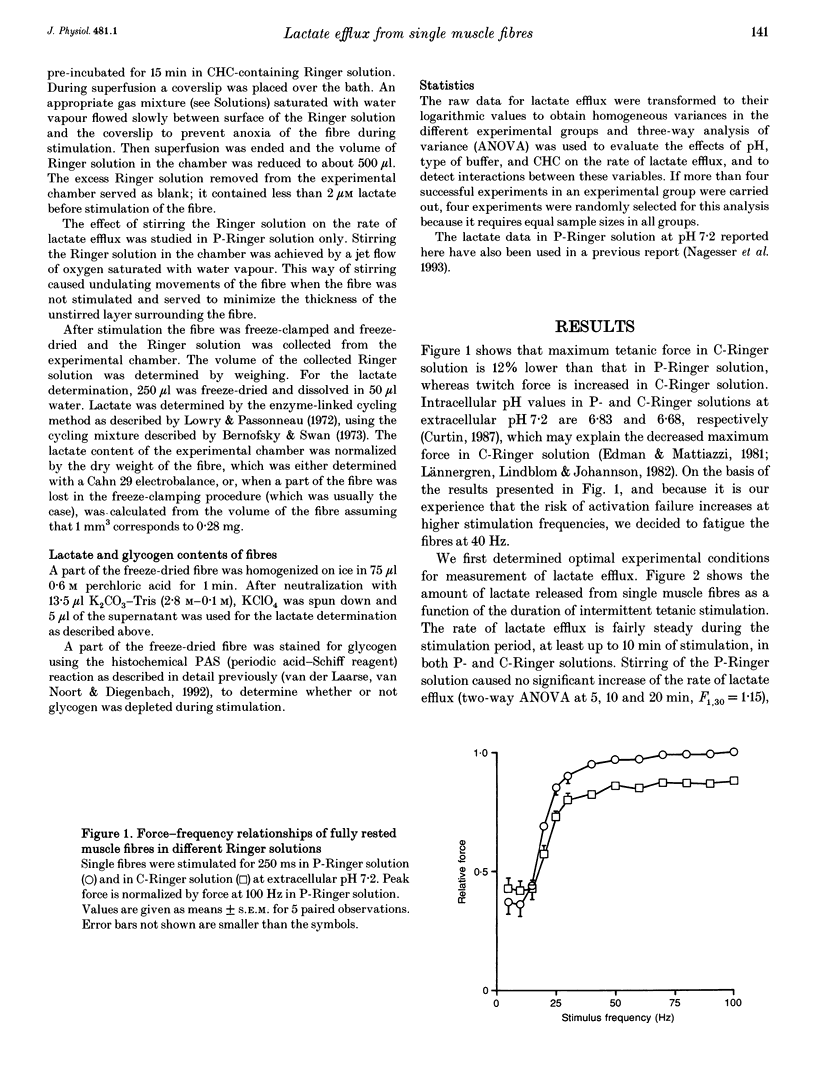
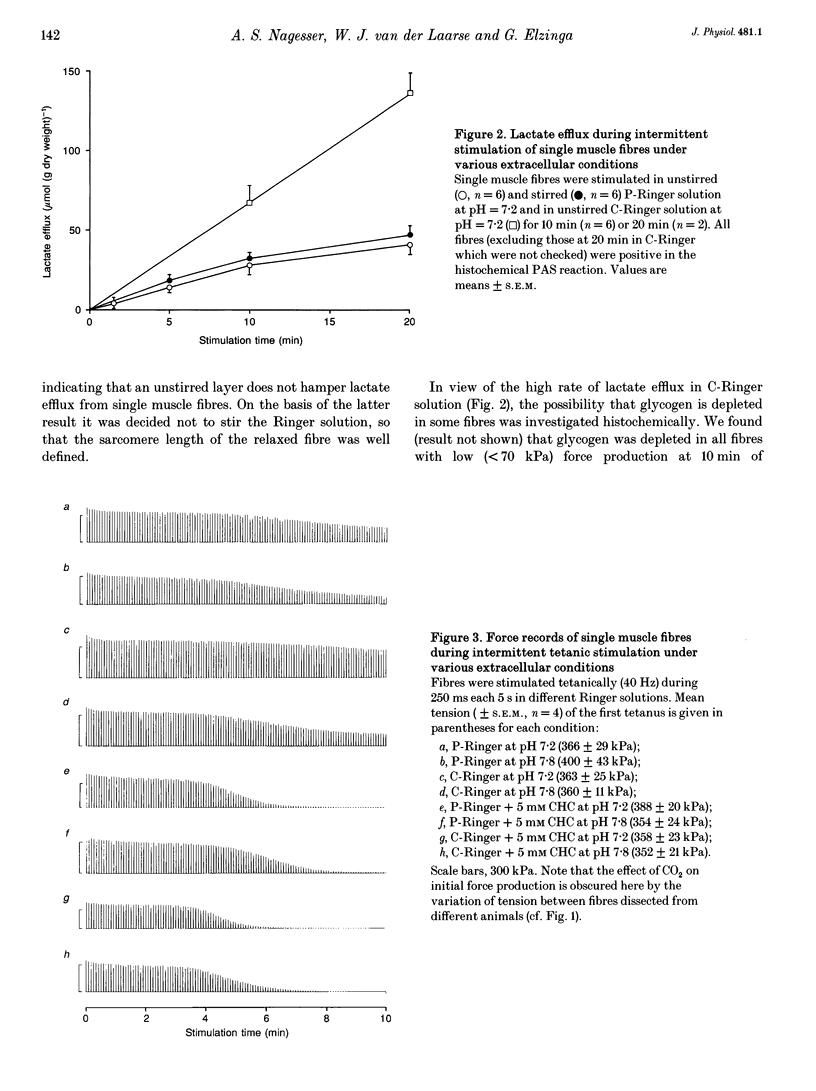
