Abstract
1. A fast inactivating transient K+ current (FK1) cloned from ferret ventricle and expressed in Xenopus oocytes was studied using the two-electrode voltage clamp technique. Removal of the NH2-terminal domain of FK1 (FK1 delta 2-146) removed fast inactivation consistent with previous findings in Kv1.4 channels. The NH2-terminal deletion mutation revealed a slow inactivation process, which matches the criteria for C-type inactivation described for Shaker B channels. 2. Inactivation of FK1 delta 2-146 at depolarized potentials was well described by a single exponential process with a voltage-insensitive time constant. In the range -90 to +20 mV, steady-state C-type inactivation was well described by a Boltzmann relationship that compares closely with inactivation measured in the presence of the NH2-terminus. These results suggest that C-type inactivation is coupled to activation. 3. The coupling of C-type inactivation to activation was assessed by mutation of the fourth positively charged residue (arginine 454) in the S4 voltage sensor to glutamine (R454Q). This mutation produced a hyperpolarizing shift in the inactivation relationship of both FK1 and FK1 delta 2-146 without altering the rate of inactivation of either clone. 4. The rates of recovery from inactivation are nearly identical in FK1 and FK1 delta 2-146. 5. To assess the mechanisms underlying recovery from inactivation the effects of elevated [K+]o and selective mutations in the extracellular pore and the S4 