Abstract
1. Electrophysiological measurements of Ca2+ influx using patch clamp methodology were combined with fluorescent monitoring of the free intracellular calcium concentration ([Ca2+]i) to determine mechanisms of Ca2+ regulation in isolated nerve endings from the rat neurohypophysis. 2. Application of step depolarizations under voltage clamp resulted in voltage-dependent calcium influx (ICa) and increase in the [Ca2+]i. The increase in [Ca2+]i was proportional to the time-integrated ICa for low calcium loads but approached an asymptote of [Ca2+]i at large Ca2+ loads. These data indicate the presence of two distinct rapid Ca2+ buffering mechanisms. 3. Dialysis of fura-2, which competes for Ca2+ binding with the endogenous Ca2+ buffers, reduced the amplitude and increased the duration of the step depolarization-evoked Ca2+ transients. More than 99% of Ca2+ influx at low Ca2+ loads is immediately buffered by this endogenous buffer component, which probably consists of intracellular Ca2+ binding proteins. 4. The capacity of the endogenous buffer for binding Ca2+ remained stable during 300 s of dialysis of the nerve endings. These properties indicated that this Ca2+ buffer component was either immobile or of high molecular weight and slowly diffusible. 5. In the presence of large Ca2+ loads a second distinct Ca2+ buffer mechanism was resolved which limited increases in [Ca2+]i to approximately 600 nM. This Ca2+ buffer exhibited high capacity but low affinity for Ca2+ and its presence resulted in a loss of proportionality between the integrated ICa and the increase in [Ca2+]i. This buffering mechanism was sensitive to the mitochondrial Ca2+ uptake inhibitor Ruthenium Red. 6. Basal [Ca2+]i, depolarization-induced changes in [Ca2+]i and recovery of [Ca2+]i to resting levels following an induced increase in [Ca2+]i were unaffected by thapsigargin and cyclopiazonic acid, specific inhibitors of intracellular Ca(2+)-ATPases. Caffeine and ryanodine were also without effect on Ca2+ regulation. 