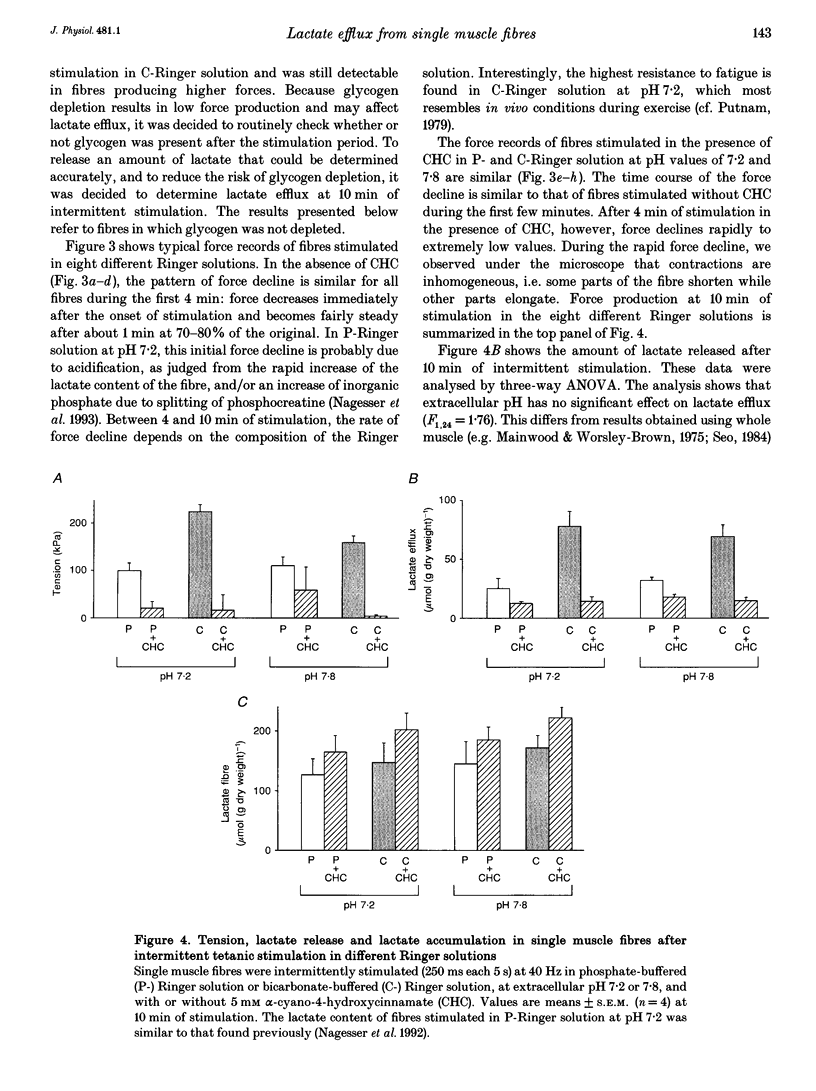
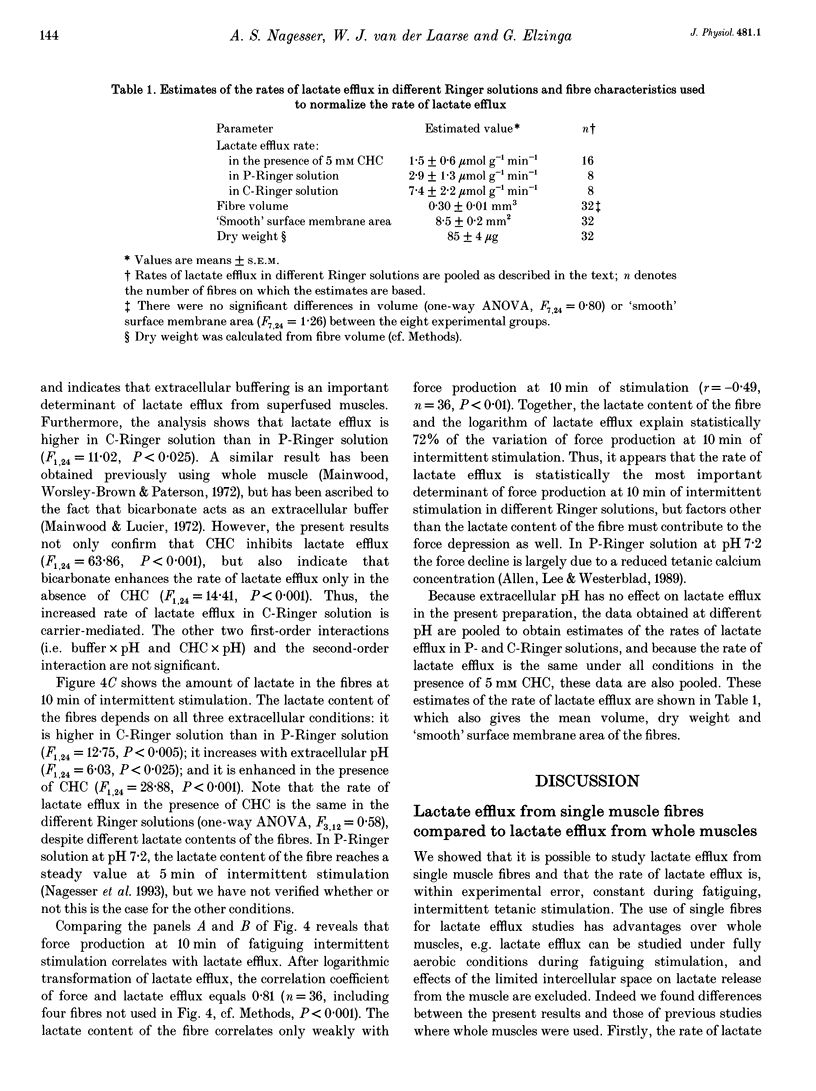
