Abstract
1. The relation between mitochondrial membrane potential (delta psi m) and cell function was investigated in single adult rat cardiac myocytes during anoxia and reoxygenation. delta psi m was studied by loading myocytes with JC-1 (5,5',6,6'-tetrachloro-1,1',3,3'- tetra-ethylbenzimidazolylcarbocyanine iodide), a fluorescent probe characterized by two emission peaks (539 and 597 nm with excitation at 490 nm) corresponding to monomer and aggregate forms of the dye. 2. De-energizing conditions applied to mitochondria, cell suspensions or single cells decreased the aggregate emission and increased the monomer emission. This latter result cannot be explained by changes of JC-1 concentration in the aqueous mitochondrial matrix phase indicating that hydrophobic interaction of the probe with membranes has to be taken into account to explain JC-1 fluorescence properties in isolated mitochondria or intact cells. 3. A different sensitivity of the two JC-1 forms to delta psi m changes was shown in isolated mitochondria by the effects of ADP and FCCP and the calibration with K+ diffusion potentials. The monomer emission was responsive to values of delta psi m below 140 mV, which hardly modified the aggregate emission. Thus JC-1 represents a unique double sensor which can provide semi-quantitative information in both low and high potential ranges. 4. At the onset of glucose-free anoxia the epifluorescence of individual myocytes studied in the single excitation (490 nm)-double emission (530 and 590 nm) mode showed a gradual decline of the aggregate emission, which reached a plateau while electrically stimulated (0.2 Hz) contraction was still retained. The subsequent failure of