7. Evoked increases in [Ca2+]i, as well as rates of recovery from a Ca2+ load, were unaffected by the extracellular [Na+], suggesting a minimal role for Na(+)-Ca2+ exchange in Ca2+ regulation in these nerve endings. 8. Application of repetitive step depolarizations for a constant period of stimulation resulted in a proportional frequency (up to 40 Hz)-dependent increase in [Ca2+]i. On the other hand, for a constant number of stimuli a reduction in the [Ca2+]i. On the other hand, for a constant number of stimuli a reduction in the [Ca2+]i increase per impulse was observed at higher frequencies.(ABSTRACT TRUNCATED AT 250 WORDS)
Full text
PDF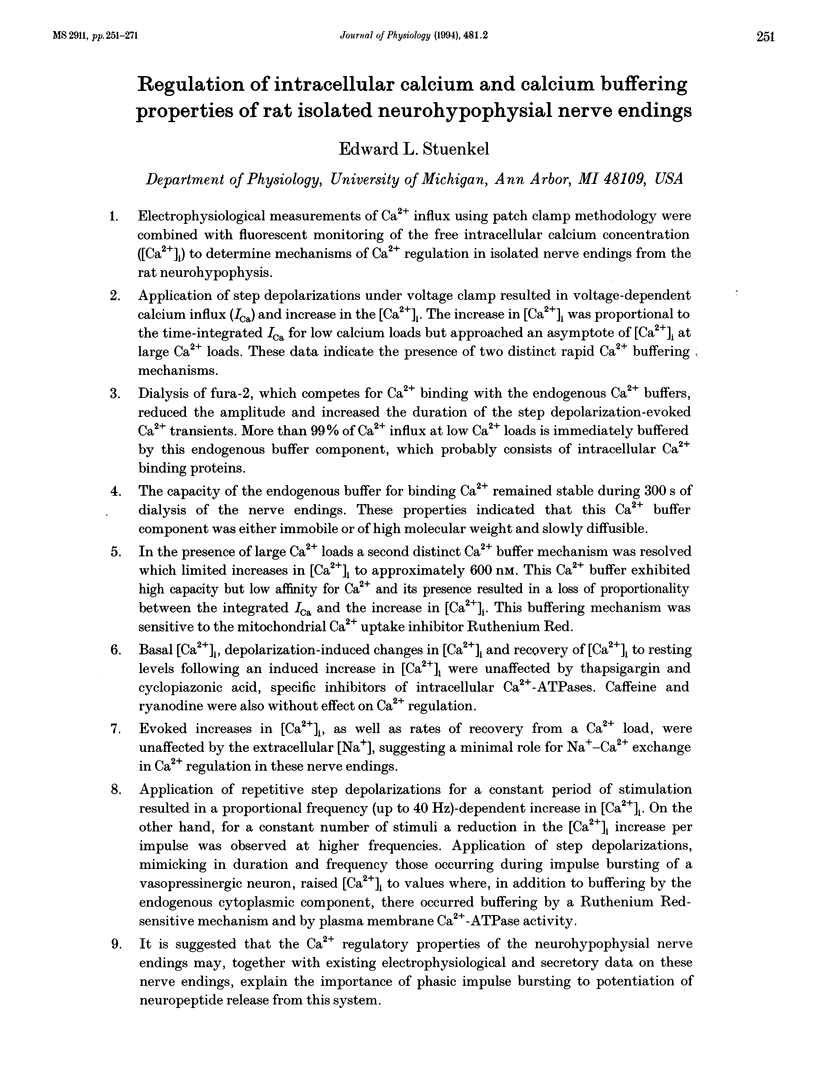
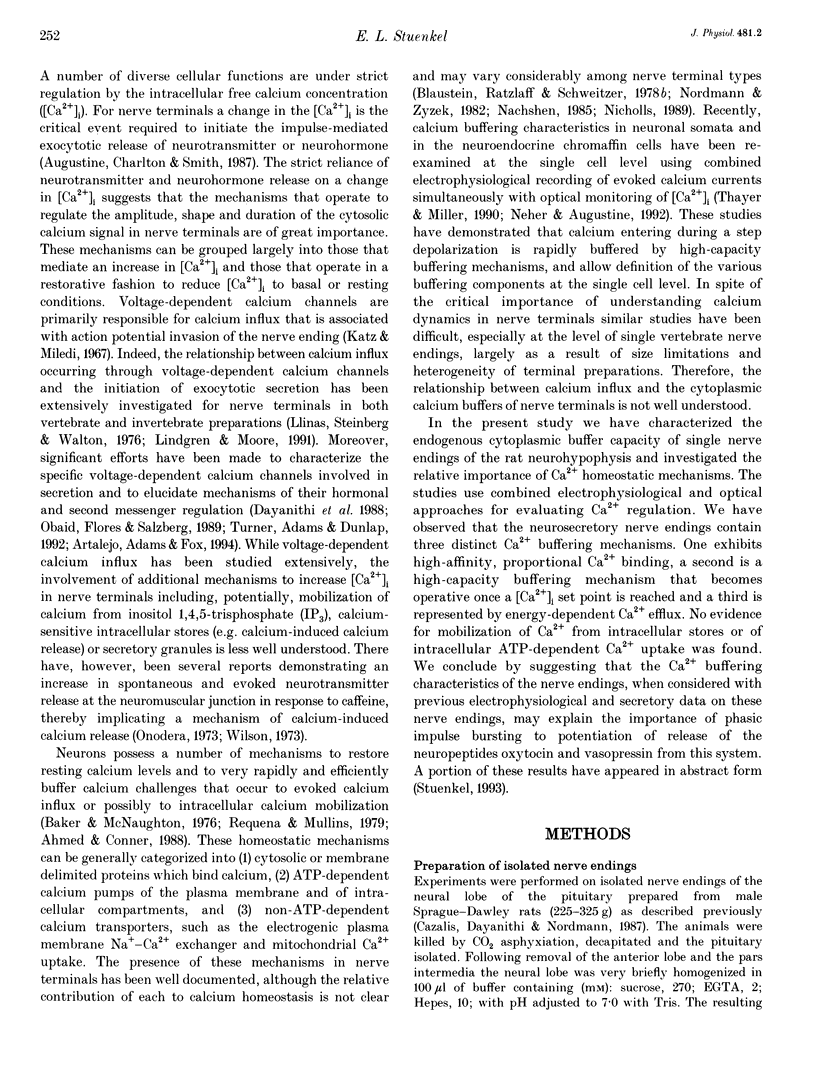
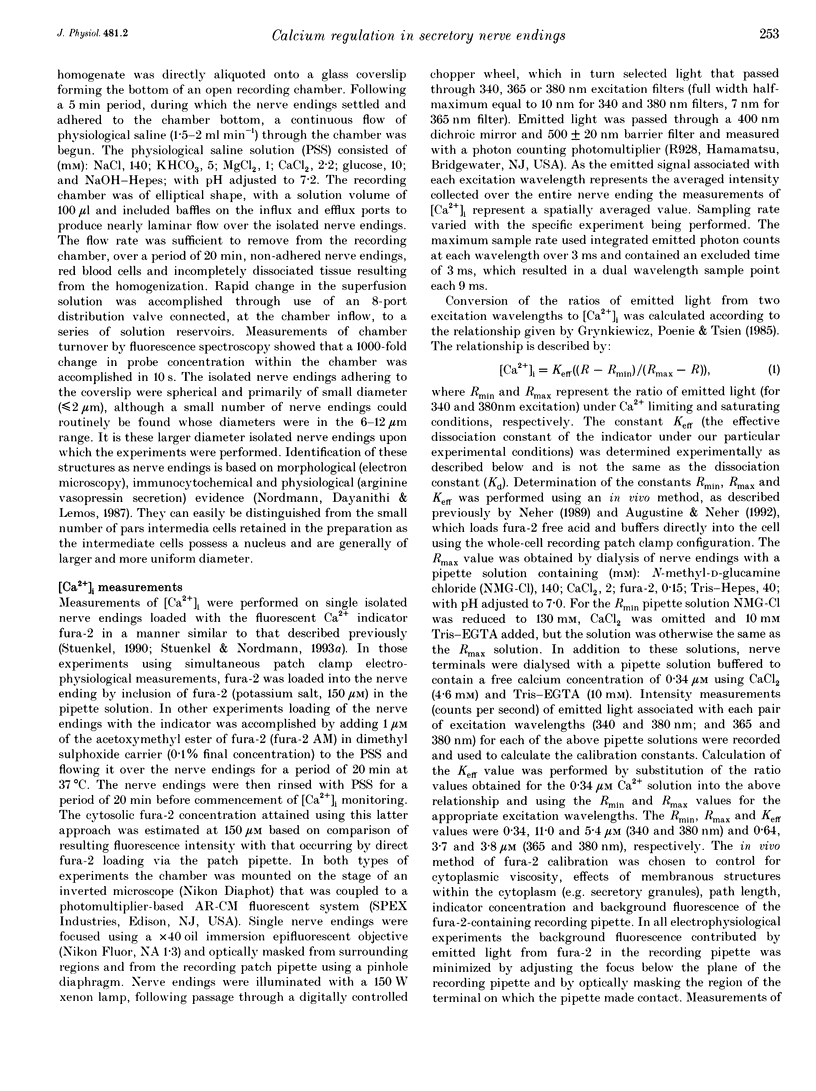
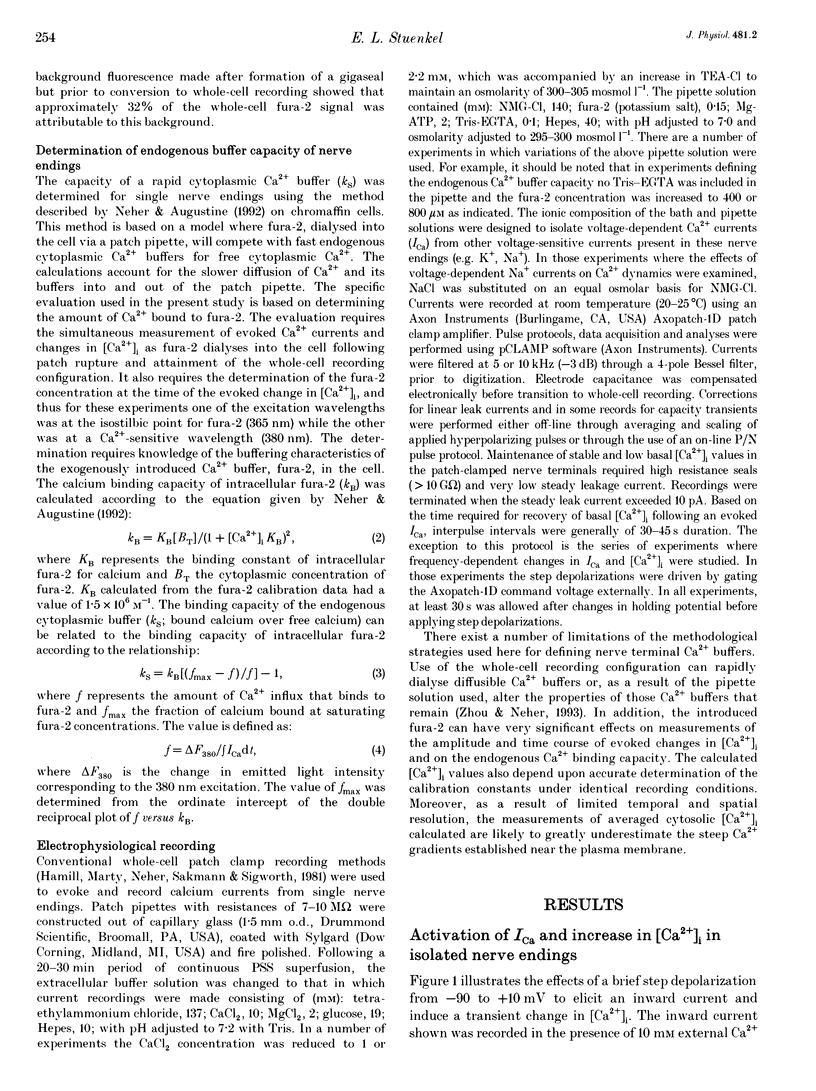
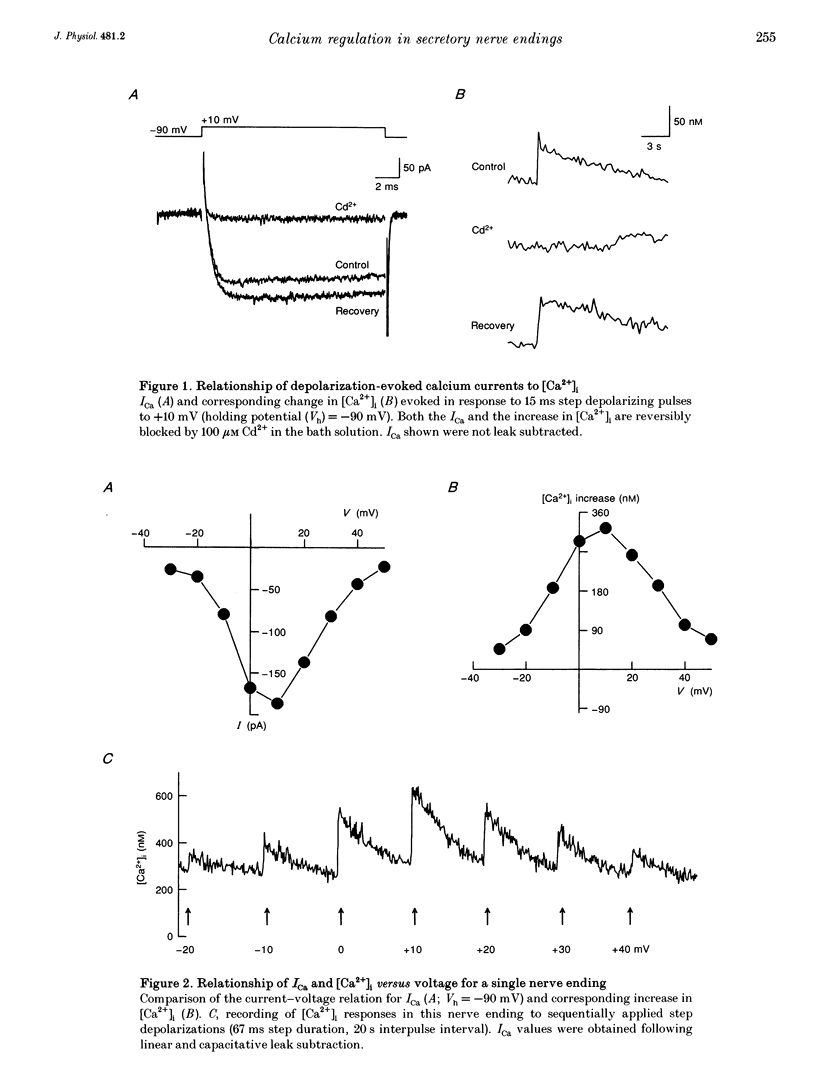
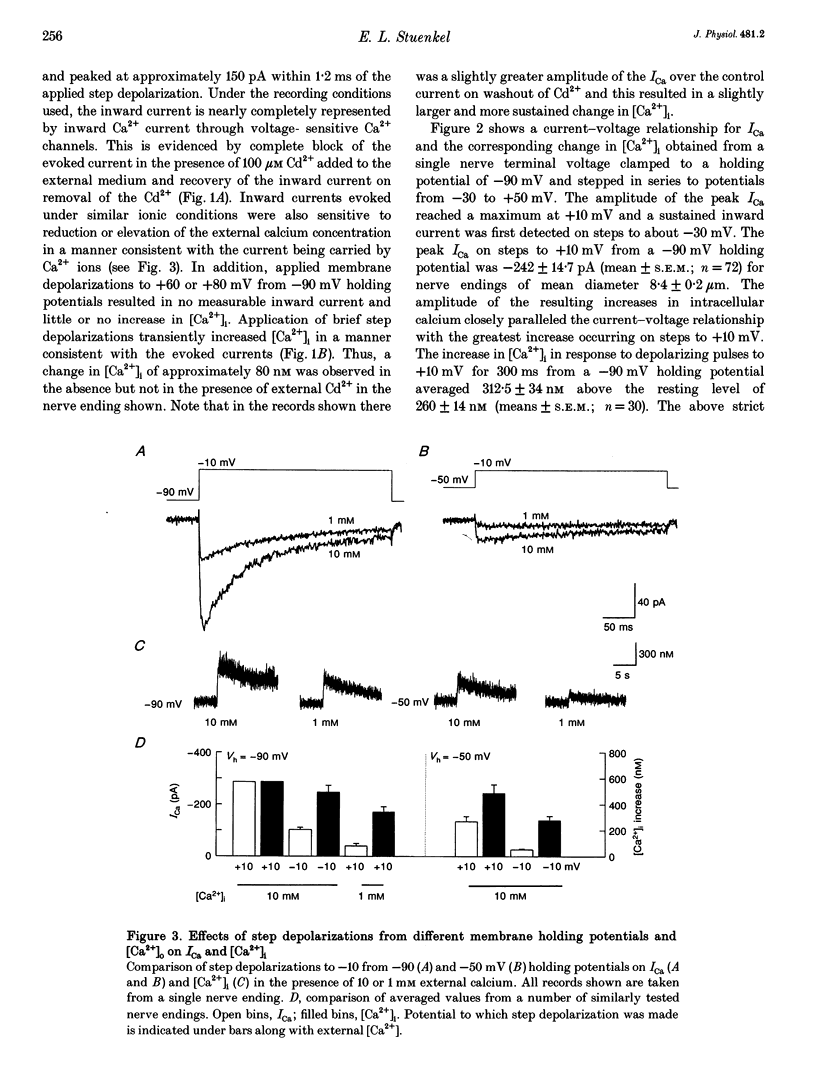
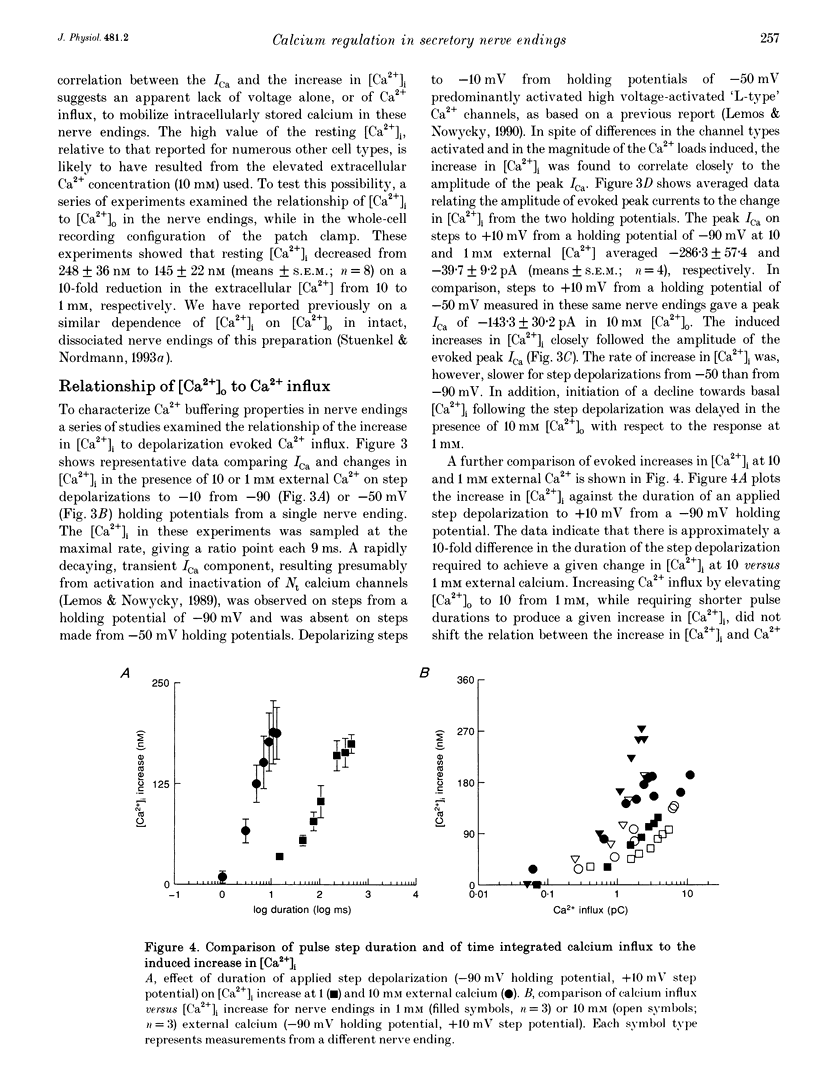
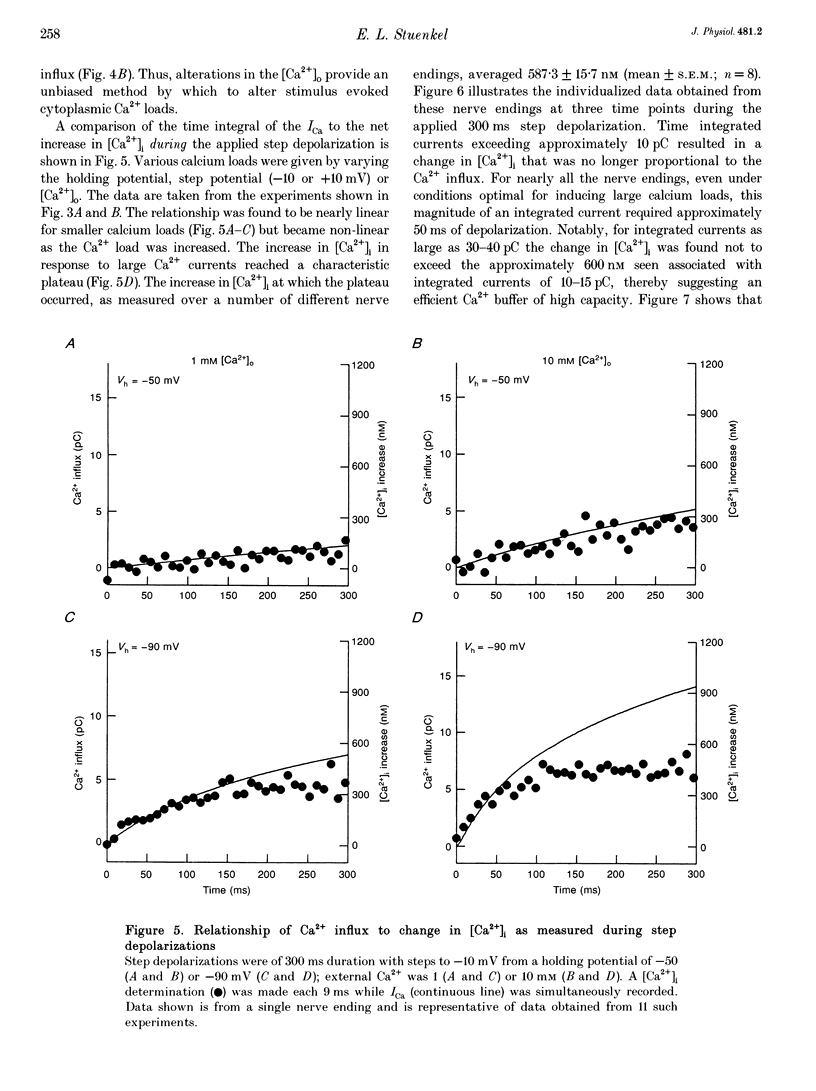
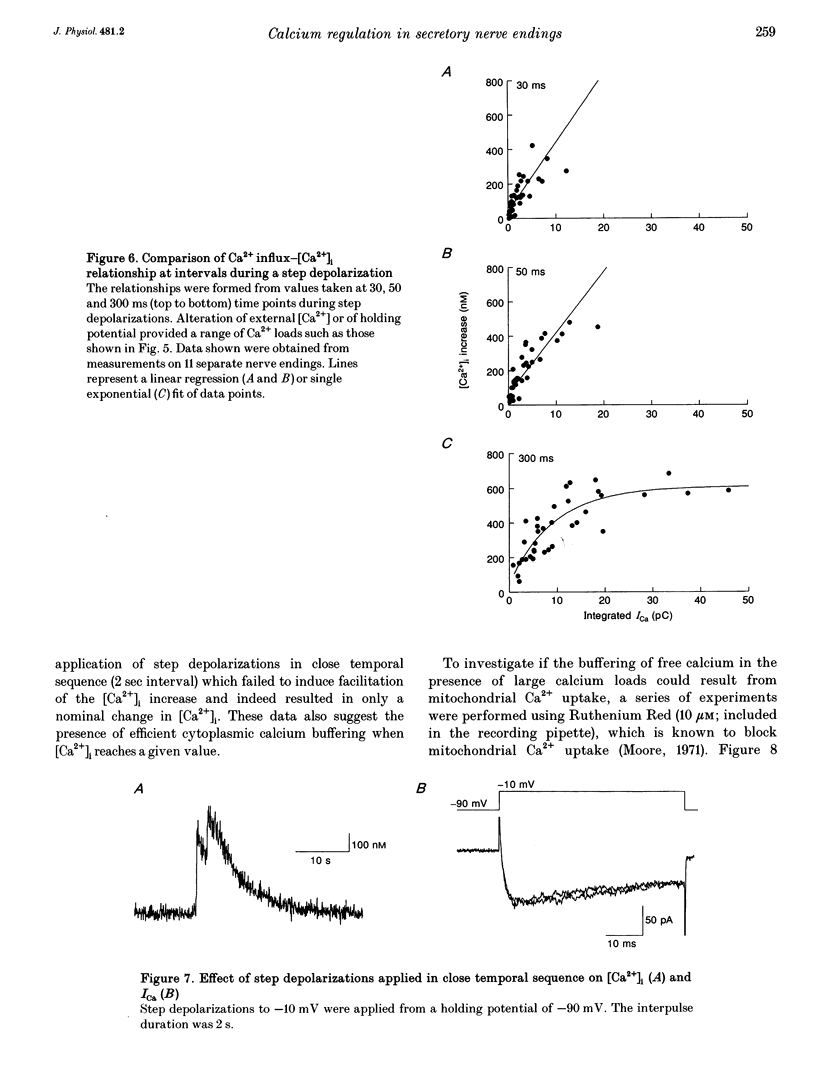
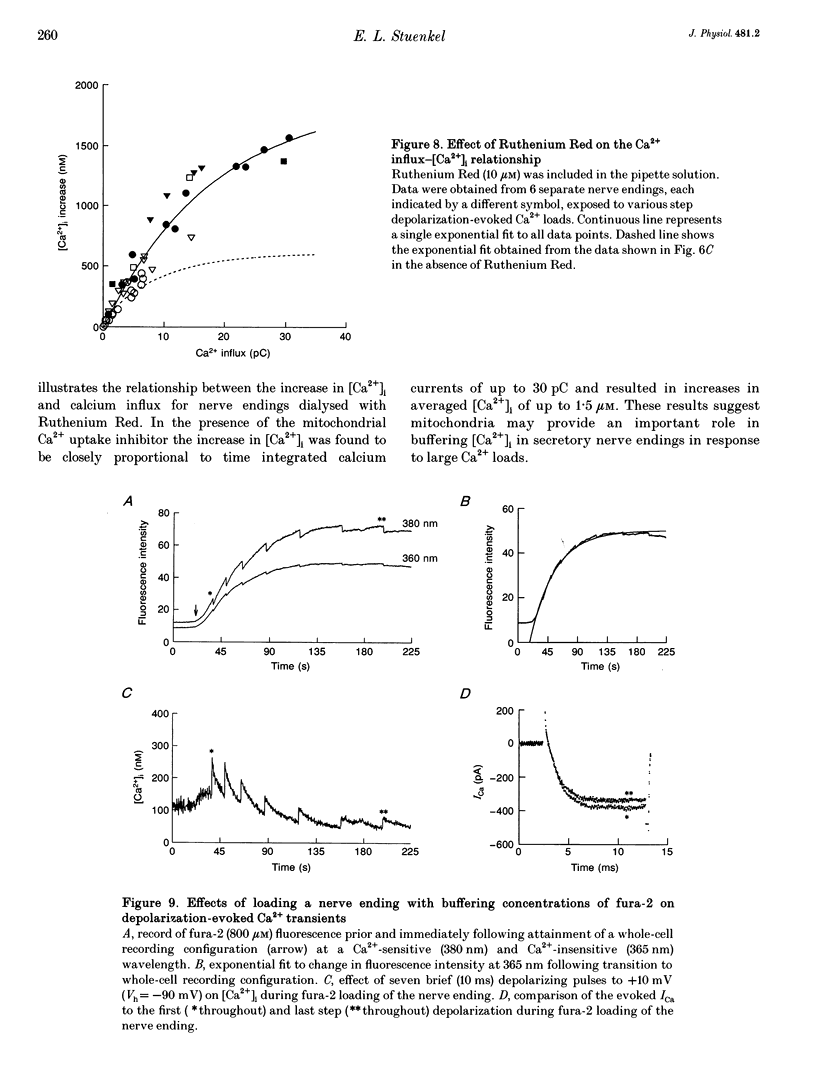
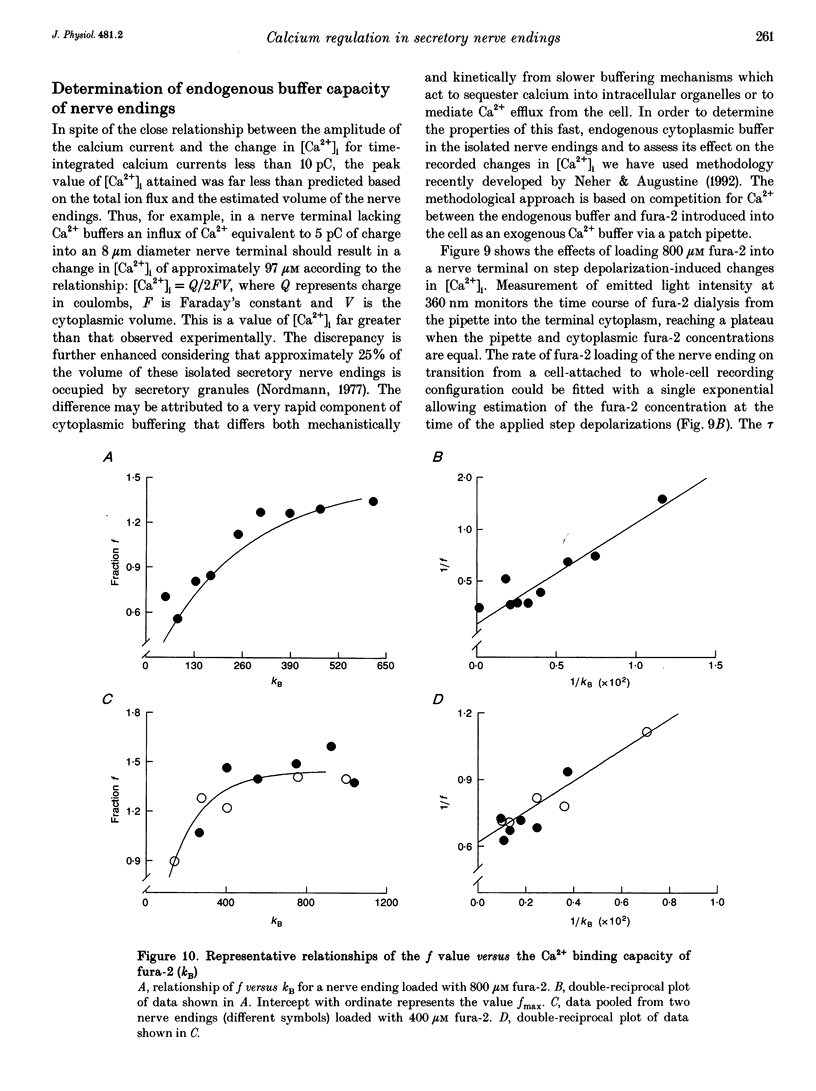
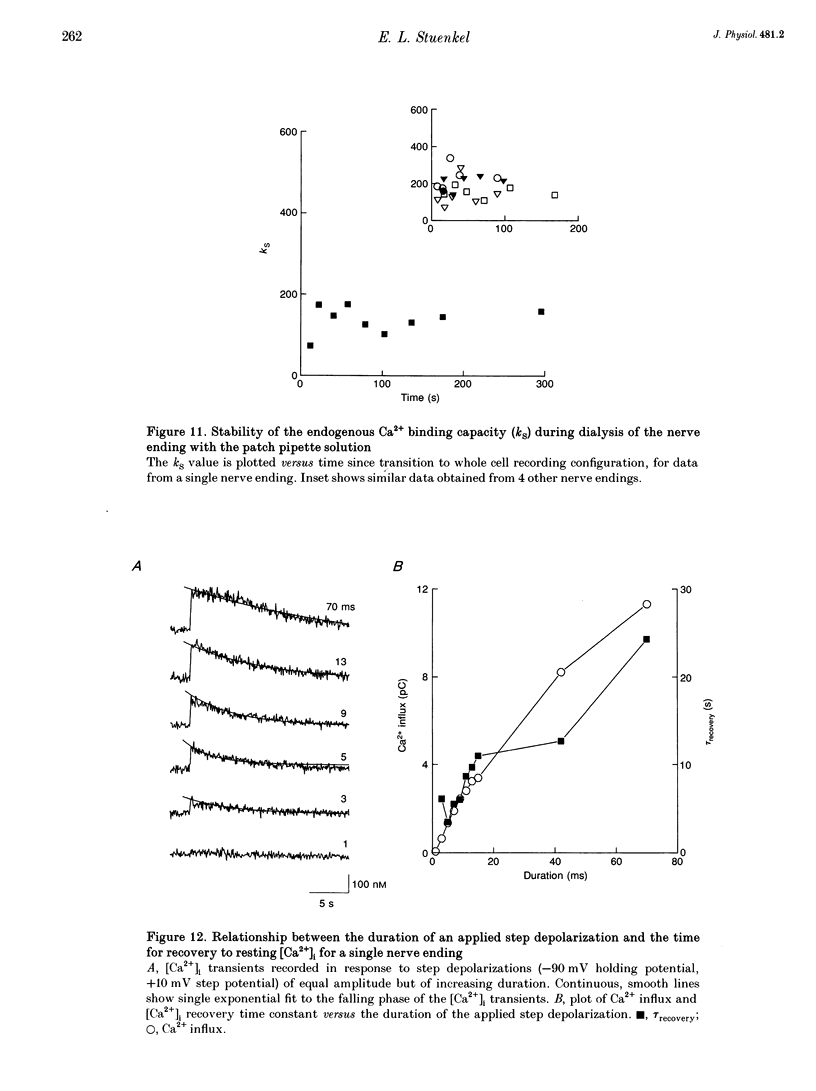
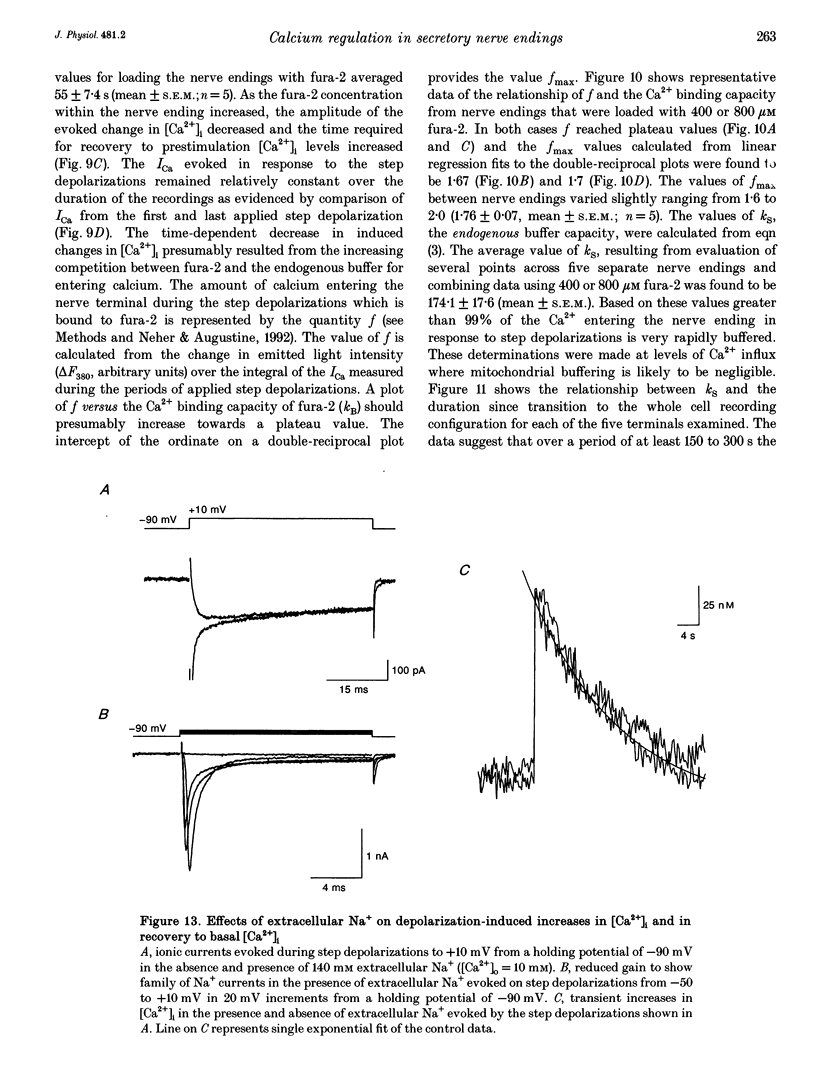
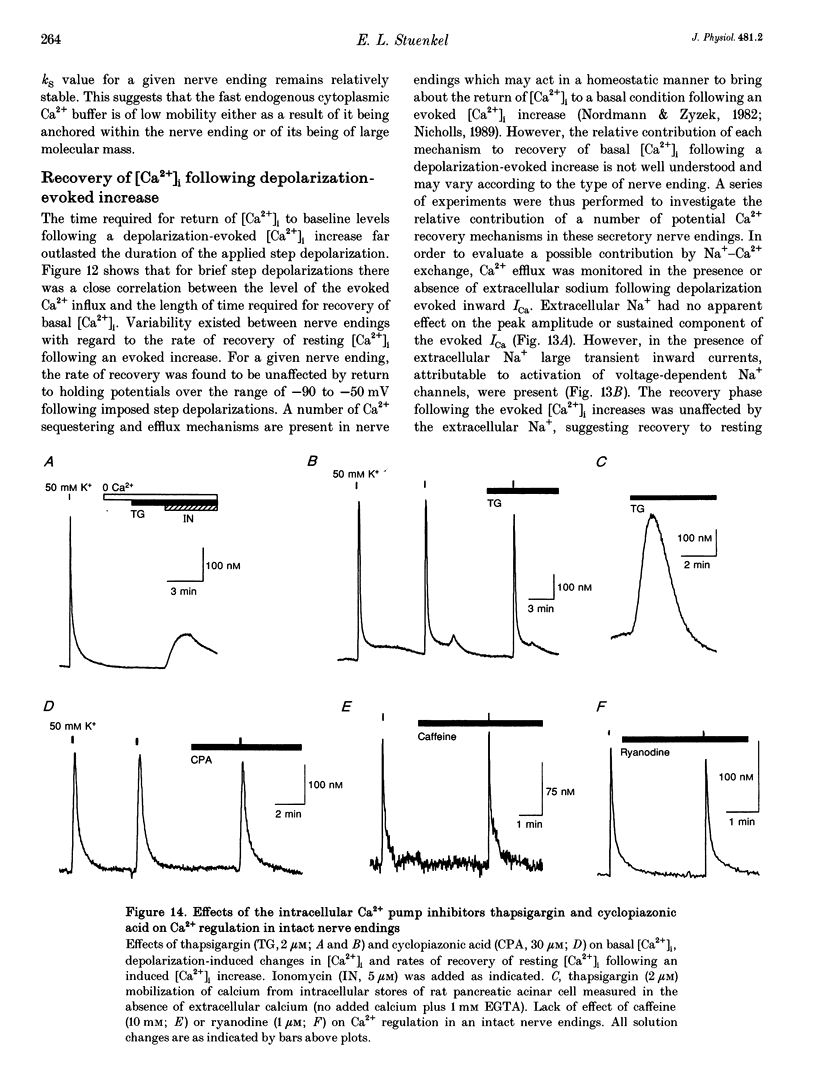
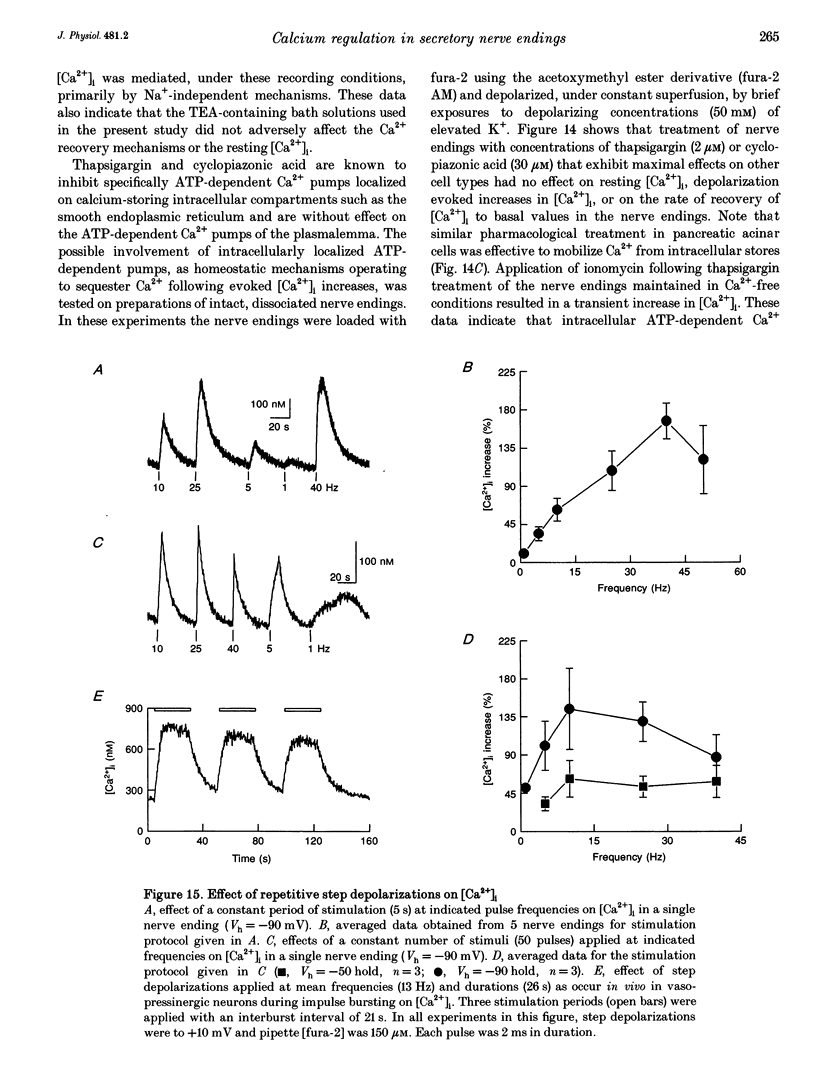
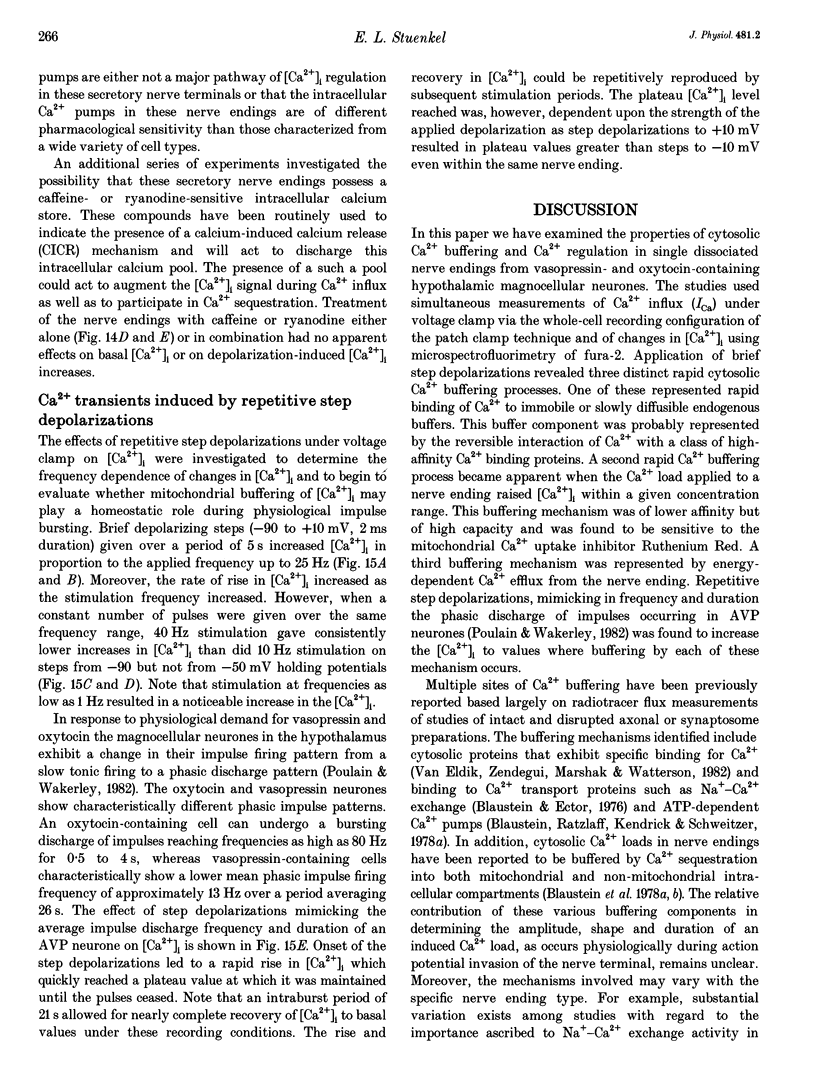
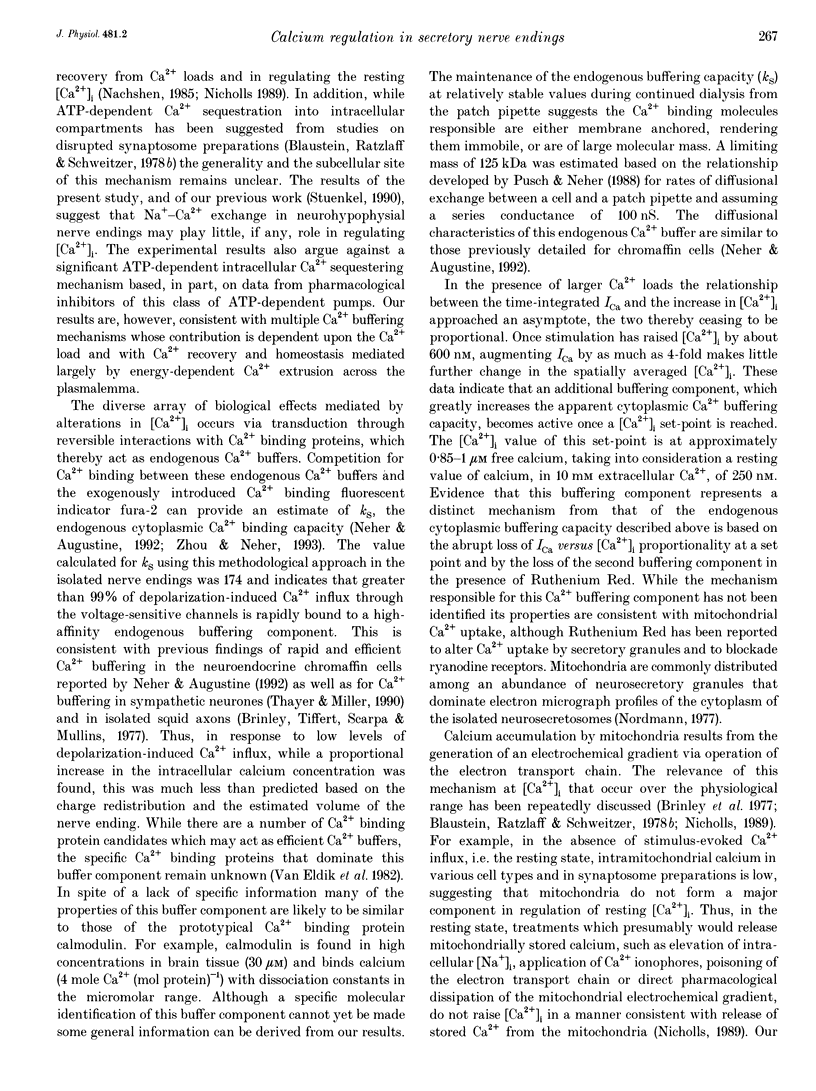
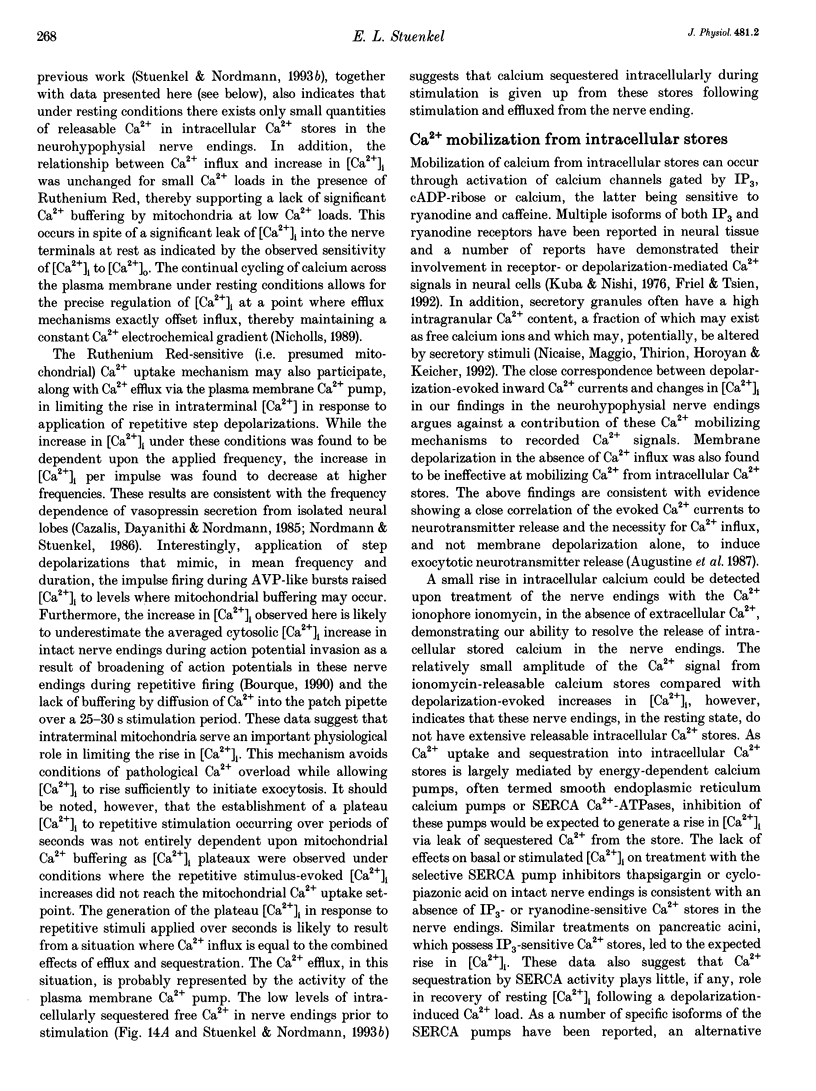
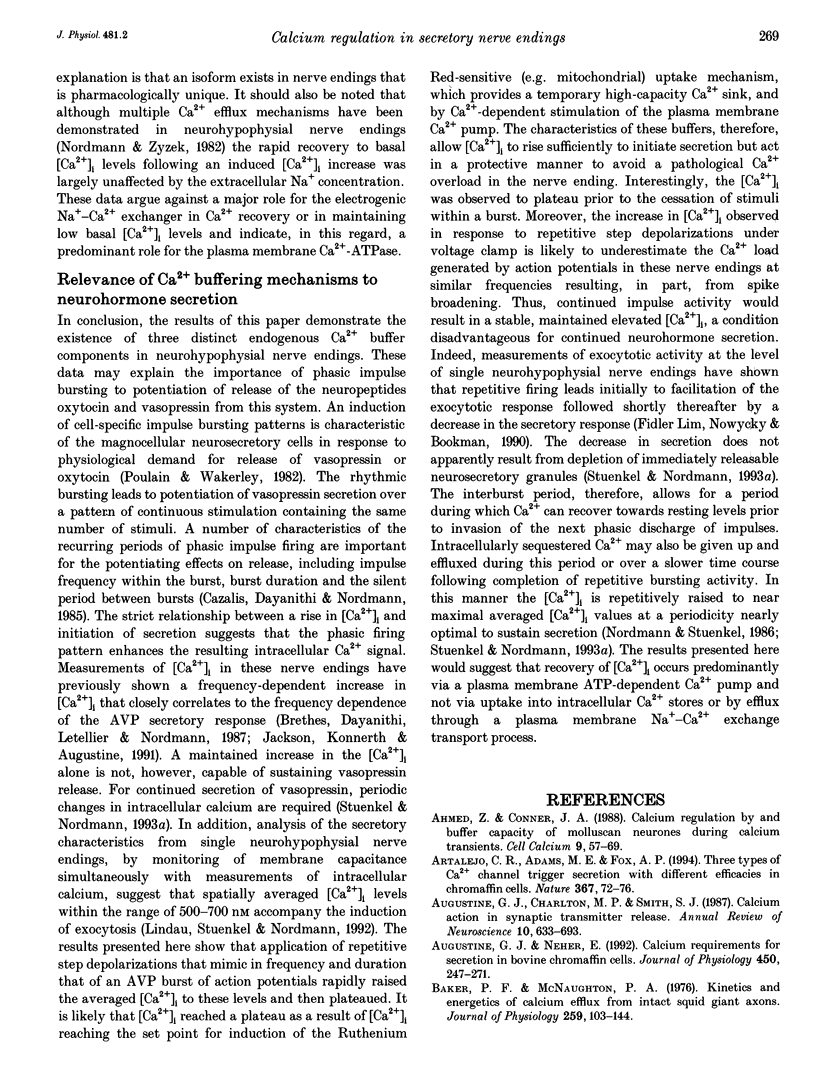
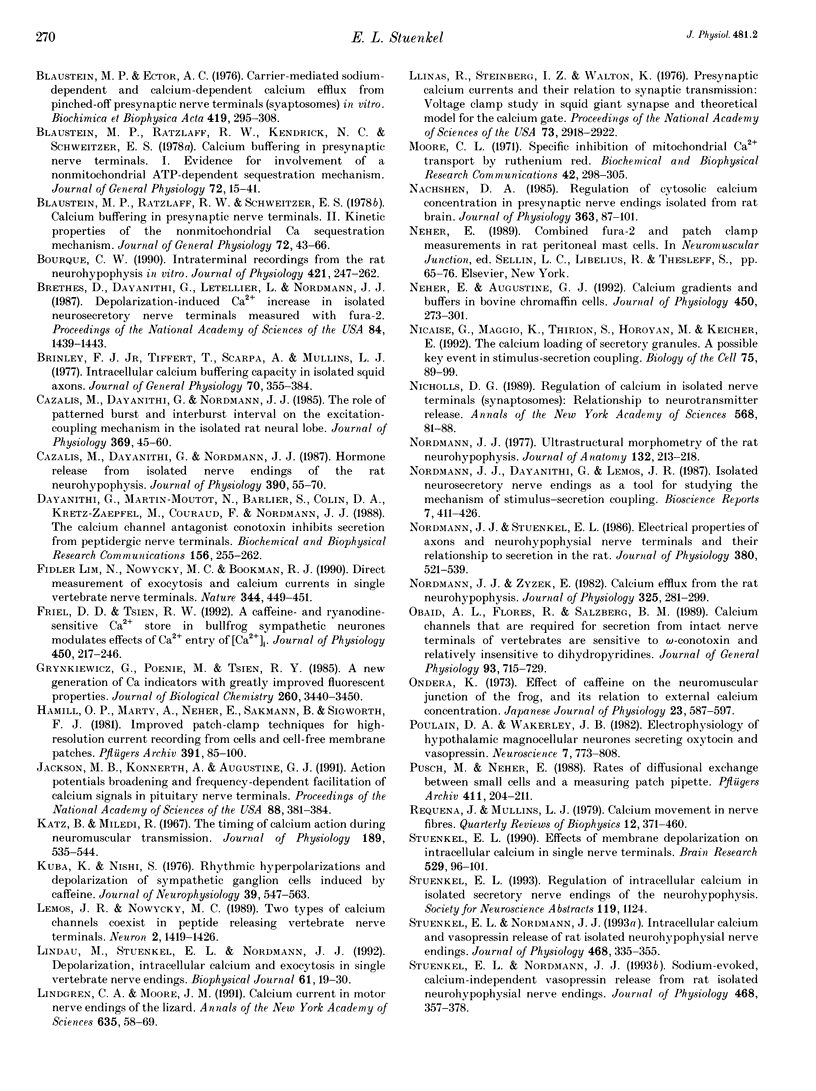
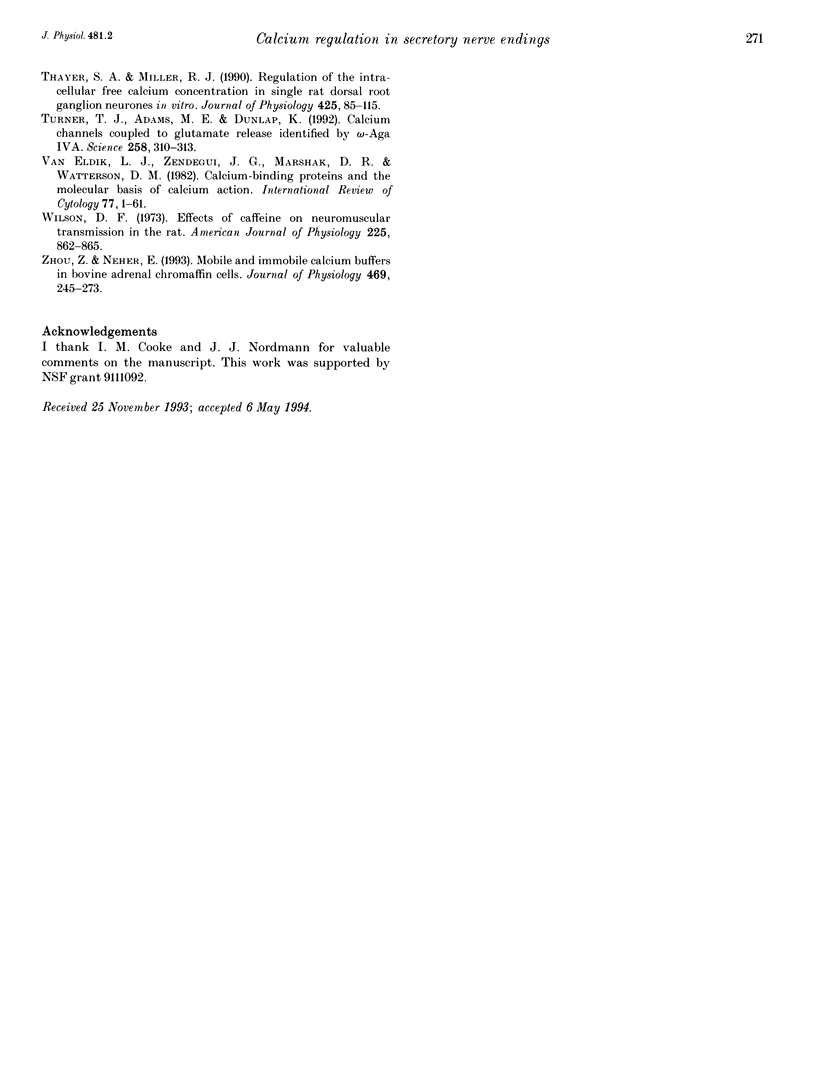
Selected References
These references are in PubMed. This may not be the complete list of references from this article.