voltage sensor were compared in FK1 and FK1 delta 2-146. The similarity in recovery rates in response to these perturbations suggests that recovery from C-type inactivation governs the overall rate of recovery of inactivated channels for both FK1 and FK1 delta 2-146. 6. Analysis of the rate of recovery of FK1 channels for inactivating pulses of different durations (70-2000 ms) indicates that recovery rate is insensitive to the duration of the inactivating pulse.
Full text
PDF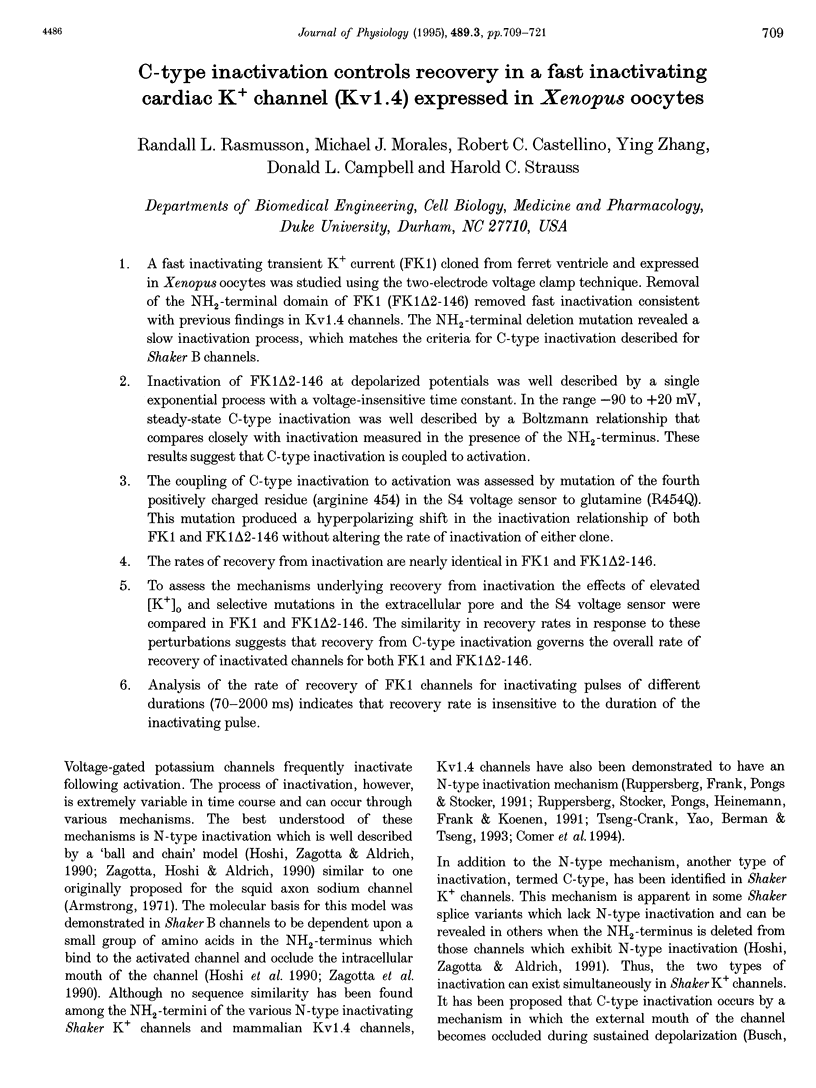
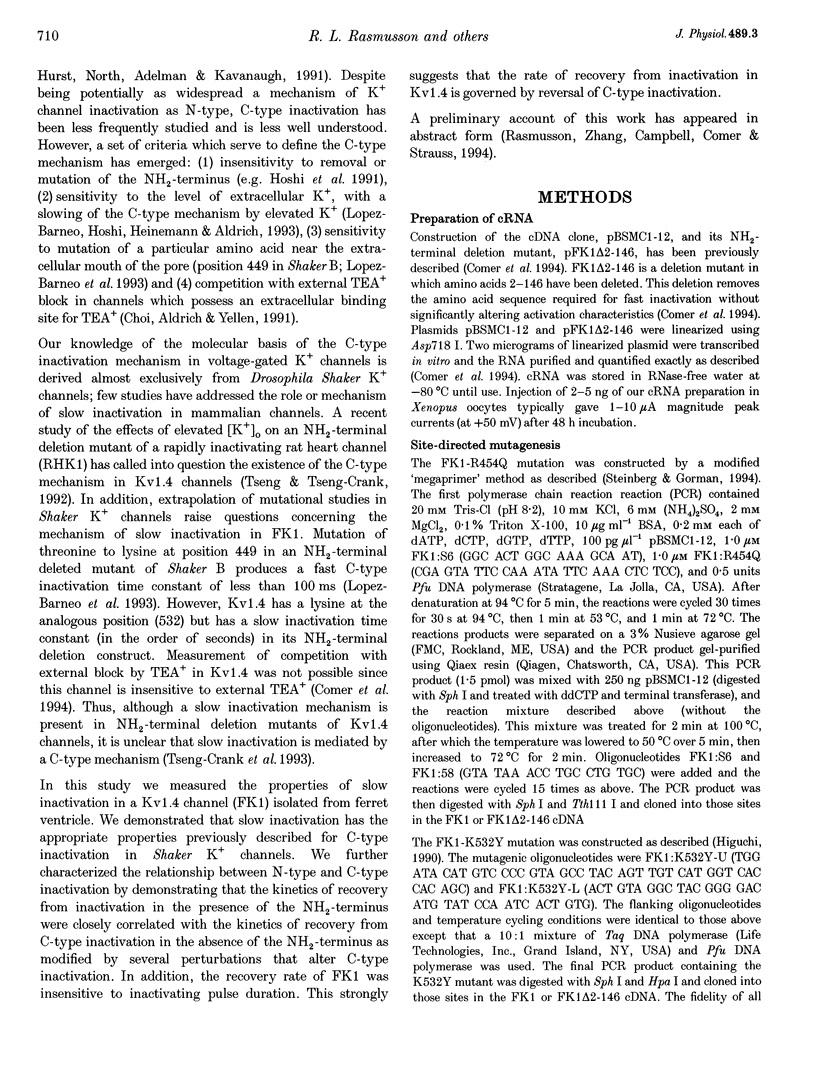
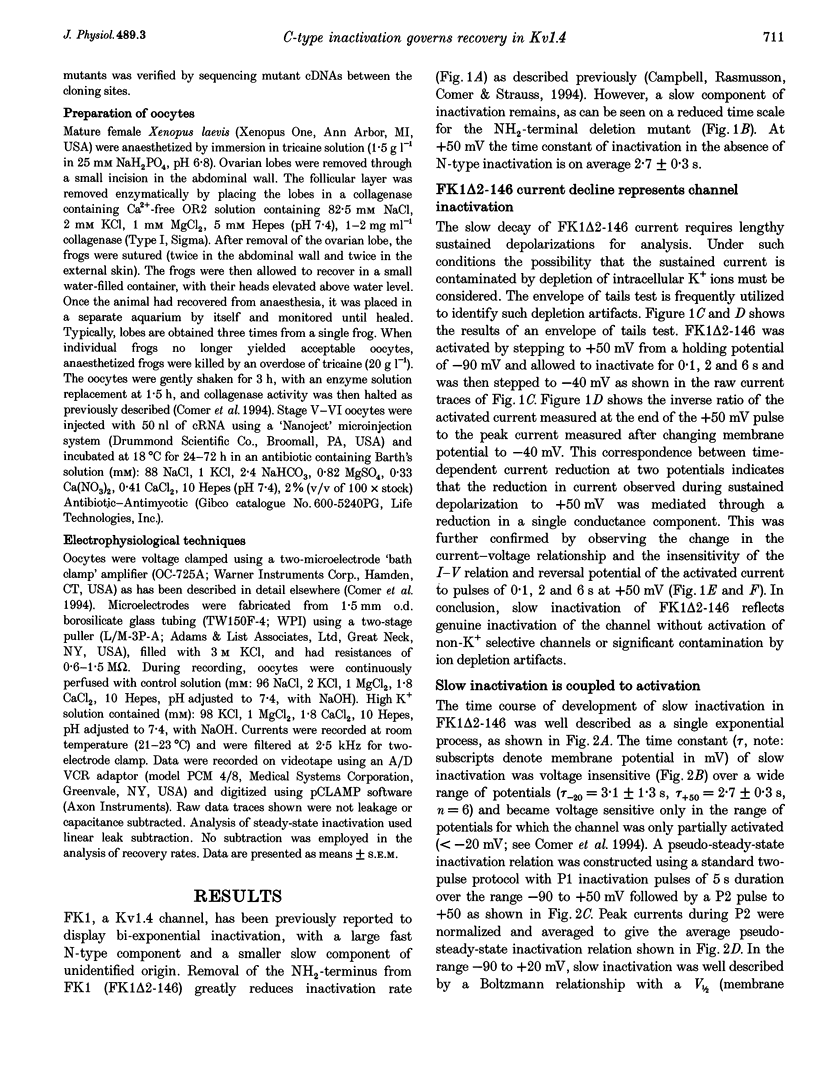
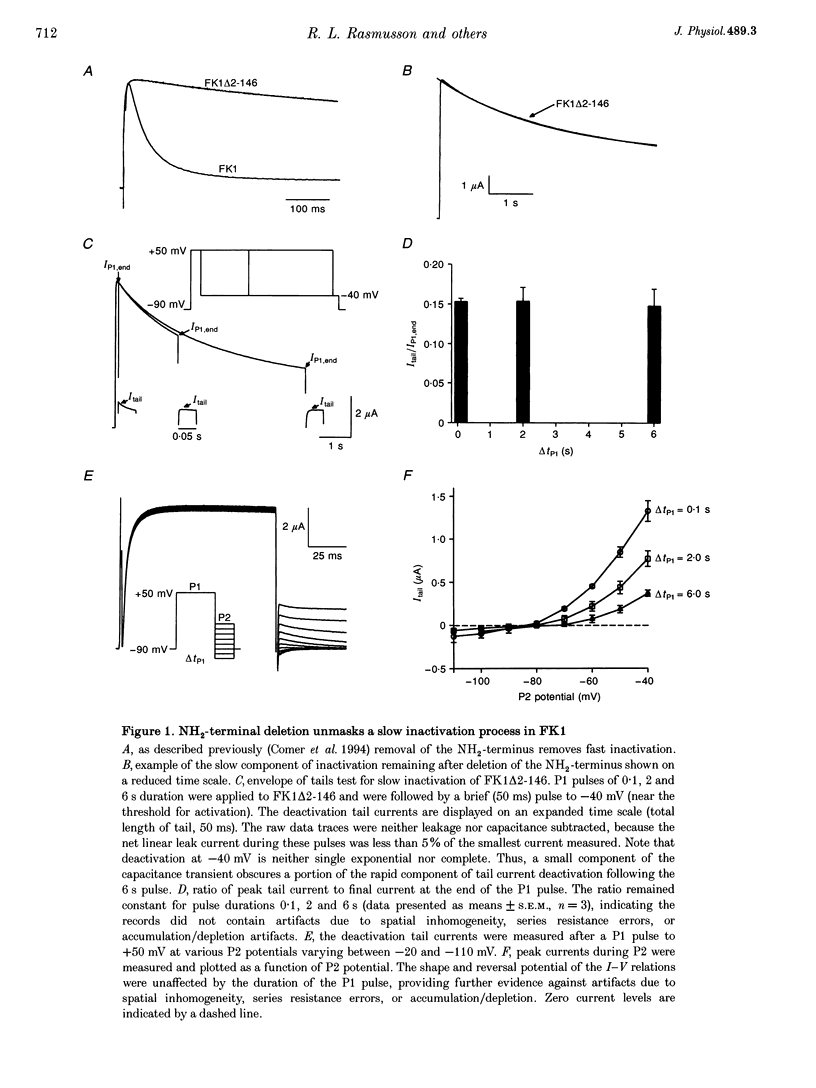
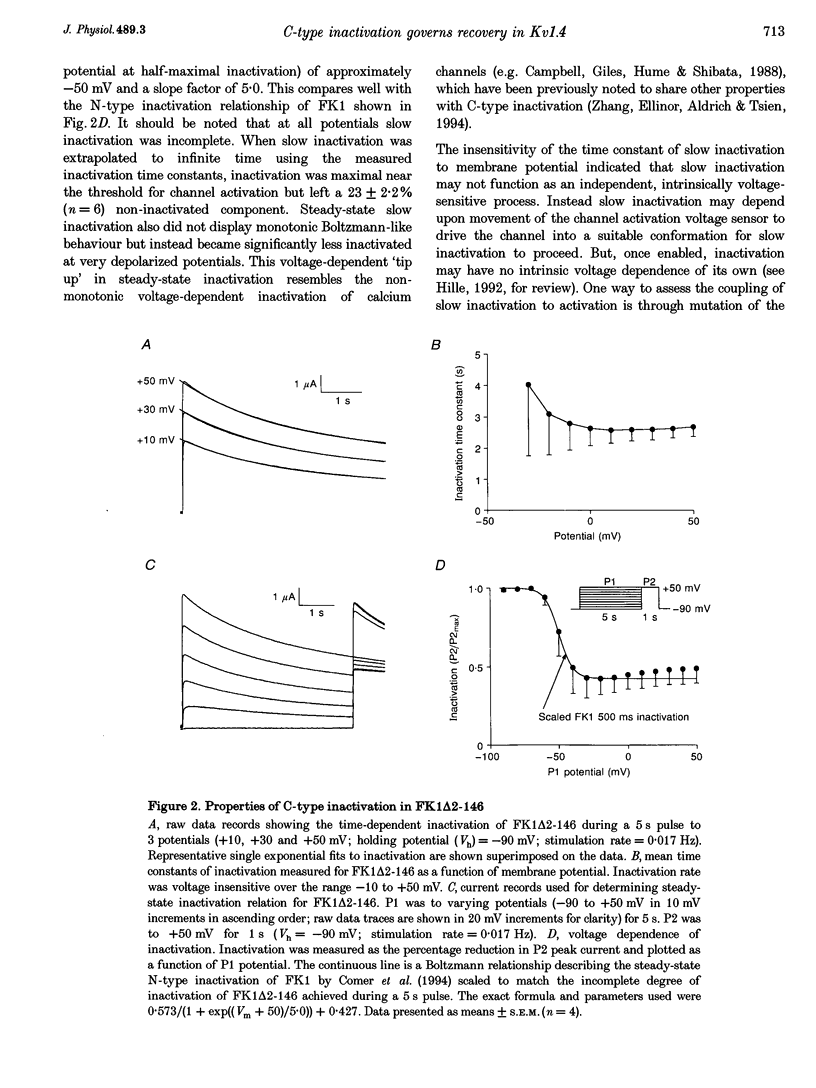
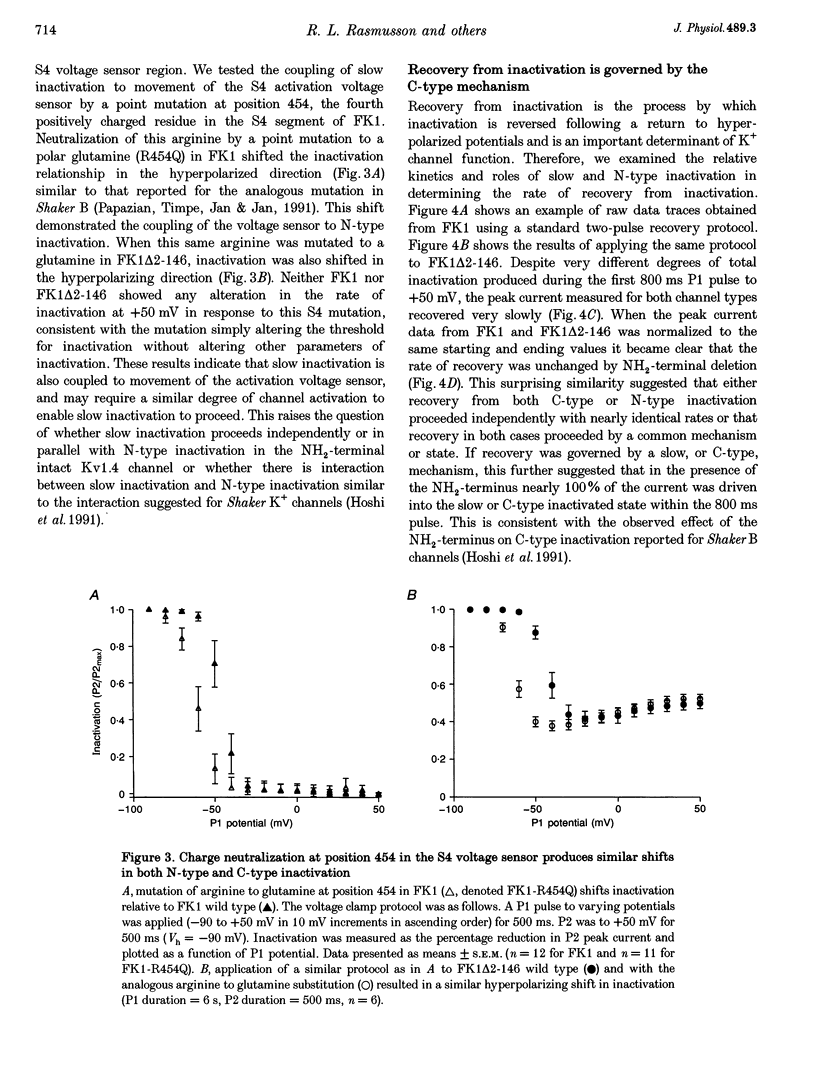
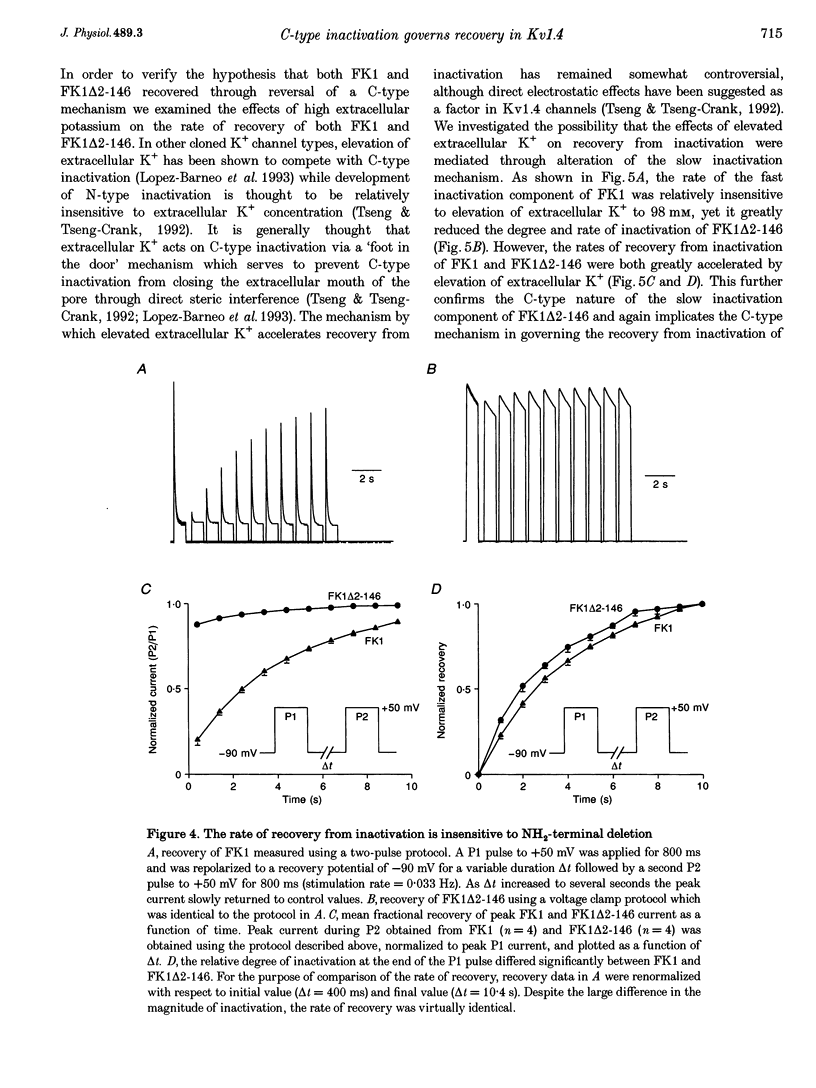