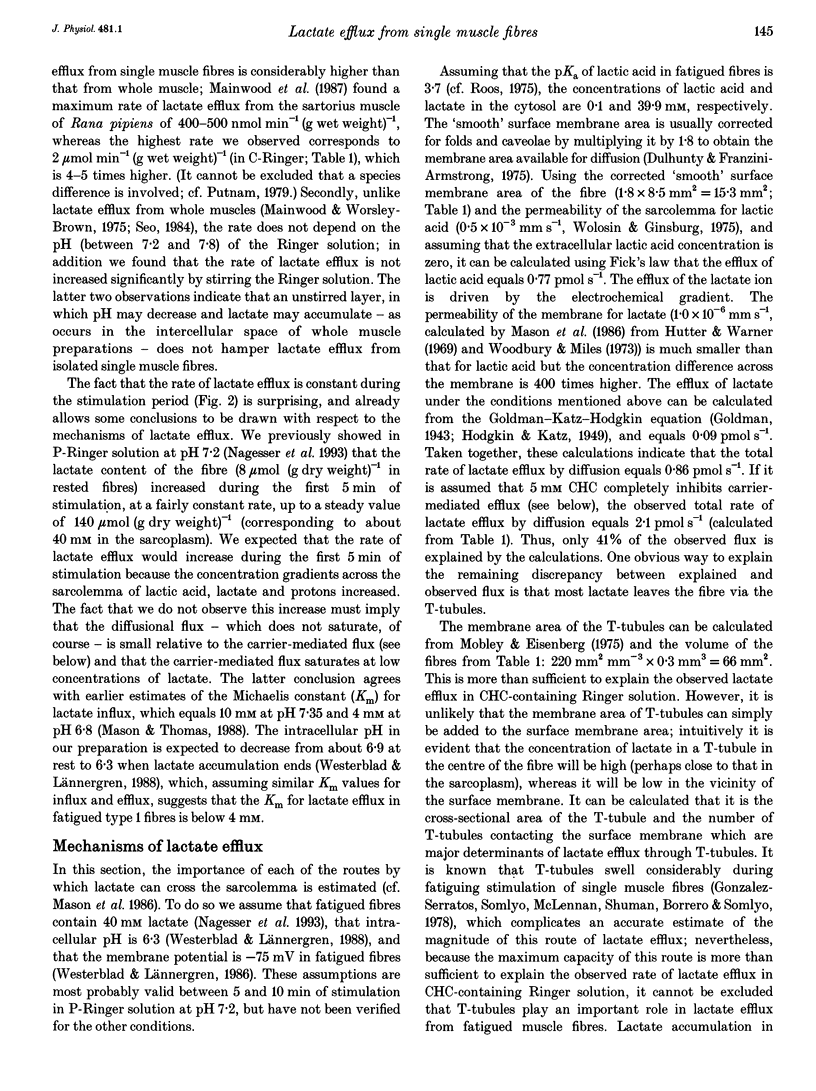
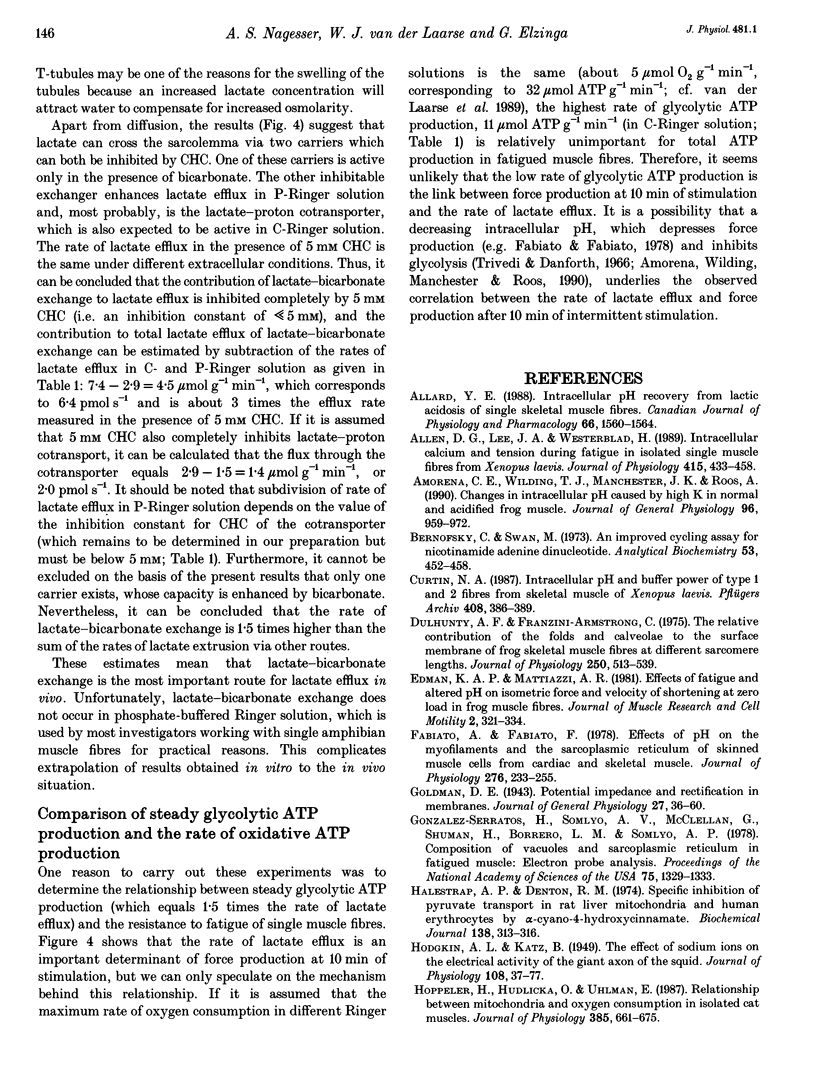