contraction was followed by the rise of the emission at 530 nm, corresponding to the monomer form of the dye, concomitantly with the development of rigor contracture. 5. The onset of the rigor was preceded by the increase in intracellular Mg2+ concentration ([Mg2+]i) monitored by mag-indo-1 epifluorescence. Since under these experimental conditions intracellular [Ca2+] and pH are fairly stable, the increase in [Mg2+]i was likely to be produced by a decrease in ATP content. 6. The inhibition of mitochondrial ATPase induced by oligomycin during anoxia was associated with a rapid and simultaneous change of both the components of JC-1 fluorescence, suggesting that delta psi m, instead of producing ATP, is generated by glycolytic ATP during anoxia. 7. The readmission of oxygen induced a rapid decrease of the monomer emission and a slower increase of the aggregate emission. These fluorescence changes were not necessarily associated with the recovery of mechanical function.(ABSTRACT TRUNCATED AT 400 WORDS)
Full text
PDF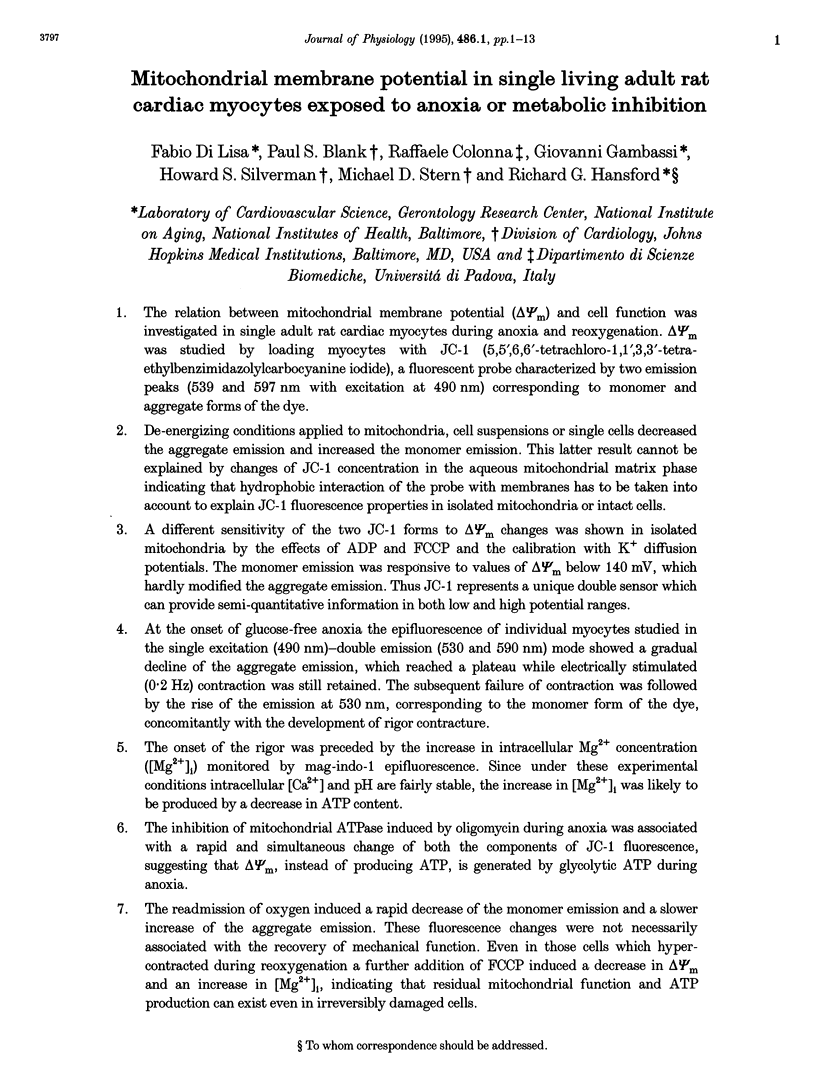
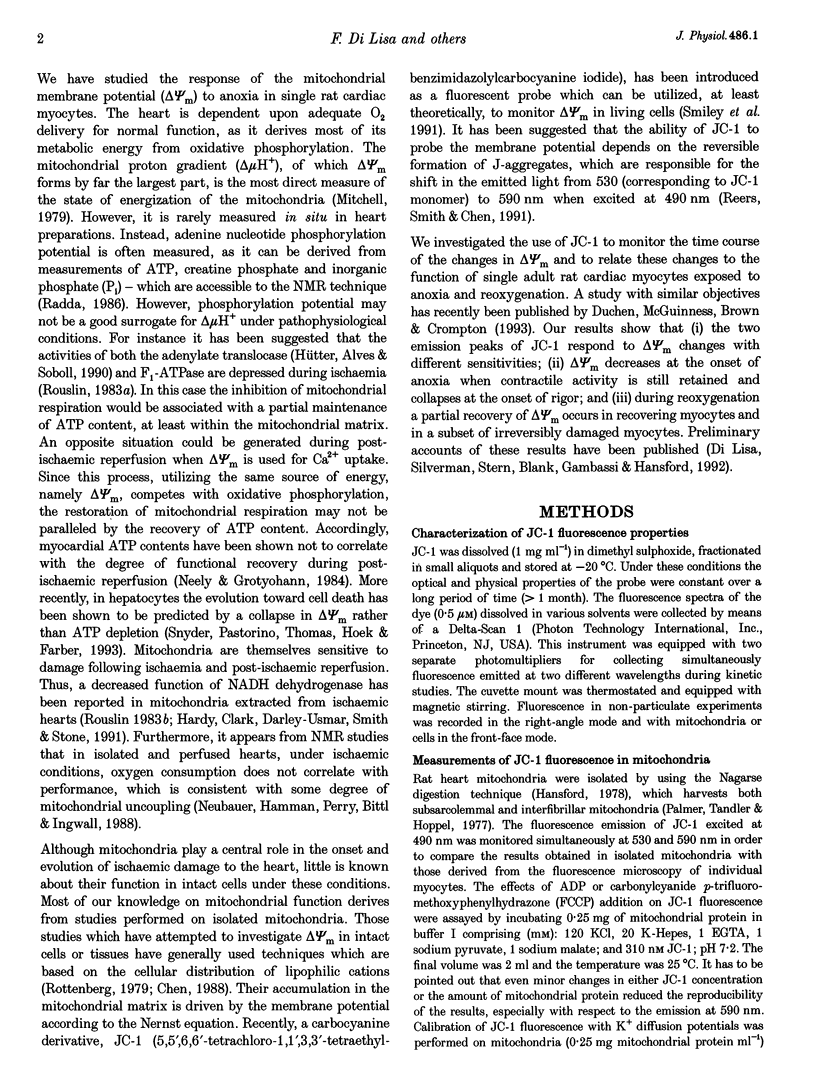
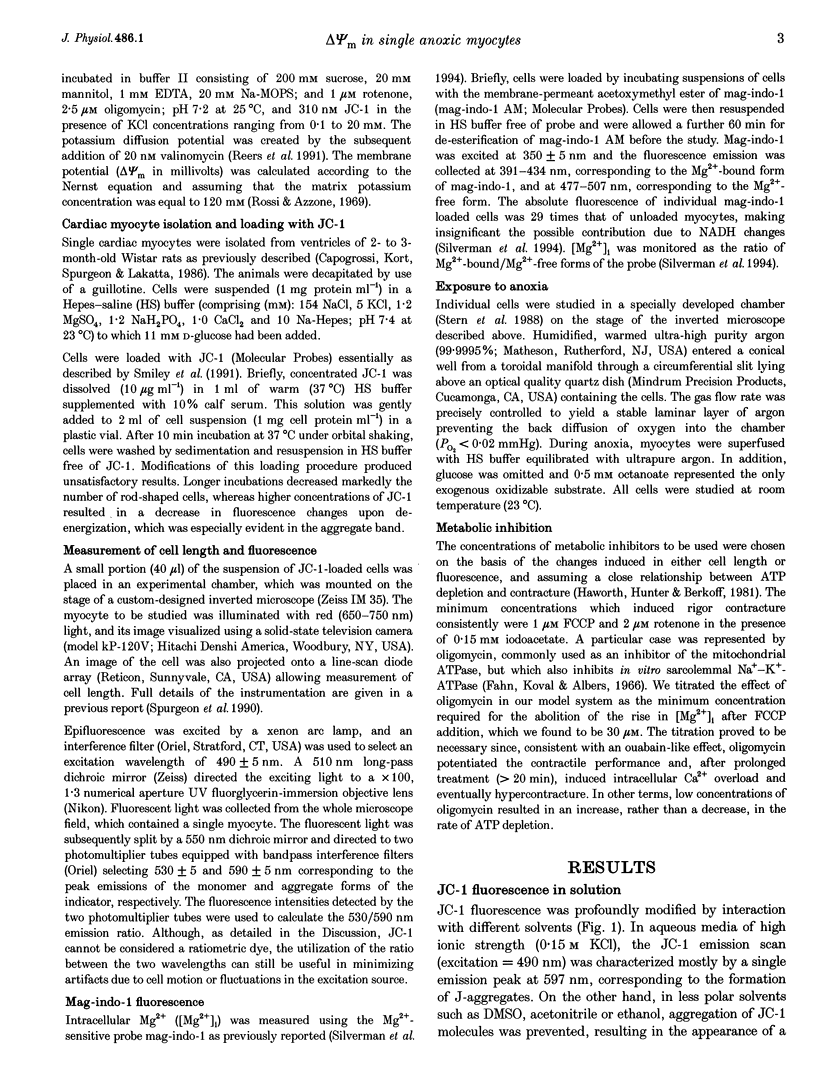
