Abstract
1. Loose-patch voltage-clamp recordings were made from rat and mouse skeletal muscle fibres denervated for up to 6 weeks. Innervated muscles possessed a Na+ current density of 107 +/- 3.3 mA cm-2 in endplate membrane, and 6.3 +/- 0.6 mA cm-2 in extrajunctional membrane. This high concentration of Na+ channels at the endplate was gradually reduced following denervation. After 6 weeks of denervation, the endplate Na+ channel concentration was reduced by 40-50%, and the density of Na+ channels in extrajunctional membrane was increased by about 30%. 2. The tetrodotoxin (TTX)-resistant form of the Na+ channel appeared after 3 days of denervation and comprised approximately 43% of the endplate Na+ channels 5-6 days after denervation. Subsequently, TTX-resistant Na+ channels were reduced in density to approximately 25% of the postjunctional Na+ channels and remained at this level up to 6 weeks after denervation. 3. RNase protection analysis showed that mRNA encoding the TTX-resistant Na+ channel was virtually absent in innervated muscle, rose > 50-fold after 3 days of denervation, then decreased by 95% 6 weeks after denervation. The density of TTX-resistant Na+ channels correlated qualitatively with changes in mRNA levels. 4. These results suggest that the density of Na+ channels at neuromuscular junctions is maintained by two mechanisms, one influenced by the nerve terminal and the other independent of innervation.