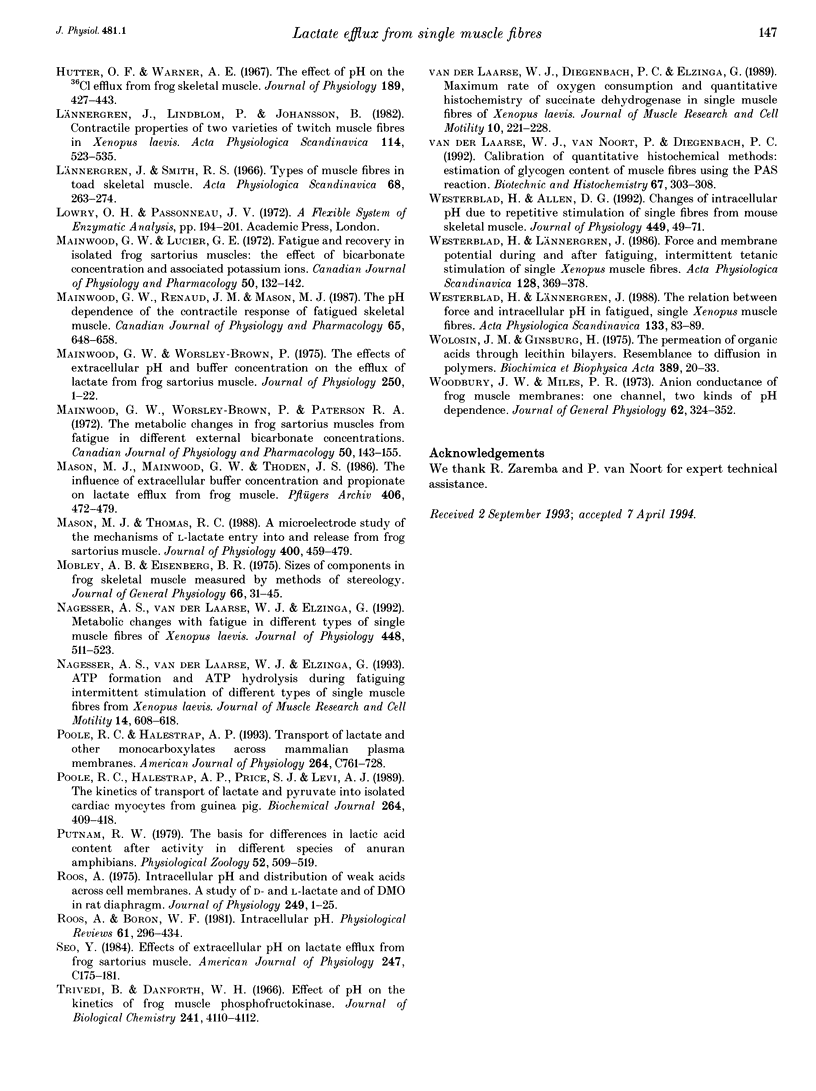
Images in this article
Selected References
These references are in PubMed. This may not be the complete list of references from this article.