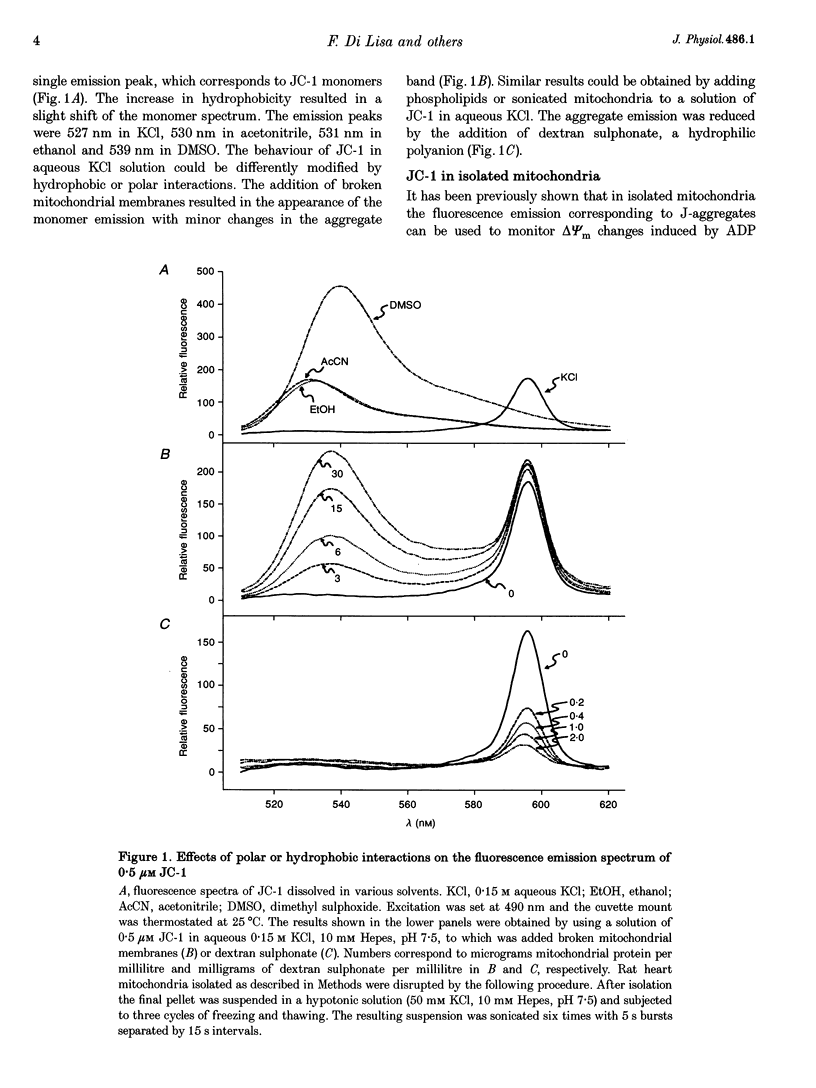
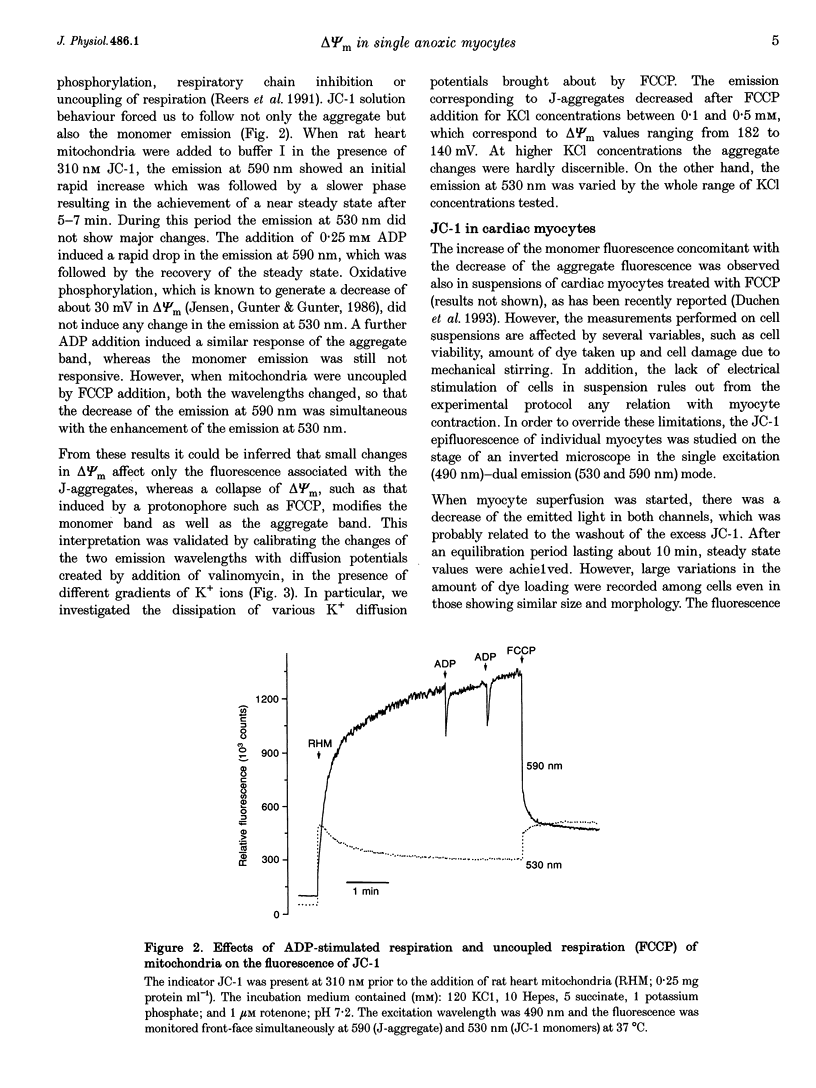
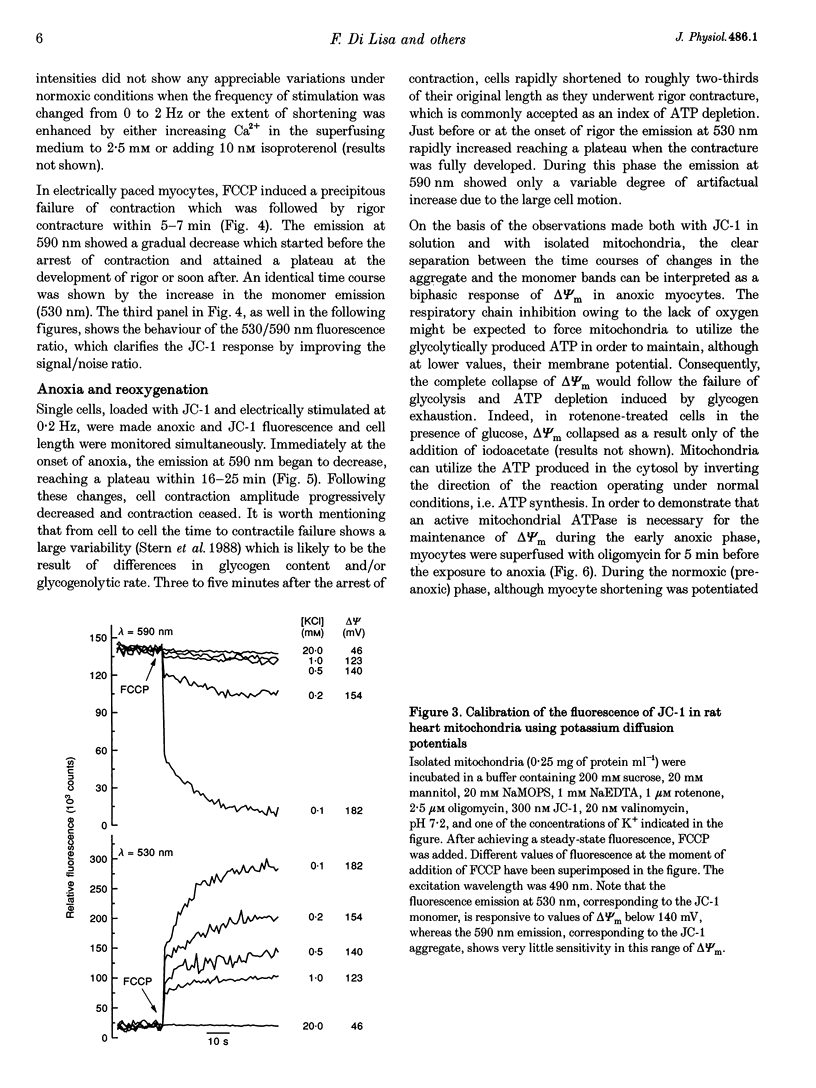
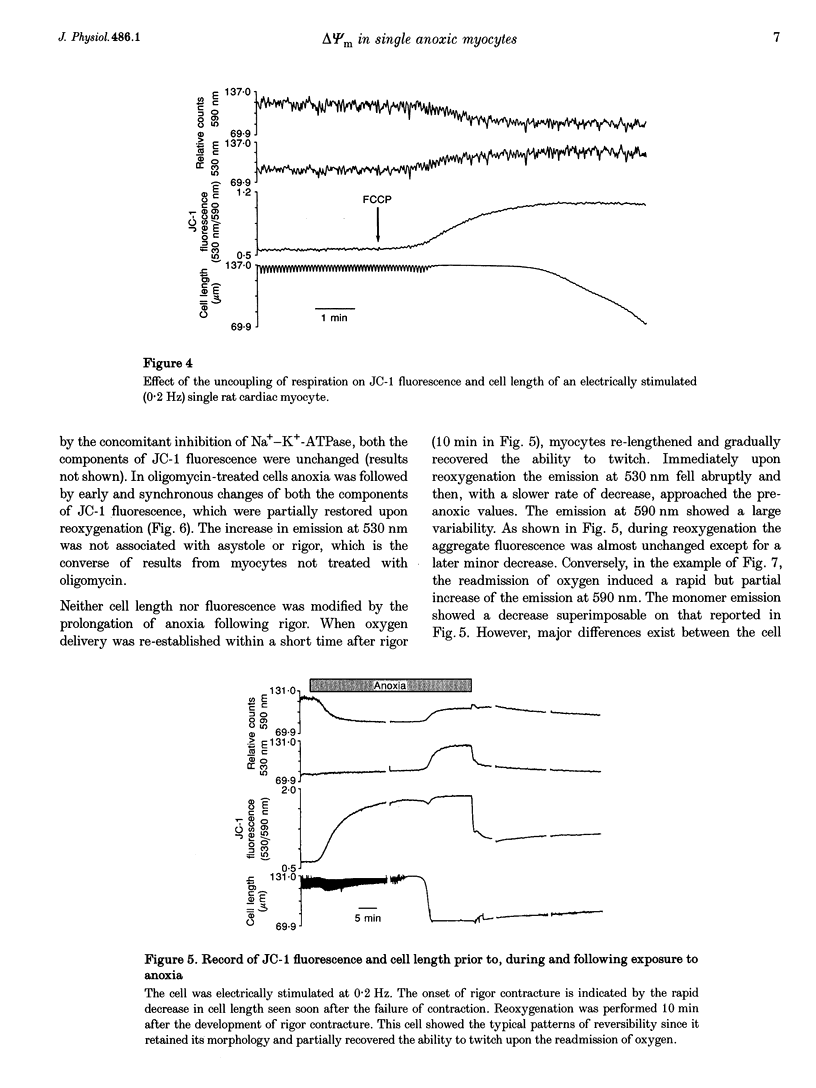
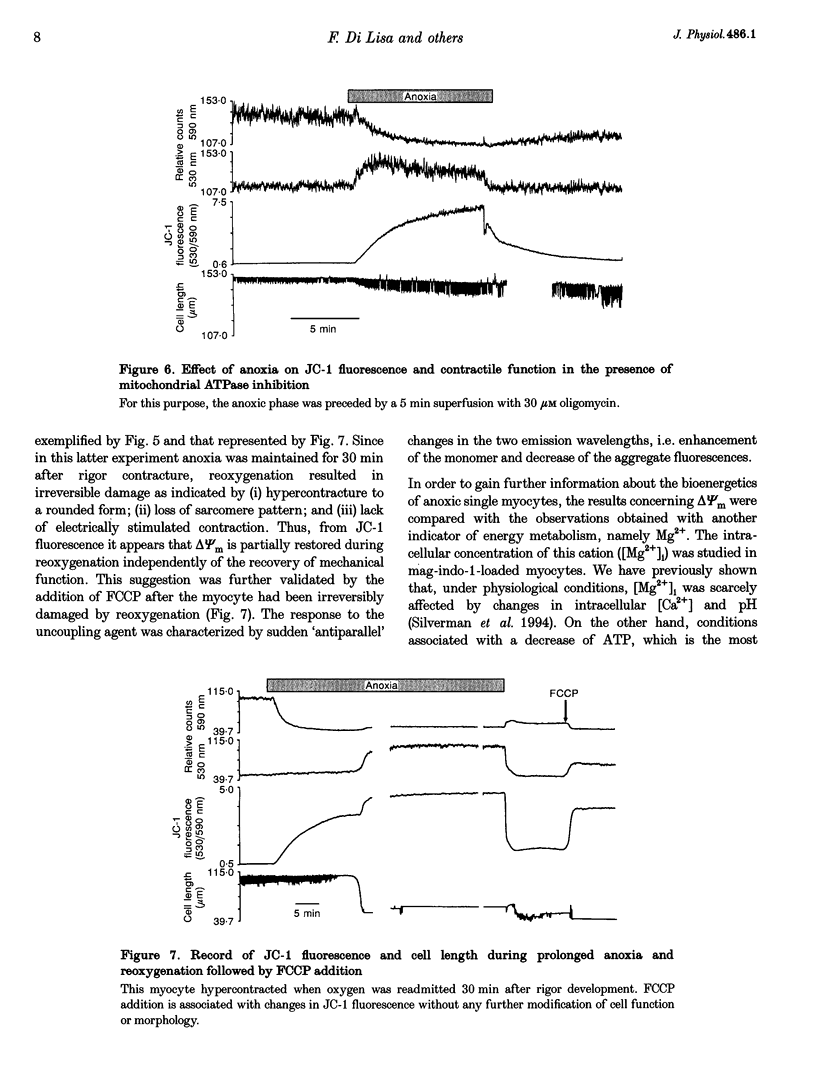
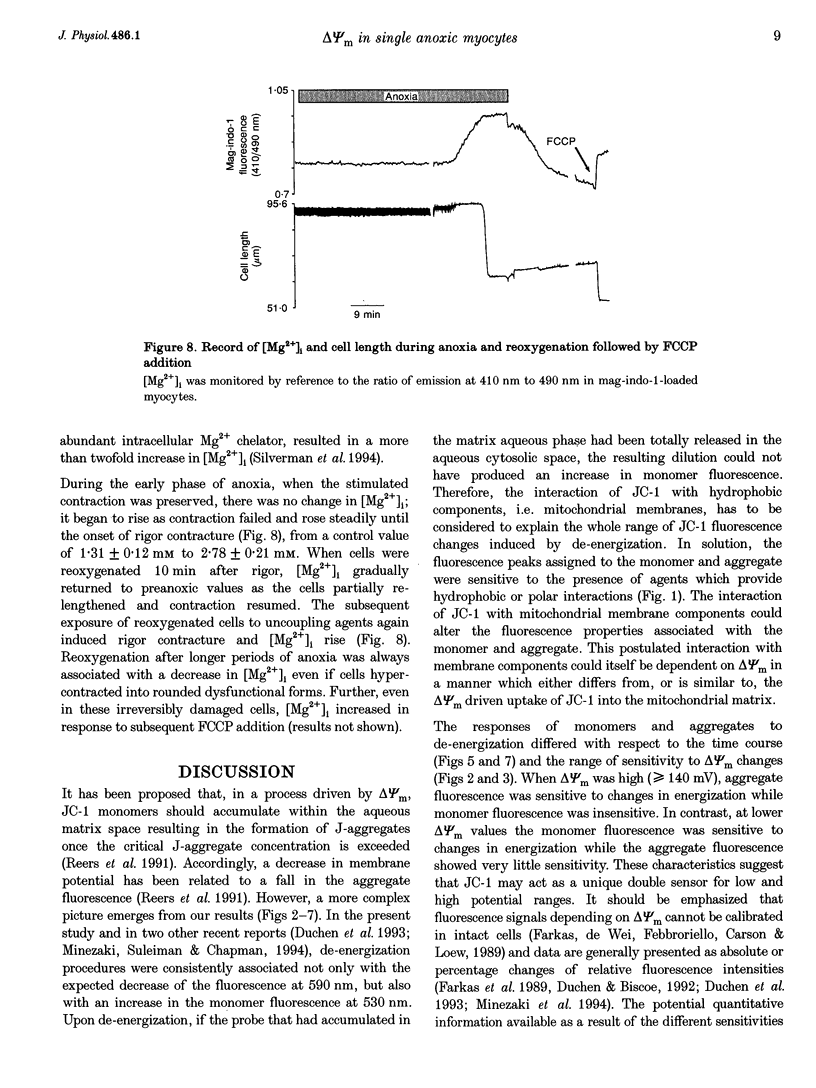
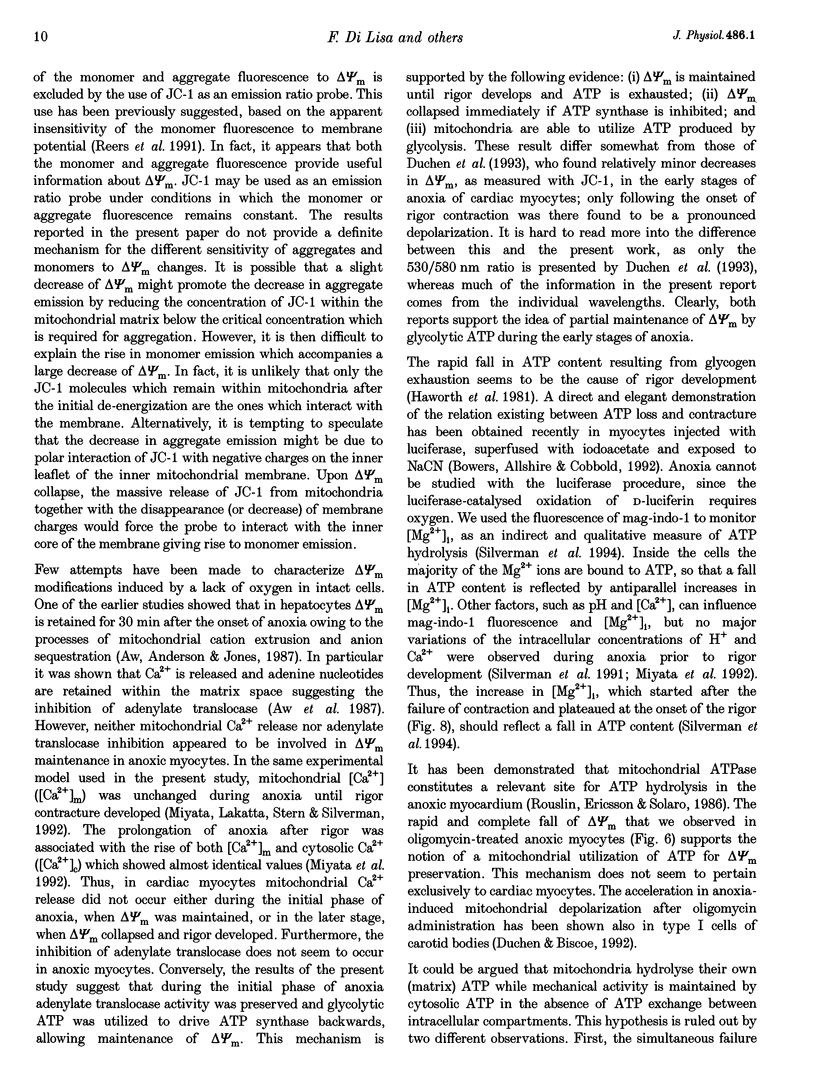
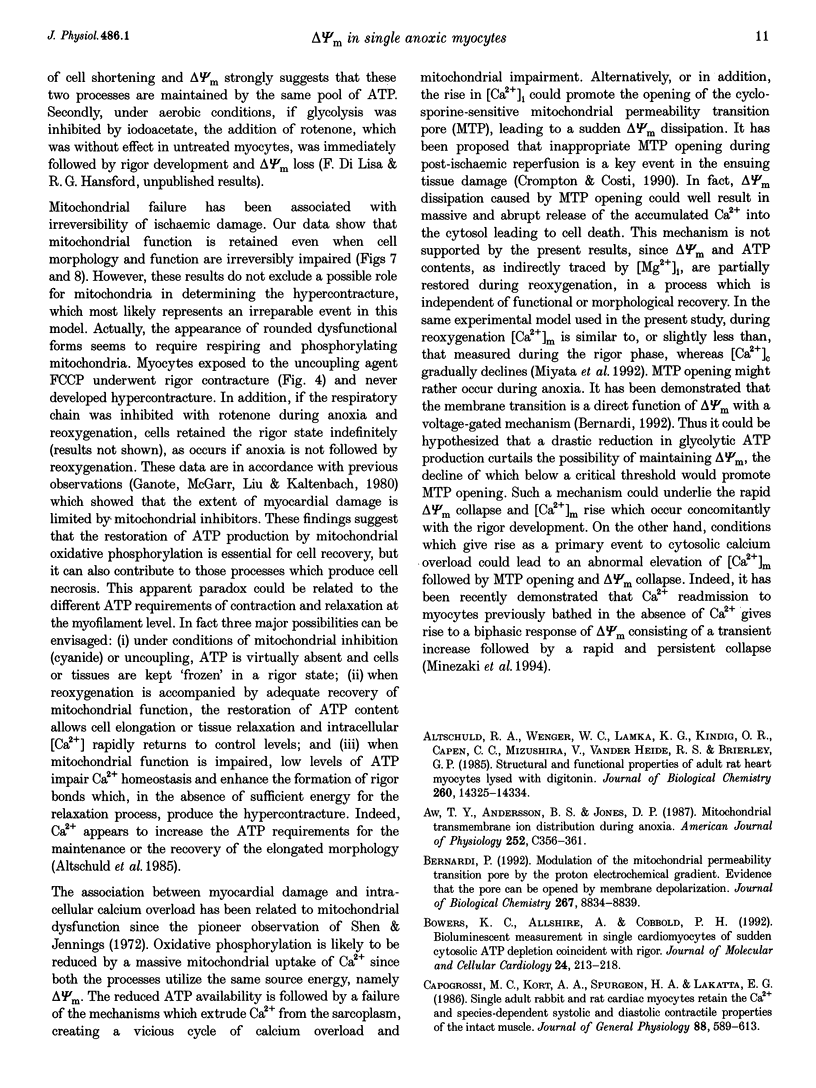
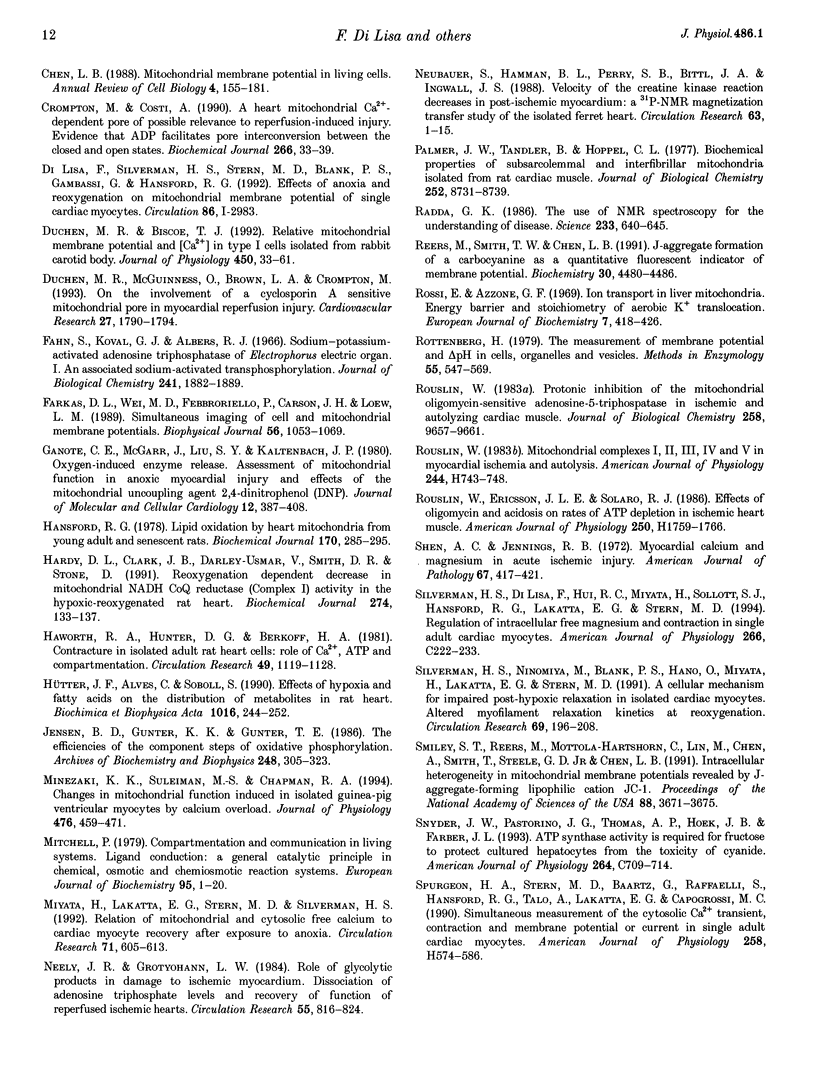
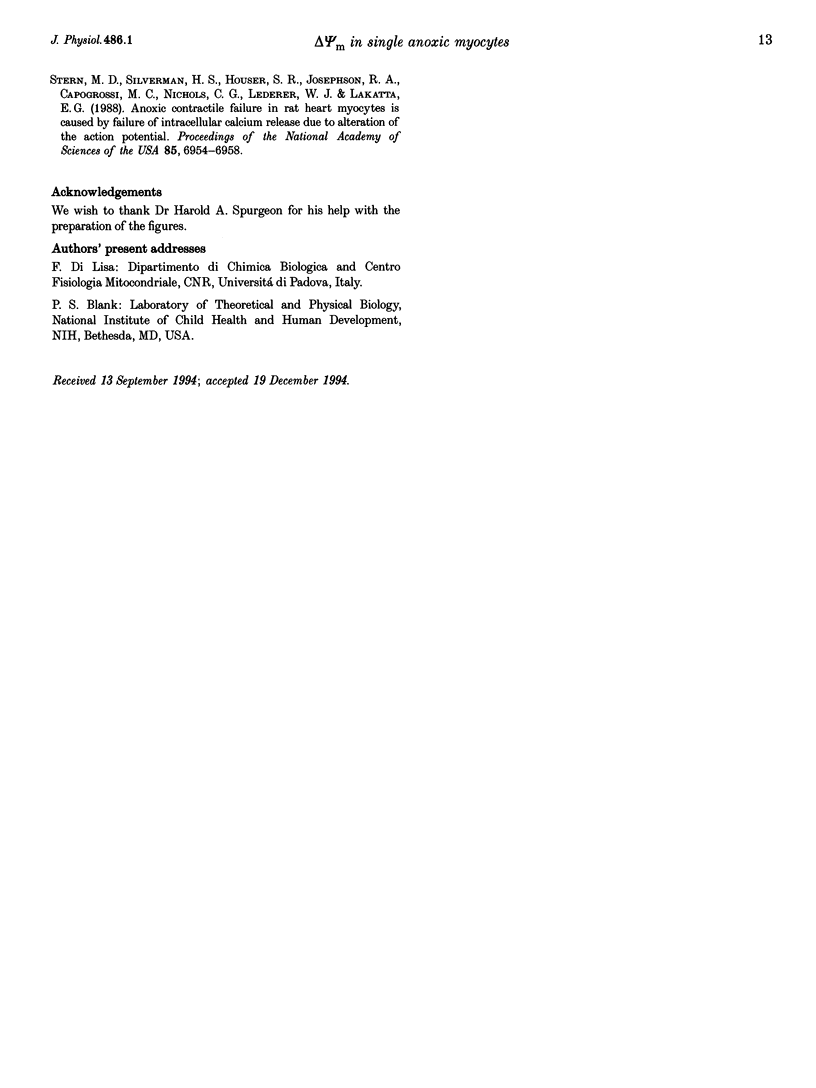
Images in this article
Selected References
These references are in PubMed. This may not be the complete list of references from this article.