- Ahmed Z., Connor J. A. Calcium regulation by and buffer capacity of molluscan neurons during calcium transients. Cell Calcium. 1988 Apr;9(2):57–69. doi: 10.1016/0143-4160(88)90025-5. [DOI] [PubMed] [Google Scholar]
- Artalejo C. R., Adams M. E., Fox A. P. Three types of Ca2+ channel trigger secretion with different efficacies in chromaffin cells. Nature. 1994 Jan 6;367(6458):72–76. doi: 10.1038/367072a0. [DOI] [PubMed] [Google Scholar]
- Augustine G. J., Charlton M. P., Smith S. J. Calcium action in synaptic transmitter release. Annu Rev Neurosci. 1987;10:633–693. doi: 10.1146/annurev.ne.10.030187.003221. [DOI] [PubMed] [Google Scholar]
- Augustine G. J., Neher E. Calcium requirements for secretion in bovine chromaffin cells. J Physiol. 1992 May;450:247–271. doi: 10.1113/jphysiol.1992.sp019126. [DOI] [PMC free article] [PubMed] [Google Scholar]
- Baker P. F., McNaughton P. A. Kinetics and energetics of calcium efflux from intact squid giant axons. J Physiol. 1976 Jul;259(1):103–144. doi: 10.1113/jphysiol.1976.sp011457. [DOI] [PMC free article] [PubMed] [Google Scholar]
- Blaustein M. P., Ector A. C. Carrier-mediated sodium-dependent and calcium-dependent calcium efflux from pinched-off presynaptic nerve terminals (synaptosomes) in vitro. Biochim Biophys Acta. 1976 Jan 21;419(2):295–308. doi: 10.1016/0005-2736(76)90355-2. [DOI] [PubMed] [Google Scholar]
- Blaustein M. P., Ratzlaff R. W., Kendrick N. C., Schweitzer E. S. Calcium buffering in presynaptic nerve terminals. I. Evidence for involvement of a nonmitochondrial ATP-dependent sequestration mechanism. J Gen Physiol. 1978 Jul;72(1):15–41. doi: 10.1085/jgp.72.1.15. [DOI] [PMC free article] [PubMed] [Google Scholar]
- Blaustein M. P., Ratzlaff R. W., Schweitzer E. S. Calcium buffering in presynaptic nerve terminals. II. Kinetic properties of the nonmitochondrial Ca sequestration mechanism. J Gen Physiol. 1978 Jul;72(1):43–66. doi: 10.1085/jgp.72.1.43. [DOI] [PMC free article] [PubMed] [Google Scholar]