Full text
PDF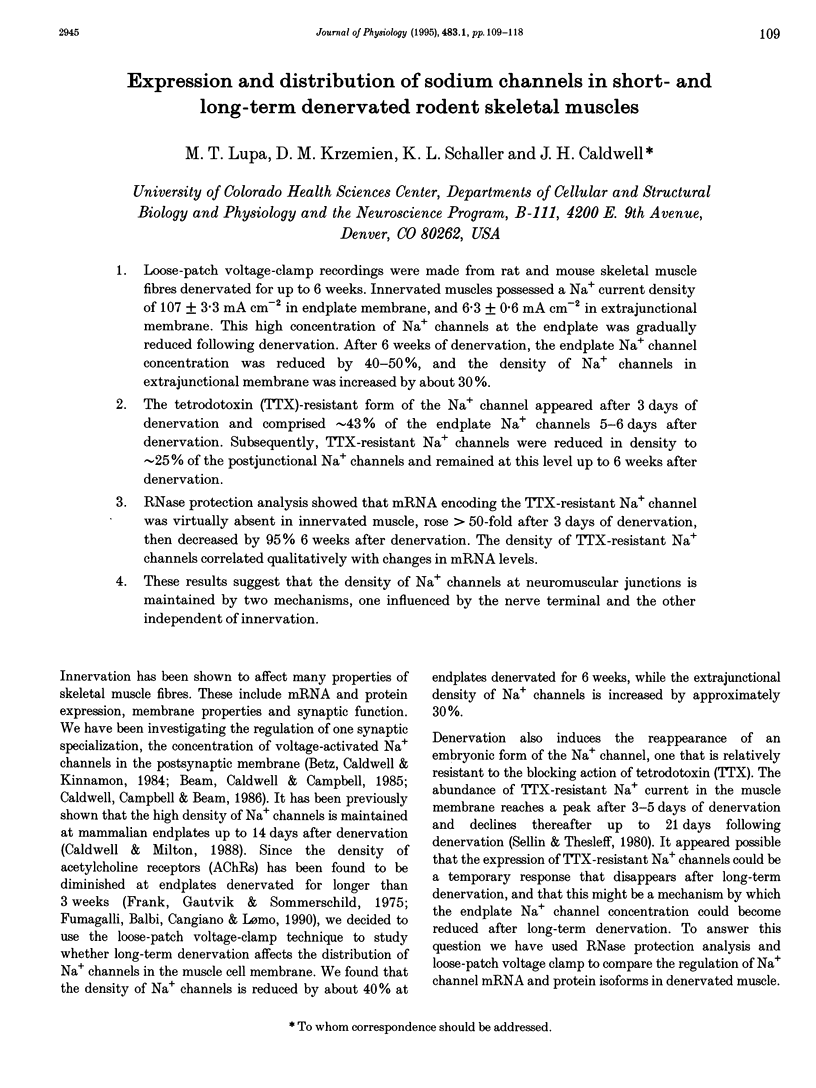
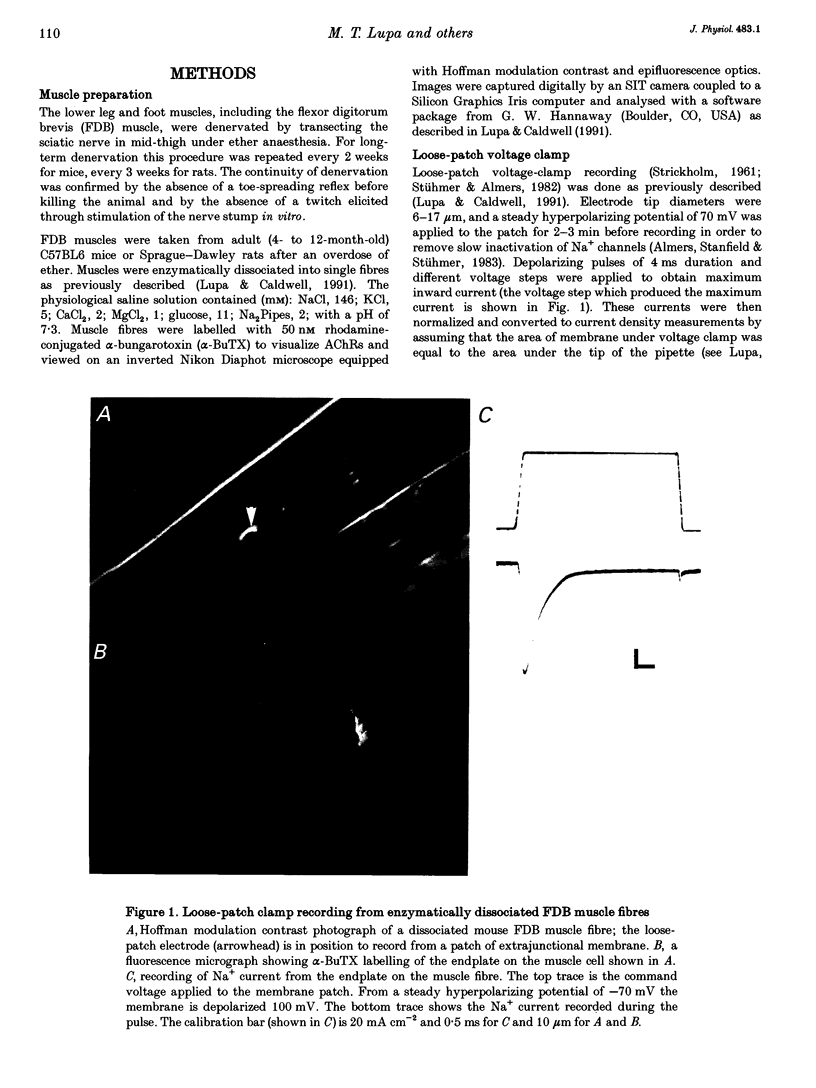
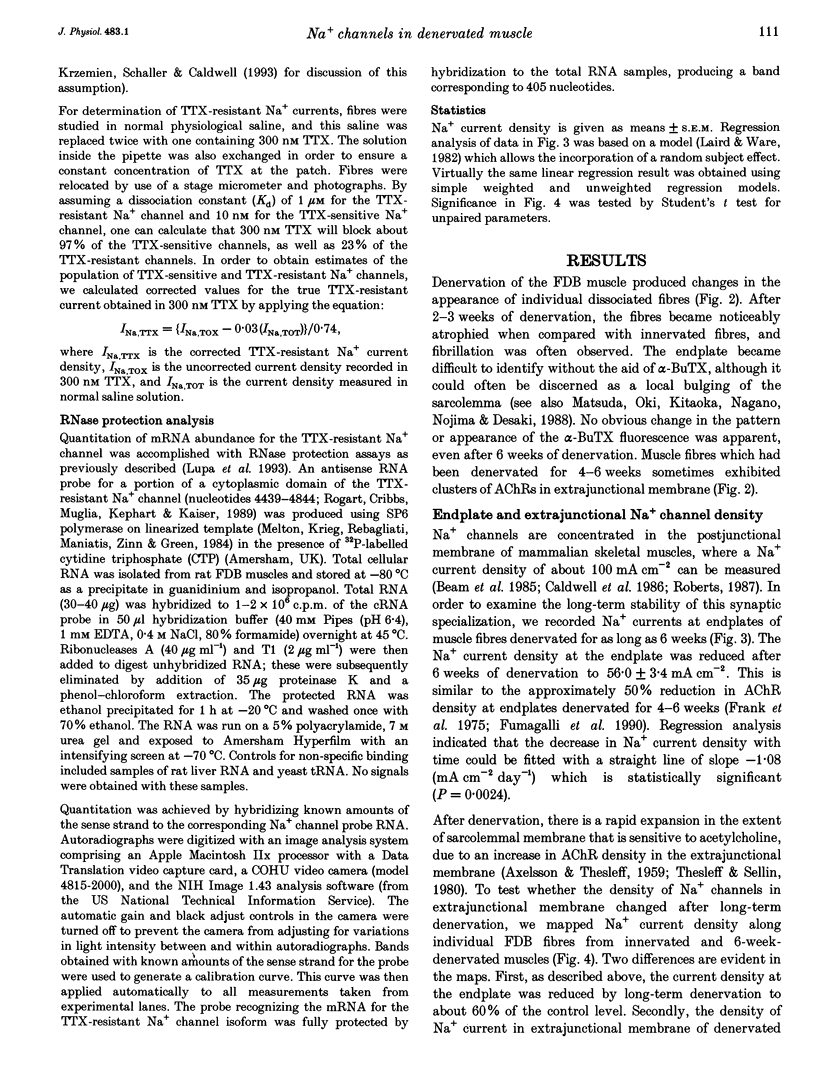
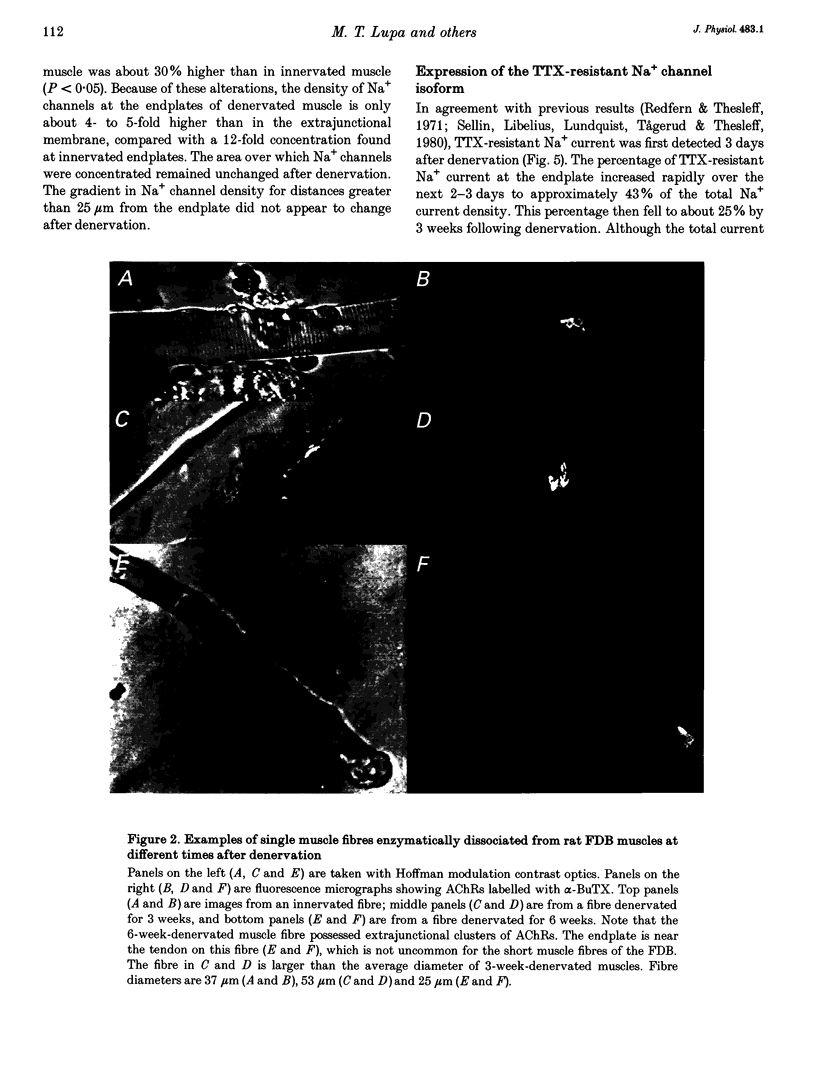
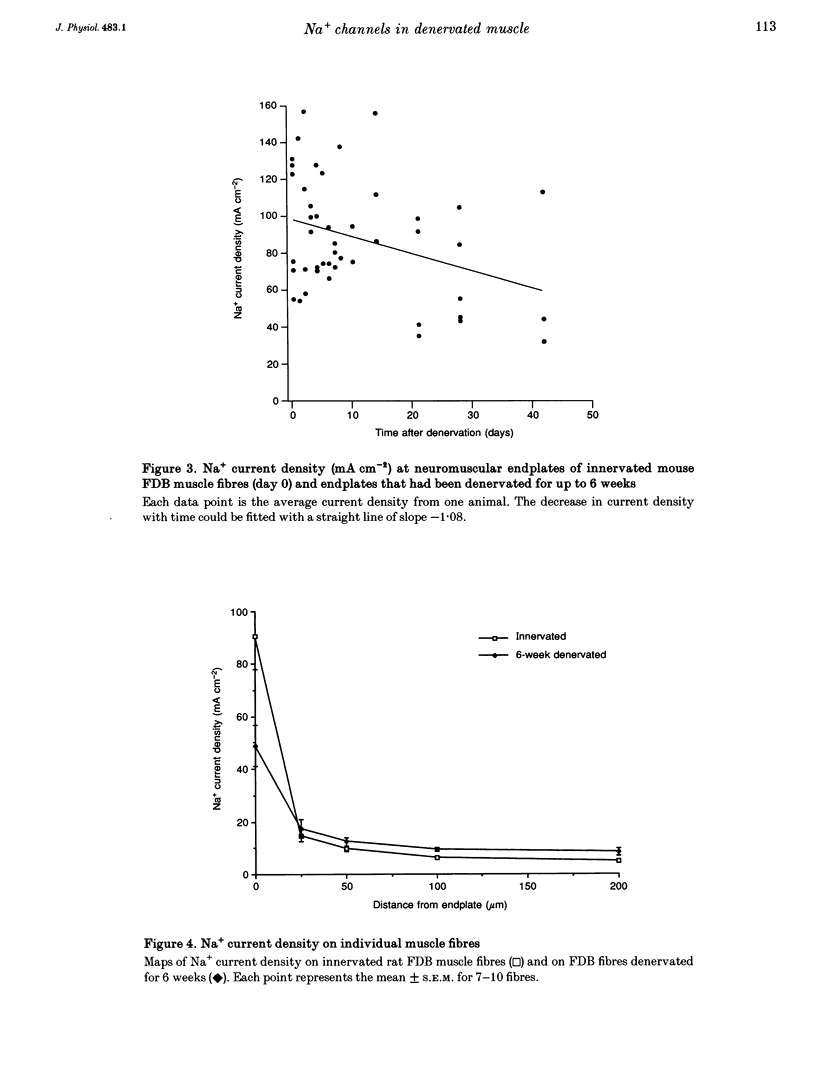
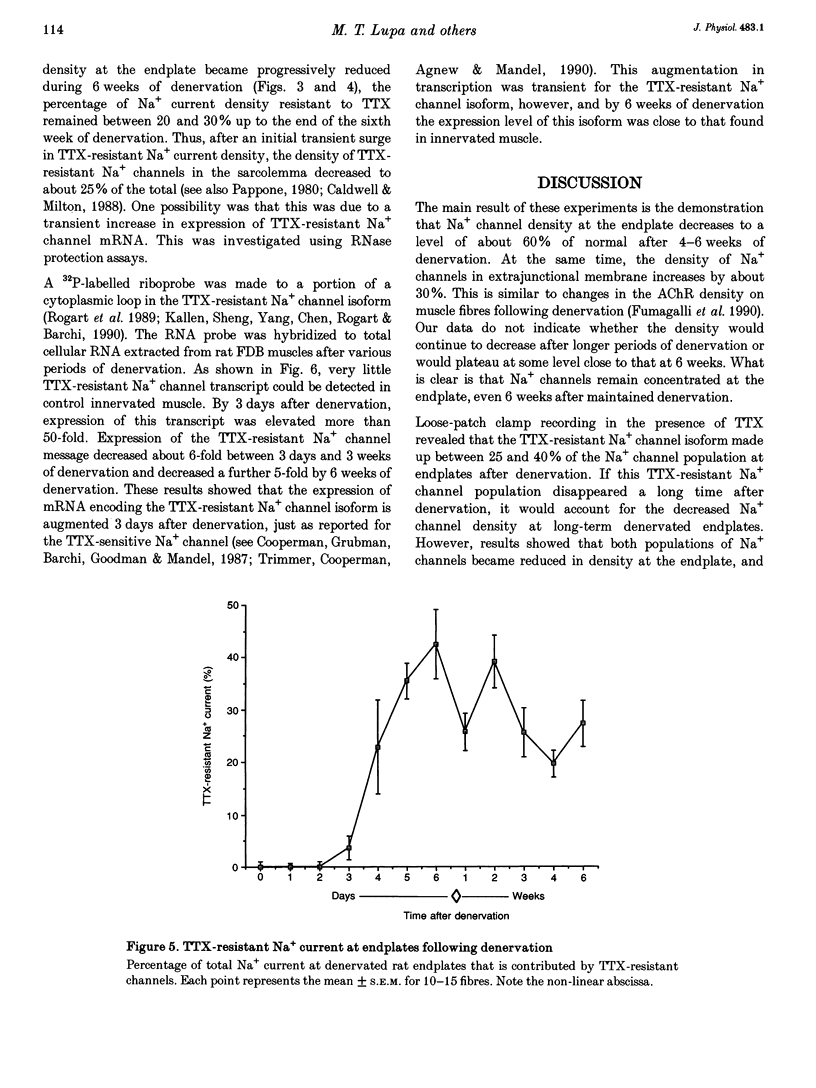
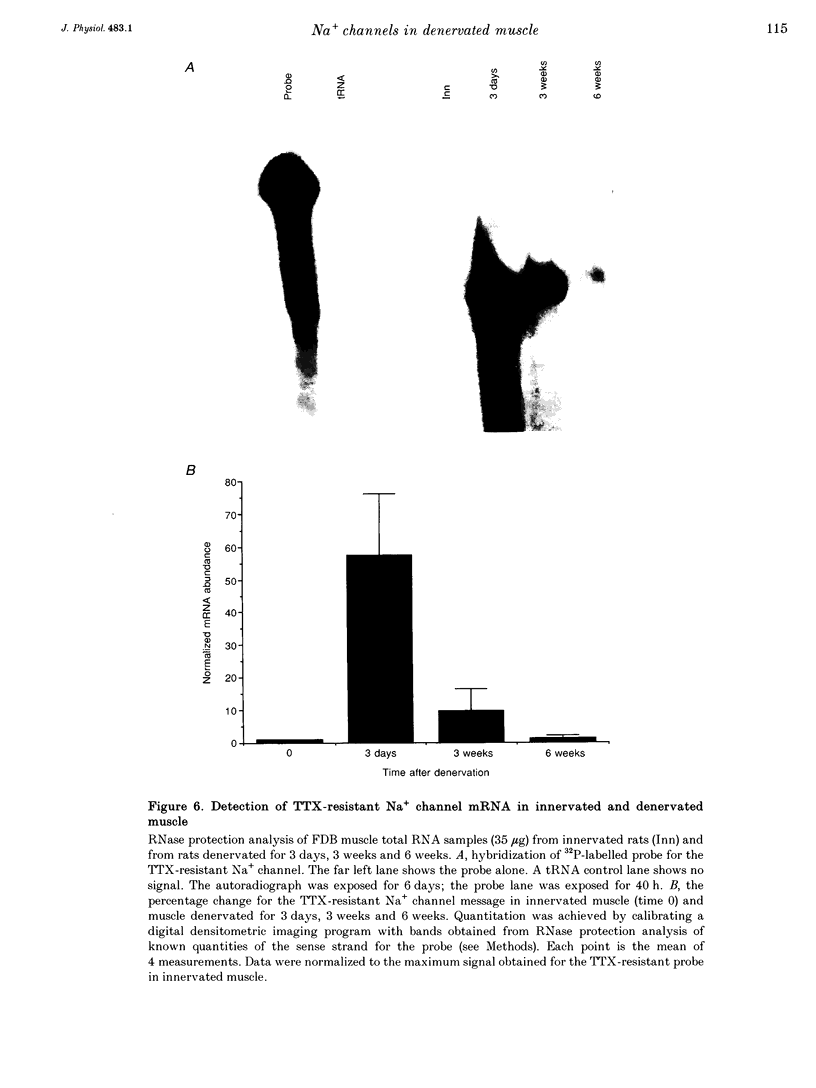
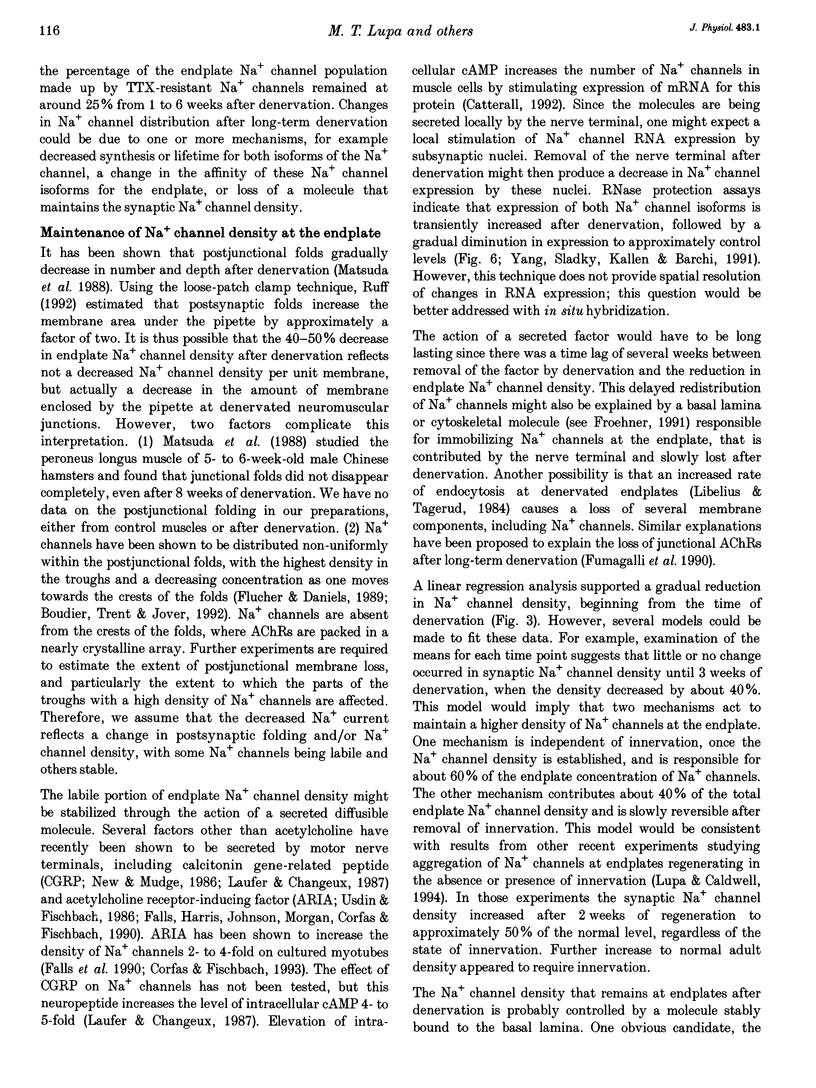
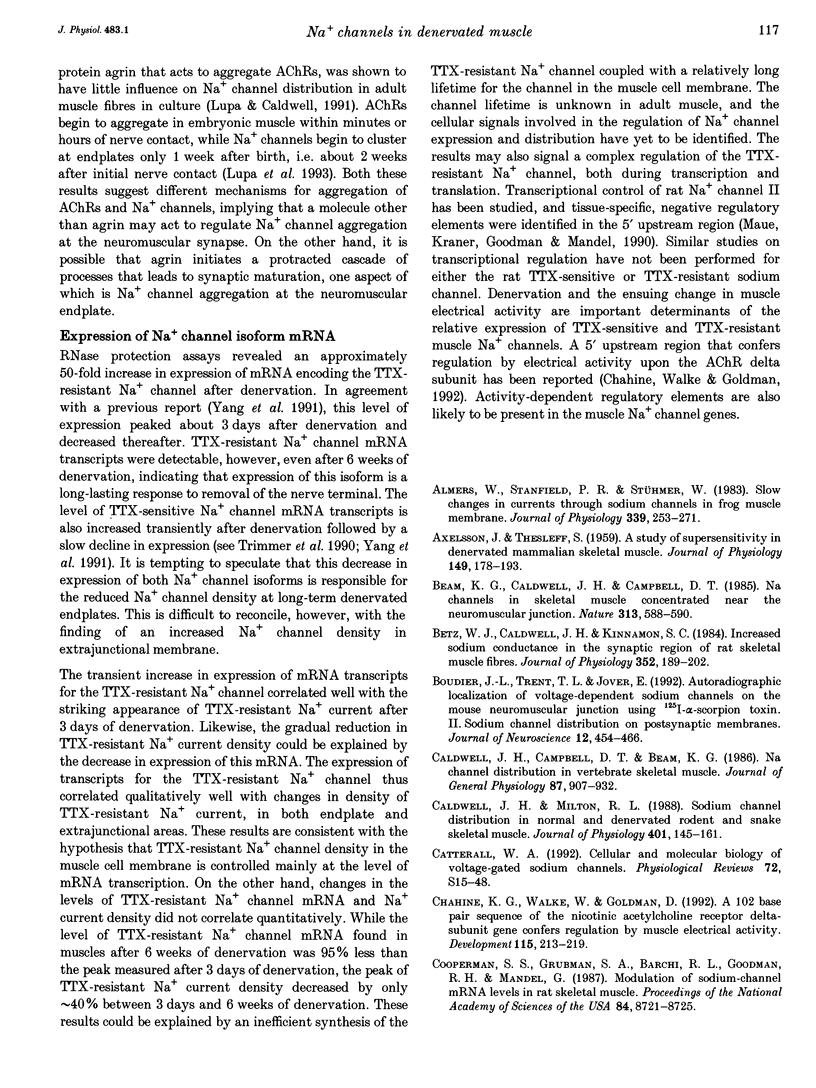