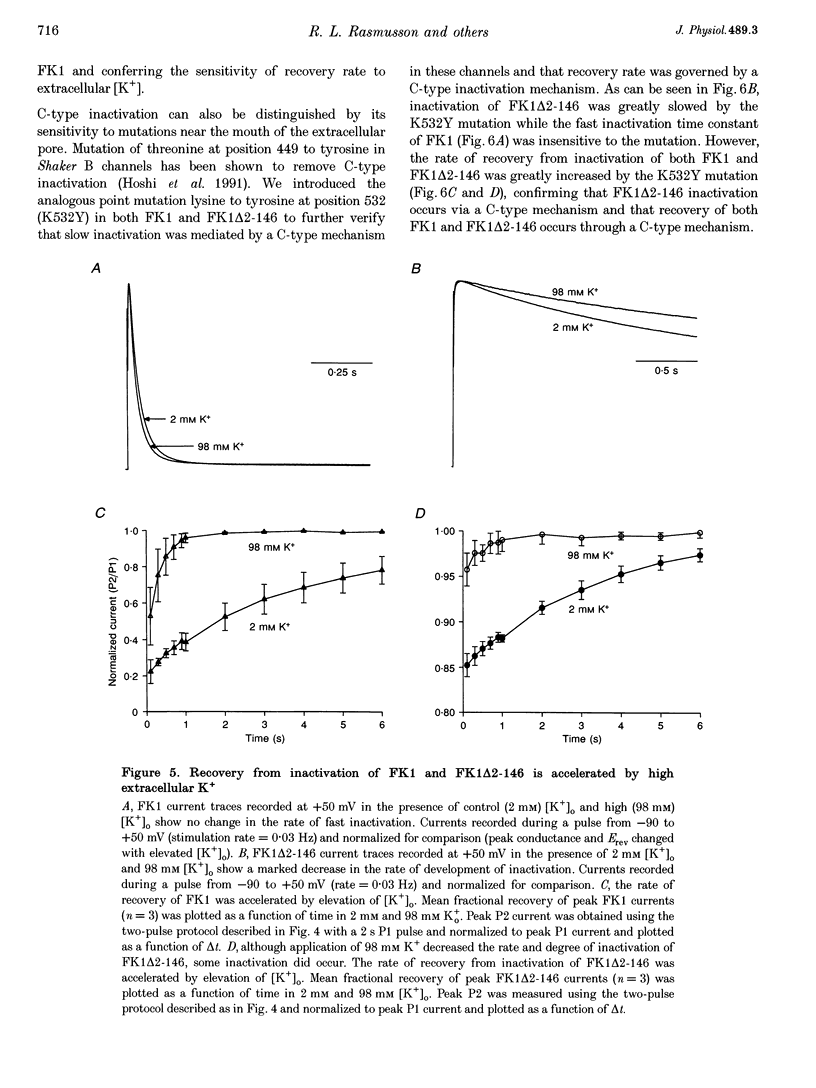
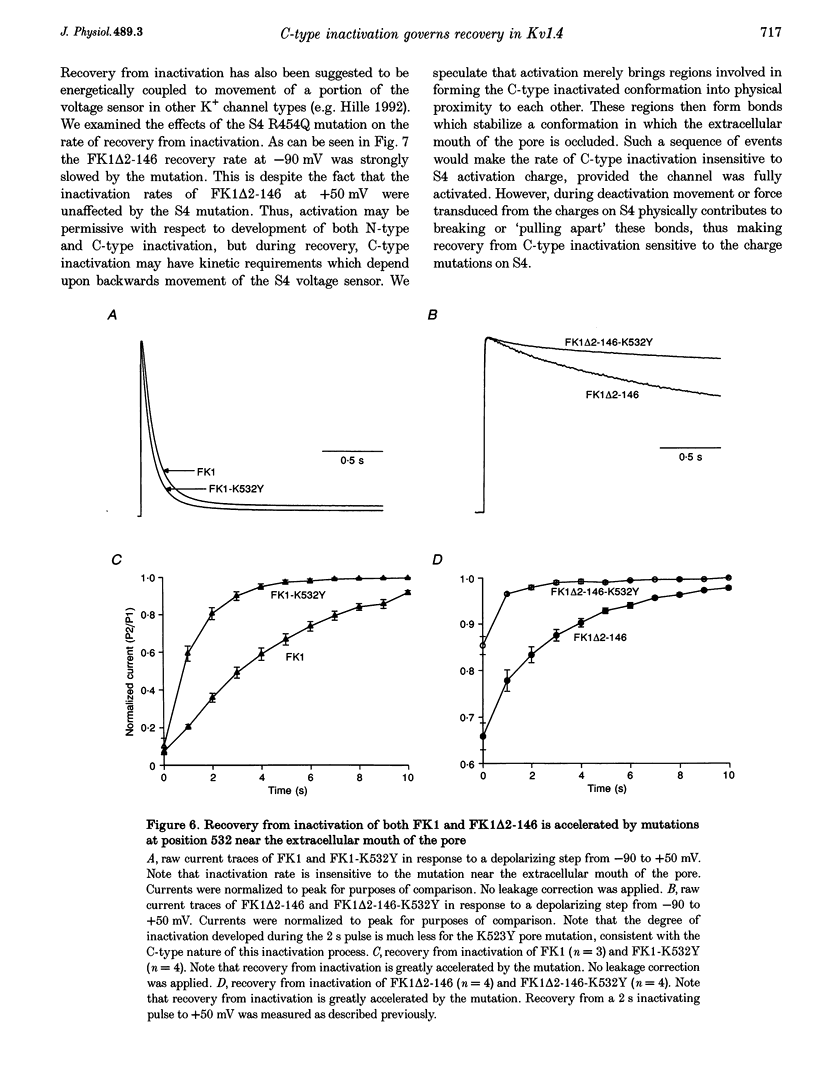
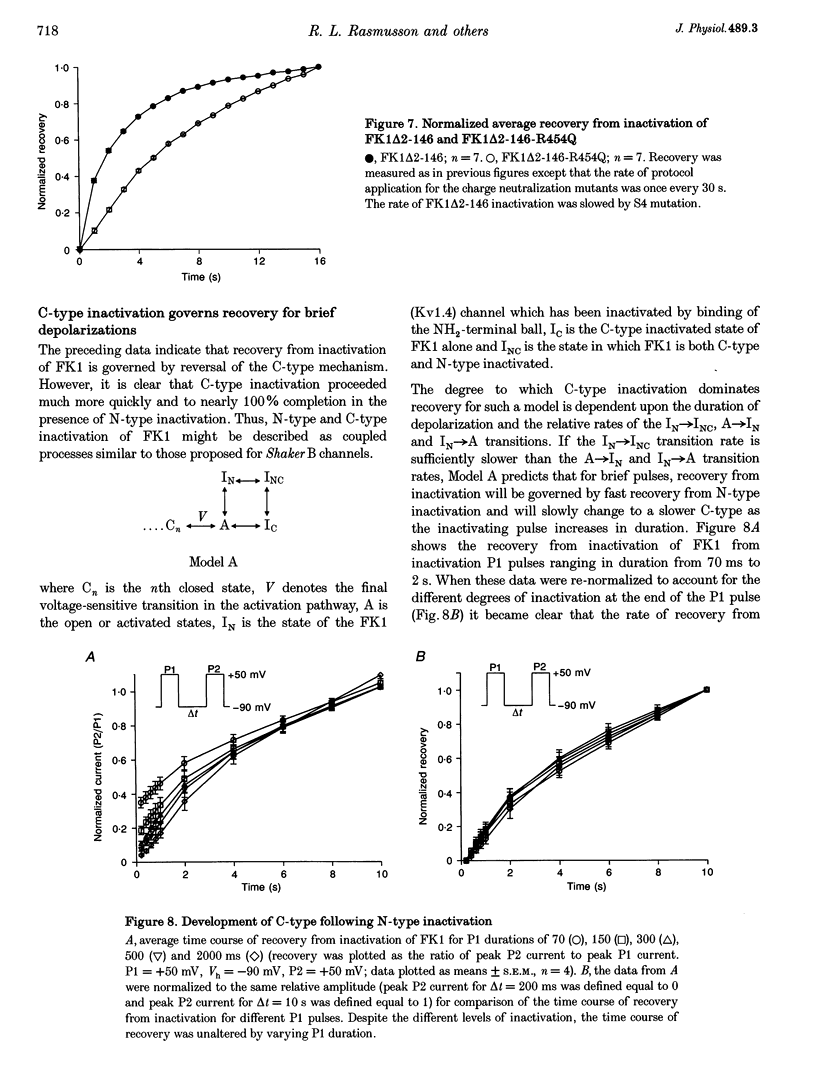
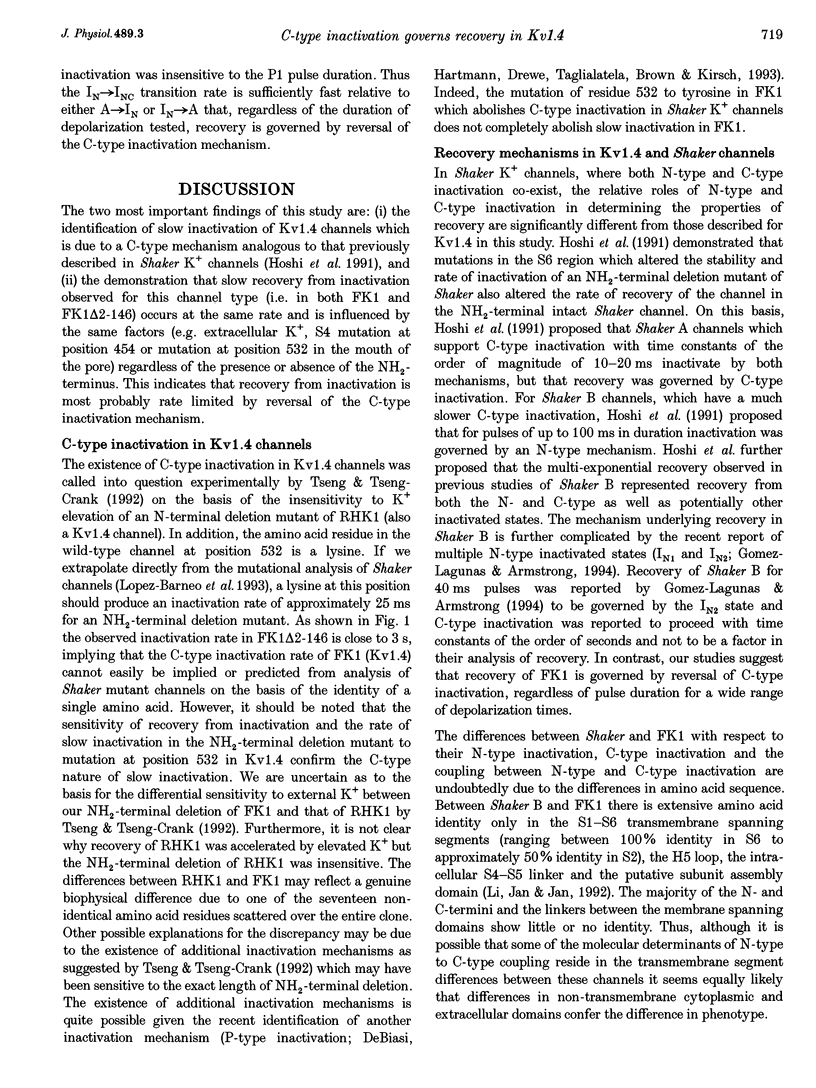
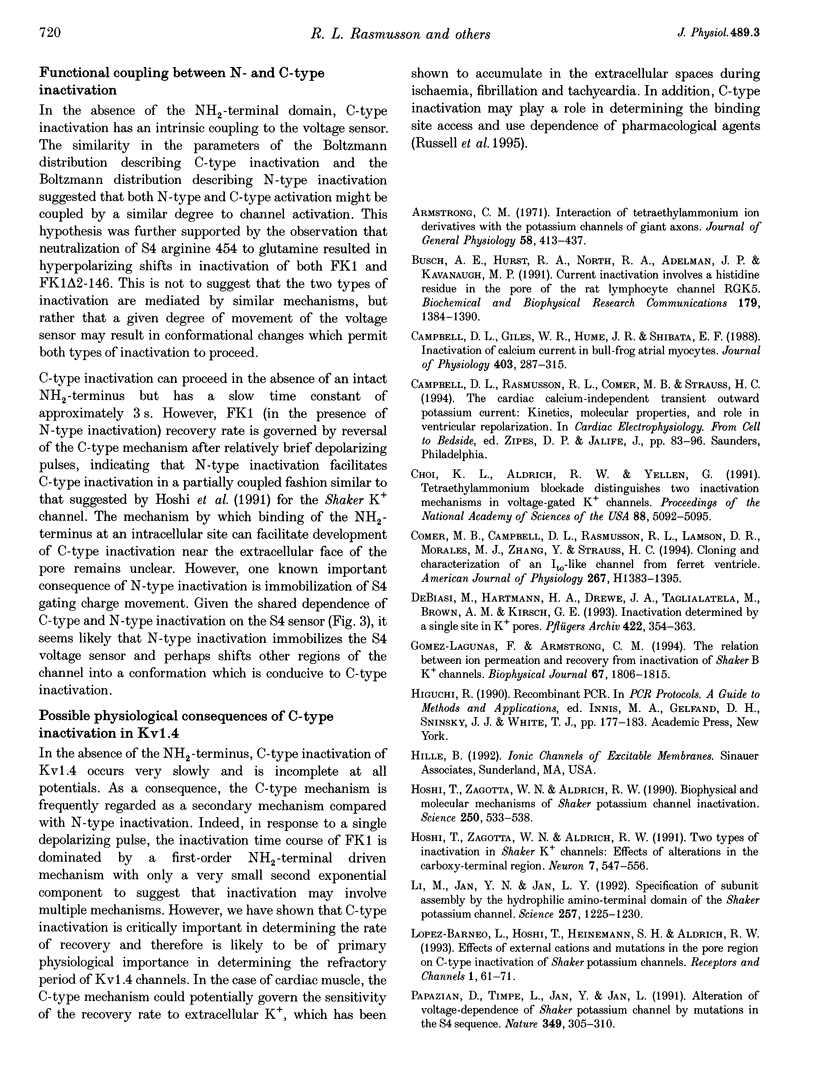
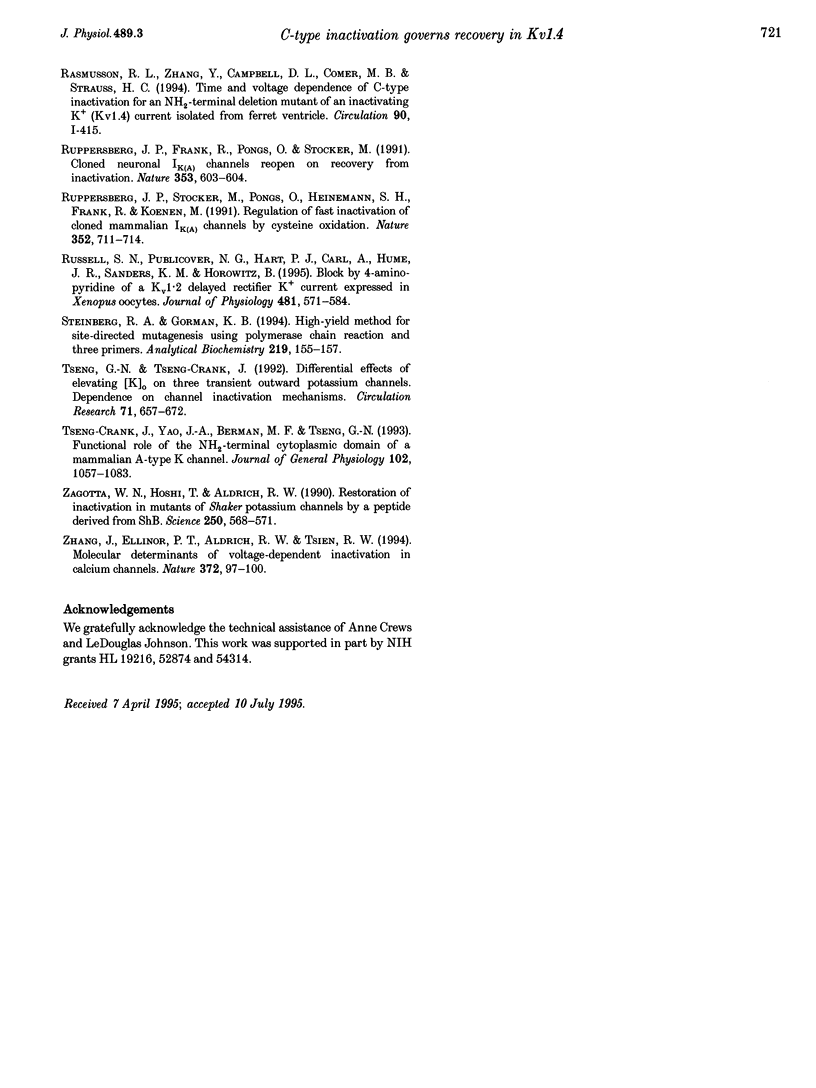
Selected References
These references are in PubMed. This may not be the complete list of references from this article.