- Bourque C. W. Intraterminal recordings from the rat neurohypophysis in vitro. J Physiol. 1990 Feb;421:247–262. doi: 10.1113/jphysiol.1990.sp017943. [DOI] [PMC free article] [PubMed] [Google Scholar]
- Brethes D., Dayanithi G., Letellier L., Nordmann J. J. Depolarization-induced Ca2+ increase in isolated neurosecretory nerve terminals measured with fura-2. Proc Natl Acad Sci U S A. 1987 Mar;84(5):1439–1443. doi: 10.1073/pnas.84.5.1439. [DOI] [PMC free article] [PubMed] [Google Scholar]
- Brinley F. J., Jr, Tiffert T., Scarpa A., Mullins L. J. Intracellular calcium buffering capacity in isolated squid axons. J Gen Physiol. 1977 Sep;70(3):355–384. doi: 10.1085/jgp.70.3.355. [DOI] [PMC free article] [PubMed] [Google Scholar]
- Cazalis M., Dayanithi G., Nordmann J. J. Hormone release from isolated nerve endings of the rat neurohypophysis. J Physiol. 1987 Sep;390:55–70. doi: 10.1113/jphysiol.1987.sp016686. [DOI] [PMC free article] [PubMed] [Google Scholar]
- Cazalis M., Dayanithi G., Nordmann J. J. The role of patterned burst and interburst interval on the excitation-coupling mechanism in the isolated rat neural lobe. J Physiol. 1985 Dec;369:45–60. doi: 10.1113/jphysiol.1985.sp015887. [DOI] [PMC free article] [PubMed] [Google Scholar]
- Dayanithi G., Martin-Moutot N., Barlier S., Colin D. A., Kretz-Zaepfel M., Couraud F., Nordmann J. J. The calcium channel antagonist omega-conotoxin inhibits secretion from peptidergic nerve terminals. Biochem Biophys Res Commun. 1988 Oct 14;156(1):255–262. doi: 10.1016/s0006-291x(88)80833-7. [DOI] [PubMed] [Google Scholar]
- Friel D. D., Tsien R. W. A caffeine- and ryanodine-sensitive Ca2+ store in bullfrog sympathetic neurones modulates effects of Ca2+ entry on [Ca2+]i. J Physiol. 1992 May;450:217–246. doi: 10.1113/jphysiol.1992.sp019125. [DOI] [PMC free article] [PubMed] [Google Scholar]