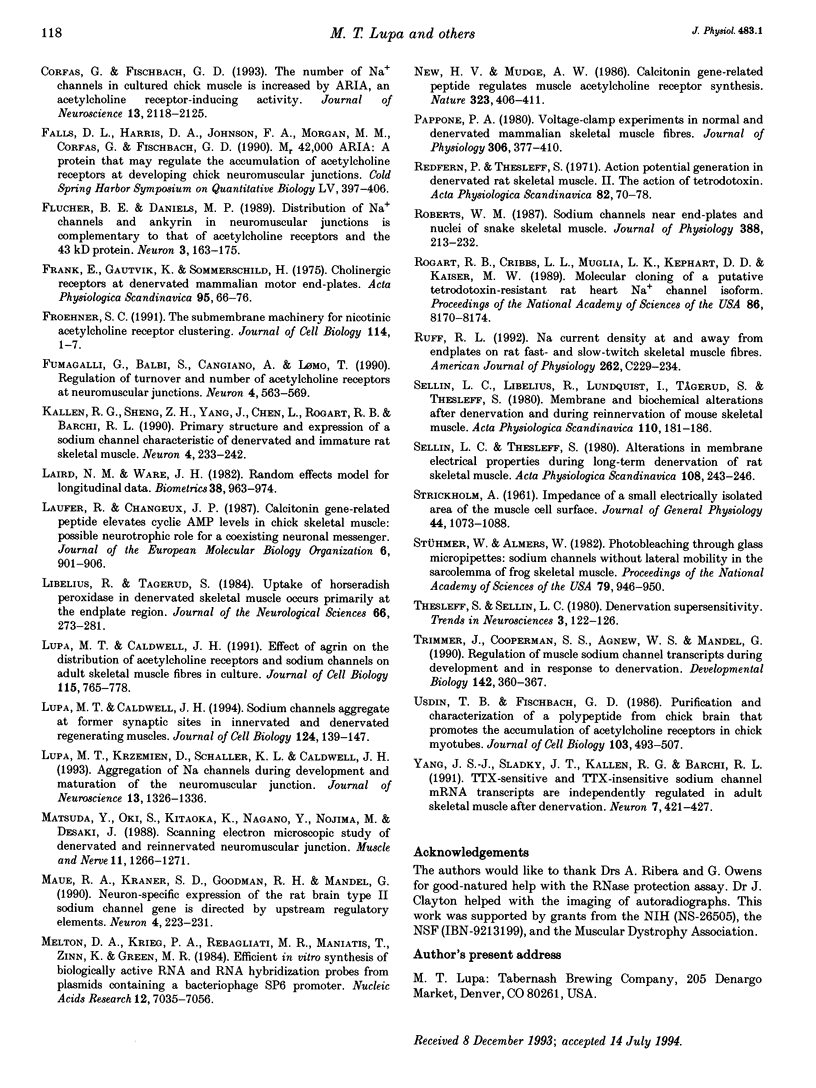
Images in this article
Selected References
These references are in PubMed. This may not be the complete list of references from this article.
- AXELSSON J., THESLEFF S. A study of supersensitivity in denervated mammalian skeletal muscle. J Physiol. 1959 Jun 23;147(1):178–193. doi: 10.1113/jphysiol.1959.sp006233. [DOI] [PMC free article] [PubMed] [Google Scholar]