- Altschuld R. A., Wenger W. C., Lamka K. G., Kindig O. R., Capen C. C., Mizuhira V., Vander Heide R. S., Brierley G. P. Structural and functional properties of adult rat heart myocytes lysed with digitonin. J Biol Chem. 1985 Nov 15;260(26):14325–14334. [PubMed] [Google Scholar]
- Aw T. Y., Andersson B. S., Jones D. P. Mitochondrial transmembrane ion distribution during anoxia. Am J Physiol. 1987 Apr;252(4 Pt 1):C356–C361. doi: 10.1152/ajpcell.1987.252.4.C356. [DOI] [PubMed] [Google Scholar]
- Bernardi P. Modulation of the mitochondrial cyclosporin A-sensitive permeability transition pore by the proton electrochemical gradient. Evidence that the pore can be opened by membrane depolarization. J Biol Chem. 1992 May 5;267(13):8834–8839. [PubMed] [Google Scholar]
- Bowers K. C., Allshire A. P., Cobbold P. H. Bioluminescent measurement in single cardiomyocytes of sudden cytosolic ATP depletion coincident with rigor. J Mol Cell Cardiol. 1992 Mar;24(3):213–218. doi: 10.1016/0022-2828(92)93159-h. [DOI] [PubMed] [Google Scholar]
- Capogrossi M. C., Kort A. A., Spurgeon H. A., Lakatta E. G. Single adult rabbit and rat cardiac myocytes retain the Ca2+- and species-dependent systolic and diastolic contractile properties of intact muscle. J Gen Physiol. 1986 Nov;88(5):589–613. doi: 10.1085/jgp.88.5.589. [DOI] [PMC free article] [PubMed] [Google Scholar]
- Chen L. B. Mitochondrial membrane potential in living cells. Annu Rev Cell Biol. 1988;4:155–181. doi: 10.1146/annurev.cb.04.110188.001103. [DOI] [PubMed] [Google Scholar]
- Crompton M., Costi A. A heart mitochondrial Ca2(+)-dependent pore of possible relevance to re-perfusion-induced injury. Evidence that ADP facilitates pore interconversion between the closed and open states. Biochem J. 1990 Feb 15;266(1):33–39. doi: 10.1042/bj2660033. [DOI] [PMC free article] [PubMed] [Google Scholar]
- Duchen M. R., Biscoe T. J. Relative mitochondrial membrane potential and [Ca2+]i in type I cells isolated from the rabbit carotid body. J Physiol. 1992 May;450:33–61. doi: 10.1113/jphysiol.1992.sp019115. [DOI] [PMC free article] [PubMed] [Google Scholar]
- Duchen M. R., McGuinness O., Brown L. A., Crompton M. On the involvement of a cyclosporin A sensitive mitochondrial pore in myocardial reperfusion injury. Cardiovasc Res. 1993 Oct;27(10):1790–1794. doi: 10.1093/cvr/27.10.1790. [DOI] [PubMed] [Google Scholar]
- Fahn S., Koval G. J., Albers R. W. Sodium-potassium-activated adenosine triphosphatase of Electrophorus electric organ. I. An associated sodium-activated transphosphorylation. J Biol Chem. 1966 Apr 25;241(8):1882–1889. [PubMed] [Google Scholar]
- Farkas D. L., Wei M. D., Febbroriello P., Carson J. H., Loew L. M. Simultaneous imaging of cell and mitochondrial membrane potentials. Biophys J. 1989 Dec;56(6):1053–1069. doi: 10.1016/S0006-3495(89)82754-7. [DOI] [PMC free article] [PubMed] [Google Scholar]
- Ganote C. E., McGarr J., Liu S. Y., Kaltenbach J. P. Oxygen-induced enzyme release. Assessment of mitochondrial function in anoxic myocardial injury and effects of the mitochondrial uncoupling agent 2,4-dinitrophenol (DNP). J Mol Cell Cardiol. 1980 Apr;12(4):387–408. doi: 10.1016/0022-2828(80)90049-8. [DOI] [PubMed] [Google Scholar]
- Hansford R. G. Lipid oxidation by heart mitochondria from young adult and senescent rats. Biochem J. 1978 Feb 15;170(2):285–295. doi: 10.1042/bj1700285. [DOI] [PMC free article] [PubMed] [Google Scholar]
- Hardy L., Clark J. B., Darley-Usmar V. M., Smith D. R., Stone D. Reoxygenation-dependent decrease in mitochondrial NADH:CoQ reductase (Complex I) activity in the hypoxic/reoxygenated rat heart. Biochem J. 1991 Feb 15;274(Pt 1):133–137. doi: 10.1042/bj2740133. [DOI] [PMC free article] [PubMed] [Google Scholar]
- Haworth R. A., Hunter D. R., Berkoff H. A. Contracture in isolated adult rat heart cells. Role of Ca2+, ATP, and compartmentation. Circ Res. 1981 Nov;49(5):1119–1128. doi: 10.1161/01.res.49.5.1119. [DOI] [PubMed] [Google Scholar]
- Hütter J. F., Alves C., Soboll S. Effects of hypoxia and fatty acids on the distribution of metabolites in rat heart. Biochim Biophys Acta. 1990 Apr 5;1016(2):244–252. doi: 10.1016/0005-2728(90)90065-c. [DOI] [PubMed] [Google Scholar]
- Ion transport in liver mitochondria. Energy barrier and stoicheometry of aerobic K+ translocation. Eur J Biochem. 1969 Jan;7(3):418–426. doi: 10.1111/j.1432-1033.1969.tb19626.x. [DOI] [PubMed] [Google Scholar]
- Jensen B. D., Gunter K. K., Gunter T. E. The efficiencies of the component steps of oxidative phosphorylation. II. Experimental determination of the efficiencies in mitochondria and examination of the equivalence of membrane potential and pH gradient in phosphorylation. Arch Biochem Biophys. 1986 Jul;248(1):305–323. doi: 10.1016/0003-9861(86)90427-3. [DOI] [PubMed] [Google Scholar]
- Minezaki K. K., Suleiman M. S., Chapman R. A. Changes in mitochondrial function induced in isolated guinea-pig ventricular myocytes by calcium overload. J Physiol. 1994 May 1;476(3):459–471. doi: 10.1113/jphysiol.1994.sp020147. [DOI] [PMC free article] [PubMed] [Google Scholar]