- Grynkiewicz G., Poenie M., Tsien R. Y. A new generation of Ca2+ indicators with greatly improved fluorescence properties. J Biol Chem. 1985 Mar 25;260(6):3440–3450. [PubMed] [Google Scholar]
- Hamill O. P., Marty A., Neher E., Sakmann B., Sigworth F. J. Improved patch-clamp techniques for high-resolution current recording from cells and cell-free membrane patches. Pflugers Arch. 1981 Aug;391(2):85–100. doi: 10.1007/BF00656997. [DOI] [PubMed] [Google Scholar]
- Jackson M. B., Konnerth A., Augustine G. J. Action potential broadening and frequency-dependent facilitation of calcium signals in pituitary nerve terminals. Proc Natl Acad Sci U S A. 1991 Jan 15;88(2):380–384. doi: 10.1073/pnas.88.2.380. [DOI] [PMC free article] [PubMed] [Google Scholar]
- Katz B., Miledi R. The timing of calcium action during neuromuscular transmission. J Physiol. 1967 Apr;189(3):535–544. doi: 10.1113/jphysiol.1967.sp008183. [DOI] [PMC free article] [PubMed] [Google Scholar]
- Kuba K., Nishi S. Rhythmic hyperpolarizations and depolarization of sympathetic ganglion cells induced by caffeine. J Neurophysiol. 1976 May;39(3):547–563. doi: 10.1152/jn.1976.39.3.547. [DOI] [PubMed] [Google Scholar]
- Lemos J. R., Nowycky M. C. Two types of calcium channels coexist in peptide-releasing vertebrate nerve terminals. Neuron. 1989 May;2(5):1419–1426. doi: 10.1016/0896-6273(89)90187-6. [DOI] [PubMed] [Google Scholar]
- Lim N. F., Nowycky M. C., Bookman R. J. Direct measurement of exocytosis and calcium currents in single vertebrate nerve terminals. Nature. 1990 Mar 29;344(6265):449–451. doi: 10.1038/344449a0. [DOI] [PubMed] [Google Scholar]
- Lindau M., Stuenkel E. L., Nordmann J. J. Depolarization, intracellular calcium and exocytosis in single vertebrate nerve endings. Biophys J. 1992 Jan;61(1):19–30. doi: 10.1016/S0006-3495(92)81812-X. [DOI] [PMC free article] [PubMed] [Google Scholar]