- Armstrong C. M. Interaction of tetraethylammonium ion derivatives with the potassium channels of giant axons. J Gen Physiol. 1971 Oct;58(4):413–437. doi: 10.1085/jgp.58.4.413. [DOI] [PMC free article] [PubMed] [Google Scholar]
- Busch A. E., Hurst R. S., North R. A., Adelman J. P., Kavanaugh M. P. Current inactivation involves a histidine residue in the pore of the rat lymphocyte potassium channel RGK5. Biochem Biophys Res Commun. 1991 Sep 30;179(3):1384–1390. doi: 10.1016/0006-291x(91)91726-s. [DOI] [PubMed] [Google Scholar]
- Campbell D. L., Giles W. R., Hume J. R., Shibata E. F. Inactivation of calcium current in bull-frog atrial myocytes. J Physiol. 1988 Sep;403:287–315. doi: 10.1113/jphysiol.1988.sp017250. [DOI] [PMC free article] [PubMed] [Google Scholar]
- Choi K. L., Aldrich R. W., Yellen G. Tetraethylammonium blockade distinguishes two inactivation mechanisms in voltage-activated K+ channels. Proc Natl Acad Sci U S A. 1991 Jun 15;88(12):5092–5095. doi: 10.1073/pnas.88.12.5092. [DOI] [PMC free article] [PubMed] [Google Scholar]
- De Biasi M., Hartmann H. A., Drewe J. A., Taglialatela M., Brown A. M., Kirsch G. E. Inactivation determined by a single site in K+ pores. Pflugers Arch. 1993 Jan;422(4):354–363. doi: 10.1007/BF00374291. [DOI] [PubMed] [Google Scholar]
- Gómez-Lagunas F., Armstrong C. M. The relation between ion permeation and recovery from inactivation of ShakerB K+ channels. Biophys J. 1994 Nov;67(5):1806–1815. doi: 10.1016/S0006-3495(94)80662-9. [DOI] [PMC free article] [PubMed] [Google Scholar]
- Hoshi T., Zagotta W. N., Aldrich R. W. Biophysical and molecular mechanisms of Shaker potassium channel inactivation. Science. 1990 Oct 26;250(4980):533–538. doi: 10.1126/science.2122519. [DOI] [PubMed] [Google Scholar]
- Hoshi T., Zagotta W. N., Aldrich R. W. Two types of inactivation in Shaker K+ channels: effects of alterations in the carboxy-terminal region. Neuron. 1991 Oct;7(4):547–556. doi: 10.1016/0896-6273(91)90367-9. [DOI] [PubMed] [Google Scholar]
- Jones S. W. Ion channels. Not an open-and-shut case. Nature. 1991 Oct 17;353(6345):603–604. doi: 10.1038/353603a0. [DOI] [PubMed] [Google Scholar]
- Lenfant C. NHLBI funding policies. Enhancing stability, predictability, and cost control. Circulation. 1994 Jul;90(1):1–1. doi: 10.1161/01.cir.90.1.1. [DOI] [PubMed] [Google Scholar]
- López-Barneo J., Hoshi T., Heinemann S. H., Aldrich R. W. Effects of external cations and mutations in the pore region on C-type inactivation of Shaker potassium channels. Receptors Channels. 1993;1(1):61–71. [PubMed] [Google Scholar]
- Papazian D. M., Timpe L. C., Jan Y. N., Jan L. Y. Alteration of voltage-dependence of Shaker potassium channel by mutations in the S4 sequence. Nature. 1991 Jan 24;349(6307):305–310. doi: 10.1038/349305a0. [DOI] [PubMed] [Google Scholar]
- Ruppersberg J. P., Stocker M., Pongs O., Heinemann S. H., Frank R., Koenen M. Regulation of fast inactivation of cloned mammalian IK(A) channels by cysteine oxidation. Nature. 1991 Aug 22;352(6337):711–714. doi: 10.1038/352711a0. [DOI] [PubMed] [Google Scholar]
- Russell S. N., Publicover N. G., Hart P. J., Carl A., Hume J. R., Sanders K. M., Horowitz B. Block by 4-aminopyridine of a Kv1.2 delayed rectifier K+ current expressed in Xenopus oocytes. J Physiol. 1994 Dec 15;481(Pt 3):571–584. doi: 10.1113/jphysiol.1994.sp020464. [DOI] [PMC free article] [PubMed] [Google Scholar]
- Steinberg R. A., Gorman K. B. A high-yield method for site-directed mutagenesis using polymerase chain reaction and three primers. Anal Biochem. 1994 May 15;219(1):155–157. doi: 10.1006/abio.1994.1246. [DOI] [PubMed] [Google Scholar]
- Tseng-Crank J., Yao J. A., Berman M. F., Tseng G. N. Functional role of the NH2-terminal cytoplasmic domain of a mammalian A-type K channel. J Gen Physiol. 1993 Dec;102(6):1057–1083. doi: 10.1085/jgp.102.6.1057. [DOI] [PMC free article] [PubMed] [Google Scholar]
- Tseng G. N., Tseng-Crank J. Differential effects of elevating [K]o on three transient outward potassium channels. Dependence on channel inactivation mechanisms. Circ Res. 1992 Sep;71(3):657–672. doi: 10.1161/01.res.71.3.657. [DOI] [PubMed] [Google Scholar]
- Zagotta W. N., Hoshi T., Aldrich R. W. Restoration of inactivation in mutants of Shaker potassium channels by a peptide derived from ShB. Science. 1990 Oct 26;250(4980):568–571. doi: 10.1126/science.2122520. [DOI] [PubMed] [Google Scholar]
- Zhang J. F., Ellinor P. T., Aldrich R. W., Tsien R. W. Molecular determinants of voltage-dependent inactivation in calcium channels. Nature. 1994 Nov 3;372(6501):97–100. doi: 10.1038/372097a0. [DOI] [PubMed] [Google Scholar]