- Mitchell P. The Ninth Sir Hans Krebs Lecture. Compartmentation and communication in living systems. Ligand conduction: a general catalytic principle in chemical, osmotic and chemiosmotic reaction systems. Eur J Biochem. 1979 Mar 15;95(1):1–20. doi: 10.1111/j.1432-1033.1979.tb12934.x. [DOI] [PubMed] [Google Scholar]
- Miyata H., Lakatta E. G., Stern M. D., Silverman H. S. Relation of mitochondrial and cytosolic free calcium to cardiac myocyte recovery after exposure to anoxia. Circ Res. 1992 Sep;71(3):605–613. doi: 10.1161/01.res.71.3.605. [DOI] [PubMed] [Google Scholar]
- Neely J. R., Grotyohann L. W. Role of glycolytic products in damage to ischemic myocardium. Dissociation of adenosine triphosphate levels and recovery of function of reperfused ischemic hearts. Circ Res. 1984 Dec;55(6):816–824. doi: 10.1161/01.res.55.6.816. [DOI] [PubMed] [Google Scholar]
- Neubauer S., Hamman B. L., Perry S. B., Bittl J. A., Ingwall J. S. Velocity of the creatine kinase reaction decreases in postischemic myocardium: a 31P-NMR magnetization transfer study of the isolated ferret heart. Circ Res. 1988 Jul;63(1):1–15. doi: 10.1161/01.res.63.1.1. [DOI] [PubMed] [Google Scholar]
- Palmer J. W., Tandler B., Hoppel C. L. Biochemical properties of subsarcolemmal and interfibrillar mitochondria isolated from rat cardiac muscle. J Biol Chem. 1977 Dec 10;252(23):8731–8739. [PubMed] [Google Scholar]
- Radda G. K. The use of NMR spectroscopy for the understanding of disease. Science. 1986 Aug 8;233(4764):640–645. doi: 10.1126/science.3726553. [DOI] [PubMed] [Google Scholar]
- Reers M., Smith T. W., Chen L. B. J-aggregate formation of a carbocyanine as a quantitative fluorescent indicator of membrane potential. Biochemistry. 1991 May 7;30(18):4480–4486. doi: 10.1021/bi00232a015. [DOI] [PubMed] [Google Scholar]
- Rottenberg H. The measurement of membrane potential and deltapH in cells, organelles, and vesicles. Methods Enzymol. 1979;55:547–569. doi: 10.1016/0076-6879(79)55066-6. [DOI] [PubMed] [Google Scholar]
- Rouslin W. Mitochondrial complexes I, II, III, IV, and V in myocardial ischemia and autolysis. Am J Physiol. 1983 Jun;244(6):H743–H748. doi: 10.1152/ajpheart.1983.244.6.H743. [DOI] [PubMed] [Google Scholar]
- Rouslin W. Protonic inhibition of the mitochondrial oligomycin-sensitive adenosine 5'-triphosphatase in ischemic and autolyzing cardiac muscle. Possible mechanism for the mitigation of ATP hydrolysis under nonenergizing conditions. J Biol Chem. 1983 Aug 25;258(16):9657–9661. [PubMed] [Google Scholar]
- Schuler G., Hambrecht R., Schlierf G., Niebauer J., Hauer K., Neumann J., Hoberg E., Drinkmann A., Bacher F., Grunze M. Regular physical exercise and low-fat diet. Effects on progression of coronary artery disease. Circulation. 1992 Jul;86(1):1–11. doi: 10.1161/01.cir.86.1.1. [DOI] [PubMed] [Google Scholar]
- Shen A. C., Jennings R. B. Myocardial calcium and magnesium in acute ischemic injury. Am J Pathol. 1972 Jun;67(3):417–440. [PMC free article] [PubMed] [Google Scholar]
- Silverman H. S., Di Lisa F., Hui R. C., Miyata H., Sollott S. J., Hanford R. G., Lakatta E. G., Stern M. D. Regulation of intracellular free Mg2+ and contraction in single adult mammalian cardiac myocytes. Am J Physiol. 1994 Jan;266(1 Pt 1):C222–C233. doi: 10.1152/ajpcell.1994.266.1.C222. [DOI] [PubMed] [Google Scholar]
- Silverman H. S., Ninomiya M., Blank P. S., Hano O., Miyata H., Spurgeon H. A., Lakatta E. G., Stern M. D. A cellular mechanism for impaired posthypoxic relaxation in isolated cardiac myocytes. Altered myofilament relaxation kinetics at reoxygenation. Circ Res. 1991 Jul;69(1):196–208. doi: 10.1161/01.res.69.1.196. [DOI] [PubMed] [Google Scholar]
- Smiley S. T., Reers M., Mottola-Hartshorn C., Lin M., Chen A., Smith T. W., Steele G. D., Jr, Chen L. B. Intracellular heterogeneity in mitochondrial membrane potentials revealed by a J-aggregate-forming lipophilic cation JC-1. Proc Natl Acad Sci U S A. 1991 May 1;88(9):3671–3675. doi: 10.1073/pnas.88.9.3671. [DOI] [PMC free article] [PubMed] [Google Scholar]
- Snyder J. W., Pastorino J. G., Thomas A. P., Hoek J. B., Farber J. L. ATP synthase activity is required for fructose to protect cultured hepatocytes from the toxicity of cyanide. Am J Physiol. 1993 Mar;264(3 Pt 1):C709–C714. doi: 10.1152/ajpcell.1993.264.3.C709. [DOI] [PubMed] [Google Scholar]
- Spurgeon H. A., Stern M. D., Baartz G., Raffaeli S., Hansford R. G., Talo A., Lakatta E. G., Capogrossi M. C. Simultaneous measurement of Ca2+, contraction, and potential in cardiac myocytes. Am J Physiol. 1990 Feb;258(2 Pt 2):H574–H586. doi: 10.1152/ajpheart.1990.258.2.H574. [DOI] [PubMed] [Google Scholar]
- Stern M. D., Silverman H. S., Houser S. R., Josephson R. A., Capogrossi M. C., Nichols C. G., Lederer W. J., Lakatta E. G. Anoxic contractile failure in rat heart myocytes is caused by failure of intracellular calcium release due to alteration of the action potential. Proc Natl Acad Sci U S A. 1988 Sep;85(18):6954–6958. doi: 10.1073/pnas.85.18.6954. [DOI] [PMC free article] [PubMed] [Google Scholar]