- Allard Y. E. Intracellular pH recovery from lactic acidosis of single skeletal muscle fibers. Can J Physiol Pharmacol. 1988 Dec;66(12):1560–1564. doi: 10.1139/y88-255. [DOI] [PubMed] [Google Scholar]
- Allen D. G., Lee J. A., Westerblad H. Intracellular calcium and tension during fatigue in isolated single muscle fibres from Xenopus laevis. J Physiol. 1989 Aug;415:433–458. doi: 10.1113/jphysiol.1989.sp017730. [DOI] [PMC free article] [PubMed] [Google Scholar]
- Amorena C. E., Wilding T. J., Manchester J. K., Roos A. Changes in intracellular pH caused by high K in normal and acidified frog muscle. Relation to metabolic changes. J Gen Physiol. 1990 Nov;96(5):959–972. doi: 10.1085/jgp.96.5.959. [DOI] [PMC free article] [PubMed] [Google Scholar]
- Bernofsky C., Swan M. An improved cycling assay for nicotinamide adenine dinucleotide. Anal Biochem. 1973 Jun;53(2):452–458. doi: 10.1016/0003-2697(73)90094-8. [DOI] [PubMed] [Google Scholar]
- Curtin N. A. Intracellular pH and buffer power of type 1 and 2 fibres from skeletal muscle of Xenopus laevis. Pflugers Arch. 1987 Apr;408(4):386–389. doi: 10.1007/BF00581133. [DOI] [PubMed] [Google Scholar]
- Dulhunty A. F., Franzini-Armstrong C. The relative contributions of the folds and caveolae to the surface membrane of frog skeletal muscle fibres at different sarcomere lengths. J Physiol. 1975 Sep;250(3):513–539. doi: 10.1113/jphysiol.1975.sp011068. [DOI] [PMC free article] [PubMed] [Google Scholar]
- Edman K. A., Mattiazzi A. R. Effects of fatigue and altered pH on isometric force and velocity of shortening at zero load in frog muscle fibres. J Muscle Res Cell Motil. 1981 Sep;2(3):321–334. doi: 10.1007/BF00713270. [DOI] [PubMed] [Google Scholar]
- Fabiato A., Fabiato F. Effects of pH on the myofilaments and the sarcoplasmic reticulum of skinned cells from cardiace and skeletal muscles. J Physiol. 1978 Mar;276:233–255. doi: 10.1113/jphysiol.1978.sp012231. [DOI] [PMC free article] [PubMed] [Google Scholar]
- Goldman D. E. POTENTIAL, IMPEDANCE, AND RECTIFICATION IN MEMBRANES. J Gen Physiol. 1943 Sep 20;27(1):37–60. doi: 10.1085/jgp.27.1.37. [DOI] [PMC free article] [PubMed] [Google Scholar]
- Gonzalez-Serratos H., Somlyo A. V., McClellan G., Shuman H., Borrero L. M., Somlyo A. P. Composition of vacuoles and sarcoplasmic reticulum in fatigued muscle: electron probe analysis. Proc Natl Acad Sci U S A. 1978 Mar;75(3):1329–1333. doi: 10.1073/pnas.75.3.1329. [DOI] [PMC free article] [PubMed] [Google Scholar]
- HODGKIN A. L., KATZ B. The effect of sodium ions on the electrical activity of giant axon of the squid. J Physiol. 1949 Mar 1;108(1):37–77. doi: 10.1113/jphysiol.1949.sp004310. [DOI] [PMC free article] [PubMed] [Google Scholar]
- Halestrap A. P., Denton R. M. Specific inhibition of pyruvate transport in rat liver mitochondria and human erythrocytes by alpha-cyano-4-hydroxycinnamate. Biochem J. 1974 Feb;138(2):313–316. doi: 10.1042/bj1380313. [DOI] [PMC free article] [PubMed] [Google Scholar]