- Lindgren C. A., Moore J. W. Calcium current in motor nerve endings of the lizard. Ann N Y Acad Sci. 1991;635:58–69. doi: 10.1111/j.1749-6632.1991.tb36481.x. [DOI] [PubMed] [Google Scholar]
- Llinás R., Steinberg I. Z., Walton K. Presynaptic calcium currents and their relation to synaptic transmission: voltage clamp study in squid giant synapse and theoretical model for the calcium gate. Proc Natl Acad Sci U S A. 1976 Aug;73(8):2918–2922. doi: 10.1073/pnas.73.8.2918. [DOI] [PMC free article] [PubMed] [Google Scholar]
- Moore C. L. Specific inhibition of mitochondrial Ca++ transport by ruthenium red. Biochem Biophys Res Commun. 1971 Jan 22;42(2):298–305. doi: 10.1016/0006-291x(71)90102-1. [DOI] [PubMed] [Google Scholar]
- Nachshen D. A. Regulation of cytosolic calcium concentration in presynaptic nerve endings isolated from rat brain. J Physiol. 1985 Jun;363:87–101. doi: 10.1113/jphysiol.1985.sp015697. [DOI] [PMC free article] [PubMed] [Google Scholar]
- Neher E., Augustine G. J. Calcium gradients and buffers in bovine chromaffin cells. J Physiol. 1992 May;450:273–301. doi: 10.1113/jphysiol.1992.sp019127. [DOI] [PMC free article] [PubMed] [Google Scholar]
- Nicaise G., Maggio K., Thirion S., Horoyan M., Keicher E. The calcium loading of secretory granules. A possible key event in stimulus-secretion coupling. Biol Cell. 1992;75(2):89–99. doi: 10.1016/0248-4900(92)90128-n. [DOI] [PubMed] [Google Scholar]
- Nicholls D. G. Regulation of calcium in isolated nerve terminals (synaptosomes): relationship to neurotransmitter release. Ann N Y Acad Sci. 1989;568:81–88. doi: 10.1111/j.1749-6632.1989.tb12493.x. [DOI] [PubMed] [Google Scholar]
- Nordmann J. J., Dayanithi G., Lemos J. R. Isolated neurosecretory nerve endings as a tool for studying the mechanism of stimulus-secretion coupling. Biosci Rep. 1987 May;7(5):411–426. doi: 10.1007/BF01362504. [DOI] [PubMed] [Google Scholar]
- Nordmann J. J., Stuenkel E. L. Electrical properties of axons and neurohypophysial nerve terminals and their relationship to secretion in the rat. J Physiol. 1986 Nov;380:521–539. doi: 10.1113/jphysiol.1986.sp016300. [DOI] [PMC free article] [PubMed] [Google Scholar]
- Nordmann J. J. Ultrastructural morphometry of the rat neurohypophysis. J Anat. 1977 Feb;123(Pt 1):213–218. [PMC free article] [PubMed] [Google Scholar]
- Nordmann J. J., Zyzek E. Calcium efflux from the rat neurohypophysis. J Physiol. 1982 Apr;325:281–299. doi: 10.1113/jphysiol.1982.sp014150. [DOI] [PMC free article] [PubMed] [Google Scholar]
- Obaid A. L., Flores R., Salzberg B. M. Calcium channels that are required for secretion from intact nerve terminals of vertebrates are sensitive to omega-conotoxin and relatively insensitive to dihydropyridines. Optical studies with and without voltage-sensitive dyes. J Gen Physiol. 1989 Apr;93(4):715–729. doi: 10.1085/jgp.93.4.715. [DOI] [PMC free article] [PubMed] [Google Scholar]
- Onodera K. Effect of caffeine on the neuromuscular junction of the frog, and its relation to external calcium concentration. Jpn J Physiol. 1973 Dec;23(6):587–597. doi: 10.2170/jjphysiol.23.587. [DOI] [PubMed] [Google Scholar]
- Poulain D. A., Wakerley J. B. Electrophysiology of hypothalamic magnocellular neurones secreting oxytocin and vasopressin. Neuroscience. 1982 Apr;7(4):773–808. doi: 10.1016/0306-4522(82)90044-6. [DOI] [PubMed] [Google Scholar]
- Pusch M., Neher E. Rates of diffusional exchange between small cells and a measuring patch pipette. Pflugers Arch. 1988 Feb;411(2):204–211. doi: 10.1007/BF00582316. [DOI] [PubMed] [Google Scholar]
- Requena J., Mullins L. J. Calcium movement in nerve fibres. Q Rev Biophys. 1979 Aug;12(3):371–460. doi: 10.1017/s0033583500005473. [DOI] [PubMed] [Google Scholar]