- Hoppeler H., Hudlicka O., Uhlmann E. Relationship between mitochondria and oxygen consumption in isolated cat muscles. J Physiol. 1987 Apr;385:661–675. doi: 10.1113/jphysiol.1987.sp016513. [DOI] [PMC free article] [PubMed] [Google Scholar]
- Hutter O. F., Warner A. E. The effect of pH on the 36-Cl efflux from frog skeletal muscle. J Physiol. 1967 Apr;189(3):427–443. doi: 10.1113/jphysiol.1967.sp008177. [DOI] [PMC free article] [PubMed] [Google Scholar]
- Lännergren J., Lindblom P., Johansson B. Contractile properties of two varieties of twitch muscle fibres in Xenopus laevis. Acta Physiol Scand. 1982 Apr;114(4):523–535. doi: 10.1111/j.1748-1716.1982.tb07020.x. [DOI] [PubMed] [Google Scholar]
- Mainwood G. W., Lucier G. E. Fatigue and recovery in isolated frog sartorius muscles: the effects of bicarbonate concentration and associated potassium loss. Can J Physiol Pharmacol. 1972 Feb;50(2):132–142. doi: 10.1139/y72-020. [DOI] [PubMed] [Google Scholar]
- Mainwood G. W., Renaud J. M., Mason M. J. The pH dependence of the contractile response of fatigued skeletal muscle. Can J Physiol Pharmacol. 1987 Apr;65(4):648–658. doi: 10.1139/y87-108. [DOI] [PubMed] [Google Scholar]
- Mainwood G. W., Worsley-Brown P., Paterson R. A. The metabolic changes in frog sartorius muscles during recovery from fatigue at different external bicarbonate concentrations. Can J Physiol Pharmacol. 1972 Feb;50(2):143–155. doi: 10.1139/y72-021. [DOI] [PubMed] [Google Scholar]
- Mainwood G. W., Worsley-Brown P. The effects of extracellular pH and buffer concentration on the efflux of lactate from frog sartorius muscle. J Physiol. 1975 Aug;250(1):1–22. doi: 10.1113/jphysiol.1975.sp011040. [DOI] [PMC free article] [PubMed] [Google Scholar]
- Mason M. J., Mainwood G. W., Thoden J. S. The influence of extracellular buffer concentration and propionate on lactate efflux from frog muscle. Pflugers Arch. 1986 May;406(5):472–479. doi: 10.1007/BF00583369. [DOI] [PubMed] [Google Scholar]
- Mason M. J., Thomas R. C. A microelectrode study of the mechanisms of L-lactate entry into and release from frog sartorius muscle. J Physiol. 1988 Jun;400:459–479. doi: 10.1113/jphysiol.1988.sp017132. [DOI] [PMC free article] [PubMed] [Google Scholar]
- Mobley B. A., Eisenberg B. R. Sizes of components in frog skeletal muscle measured by methods of stereology. J Gen Physiol. 1975 Jul;66(1):31–45. doi: 10.1085/jgp.66.1.31. [DOI] [PMC free article] [PubMed] [Google Scholar]
- Nagesser A. S., Van der Laarse W. J., Elzinga G. ATP formation and ATP hydrolysis during fatiguing, intermittent stimulation of different types of single muscle fibres from Xenopus laevis. J Muscle Res Cell Motil. 1993 Dec;14(6):608–618. doi: 10.1007/BF00141558. [DOI] [PubMed] [Google Scholar]
- Nagesser A. S., van der Laarse W. J., Elzinga G. Metabolic changes with fatigue in different types of single muscle fibres of Xenopus laevis. J Physiol. 1992 Mar;448:511–523. doi: 10.1113/jphysiol.1992.sp019054. [DOI] [PMC free article] [PubMed] [Google Scholar]