- Stuenkel E. L. Effects of membrane depolarization on intracellular calcium in single nerve terminals. Brain Res. 1990 Oct 8;529(1-2):96–101. doi: 10.1016/0006-8993(90)90815-s. [DOI] [PubMed] [Google Scholar]
- Stuenkel E. L., Nordmann J. J. Intracellular calcium and vasopressin release of rat isolated neurohypophysial nerve endings. J Physiol. 1993 Aug;468:335–355. doi: 10.1113/jphysiol.1993.sp019775. [DOI] [PMC free article] [PubMed] [Google Scholar]
- Stuenkel E. L., Nordmann J. J. Sodium-evoked, calcium-independent vasopressin release from rat isolated neurohypophysial nerve endings. J Physiol. 1993 Aug;468:357–378. doi: 10.1113/jphysiol.1993.sp019776. [DOI] [PMC free article] [PubMed] [Google Scholar]
- Thayer S. A., Miller R. J. Regulation of the intracellular free calcium concentration in single rat dorsal root ganglion neurones in vitro. J Physiol. 1990 Jun;425:85–115. doi: 10.1113/jphysiol.1990.sp018094. [DOI] [PMC free article] [PubMed] [Google Scholar]
- Turner T. J., Adams M. E., Dunlap K. Calcium channels coupled to glutamate release identified by omega-Aga-IVA. Science. 1992 Oct 9;258(5080):310–313. doi: 10.1126/science.1357749. [DOI] [PubMed] [Google Scholar]
- Van Eldik L. J., Zendegui J. G., Marshak D. R., Watterson D. M. Calcium-binding proteins and the molecular basis of calcium action. Int Rev Cytol. 1982;77:1–61. doi: 10.1016/s0074-7696(08)62463-8. [DOI] [PubMed] [Google Scholar]
- Wilson D. F. Effects of caffeine on neuromuscular transmission in the rat. Am J Physiol. 1973 Oct;225(4):862–865. doi: 10.1152/ajplegacy.1973.225.4.862. [DOI] [PubMed] [Google Scholar]
- Zhou Z., Neher E. Mobile and immobile calcium buffers in bovine adrenal chromaffin cells. J Physiol. 1993 Sep;469:245–273. doi: 10.1113/jphysiol.1993.sp019813. [DOI] [PMC free article] [PubMed] [Google Scholar]