- Poole R. C., Halestrap A. P., Price S. J., Levi A. J. The kinetics of transport of lactate and pyruvate into isolated cardiac myocytes from guinea pig. Kinetic evidence for the presence of a carrier distinct from that in erythrocytes and hepatocytes. Biochem J. 1989 Dec 1;264(2):409–418. doi: 10.1042/bj2640409. [DOI] [PMC free article] [PubMed] [Google Scholar]
- Poole R. C., Halestrap A. P. Transport of lactate and other monocarboxylates across mammalian plasma membranes. Am J Physiol. 1993 Apr;264(4 Pt 1):C761–C782. doi: 10.1152/ajpcell.1993.264.4.C761. [DOI] [PubMed] [Google Scholar]
- Roos A., Boron W. F. Intracellular pH. Physiol Rev. 1981 Apr;61(2):296–434. doi: 10.1152/physrev.1981.61.2.296. [DOI] [PubMed] [Google Scholar]
- Roos A. Intracellular pH and distribution of weak acids across cell membranes. A study of D- and L-lactate and of DMO in rat diaphragm. J Physiol. 1975 Jul;249(1):1–25. doi: 10.1113/jphysiol.1975.sp011000. [DOI] [PMC free article] [PubMed] [Google Scholar]
- Seo Y. Effects of extracellular pH on lactate efflux from frog sartorius muscle. Am J Physiol. 1984 Sep;247(3 Pt 1):C175–C181. doi: 10.1152/ajpcell.1984.247.3.C175. [DOI] [PubMed] [Google Scholar]
- Trivedi B., Danforth W. H. Effect of pH on the kinetics of frog muscle phosphofructokinase. J Biol Chem. 1966 Sep 10;241(17):4110–4112. [PubMed] [Google Scholar]
- Westerblad H., Allen D. G. Changes of intracellular pH due to repetitive stimulation of single fibres from mouse skeletal muscle. J Physiol. 1992 Apr;449:49–71. doi: 10.1113/jphysiol.1992.sp019074. [DOI] [PMC free article] [PubMed] [Google Scholar]
- Westerblad H., Lännergren J. Force and membrane potential during and after fatiguing, intermittent tetanic stimulation of single Xenopus muscle fibres. Acta Physiol Scand. 1986 Nov;128(3):369–378. doi: 10.1111/j.1748-1716.1986.tb07990.x. [DOI] [PubMed] [Google Scholar]
- Westerblad H., Lännergren J. The relation between force and intracellular pH in fatigued, single Xenopus muscle fibres. Acta Physiol Scand. 1988 May;133(1):83–89. doi: 10.1111/j.1748-1716.1988.tb08383.x. [DOI] [PubMed] [Google Scholar]
- Wolosin J. M., Ginsburg H. The permeation of organic acids through lecithin bilayers. Resemblance to diffusion in polymers. Biochim Biophys Acta. 1975 Apr 21;389(1):20–33. doi: 10.1016/0005-2736(75)90382-x. [DOI] [PubMed] [Google Scholar]
- Woodbury J. W., Miles P. R. Anion conductance of frog muscle membranes: one channel, two kinds of pH dependence. J Gen Physiol. 1973 Sep;62(3):324–353. doi: 10.1085/jgp.62.3.324. [DOI] [PMC free article] [PubMed] [Google Scholar]
- van der Laarse W. J., Diegenbach P. C., Elzinga G. Maximum rate of oxygen consumption and quantitative histochemistry of succinate dehydrogenase in single muscle fibres of Xenopus laevis. J Muscle Res Cell Motil. 1989 Jun;10(3):221–228. doi: 10.1007/BF01739812. [DOI] [PubMed] [Google Scholar]
- van der Laarse W. J., van Noort P., Diegenbach P. C. Calibration of quantitative histochemical methods: estimation of glycogen content of muscle fibers using the PAS reaction. Biotech Histochem. 1992 Sep;67(5):303–308. doi: 10.3109/10520299209110039. [DOI] [PubMed] [Google Scholar]