- Almers W., Stanfield P. R., Stühmer W. Slow changes in currents through sodium channels in frog muscle membrane. J Physiol. 1983 Jun;339:253–271. doi: 10.1113/jphysiol.1983.sp014715. [DOI] [PMC free article] [PubMed] [Google Scholar]
- Beam K. G., Caldwell J. H., Campbell D. T. Na channels in skeletal muscle concentrated near the neuromuscular junction. Nature. 1985 Feb 14;313(6003):588–590. doi: 10.1038/313588a0. [DOI] [PubMed] [Google Scholar]
- Betz W. J., Caldwell J. H., Kinnamon S. C. Increased sodium conductance in the synaptic region of rat skeletal muscle fibres. J Physiol. 1984 Jul;352:189–202. doi: 10.1113/jphysiol.1984.sp015286. [DOI] [PMC free article] [PubMed] [Google Scholar]
- Boudier J. L., Le Treut T., Jover E. Autoradiographic localization of voltage-dependent sodium channels on the mouse neuromuscular junction using 125I-alpha scorpion toxin. II. Sodium distribution on postsynaptic membranes. J Neurosci. 1992 Feb;12(2):454–466. doi: 10.1523/JNEUROSCI.12-02-00454.1992. [DOI] [PMC free article] [PubMed] [Google Scholar]
- Caldwell J. H., Campbell D. T., Beam K. G. Na channel distribution in vertebrate skeletal muscle. J Gen Physiol. 1986 Jun;87(6):907–932. doi: 10.1085/jgp.87.6.907. [DOI] [PMC free article] [PubMed] [Google Scholar]
- Caldwell J. H., Milton R. L. Sodium channel distribution in normal and denervated rodent and snake skeletal muscle. J Physiol. 1988 Jul;401:145–161. doi: 10.1113/jphysiol.1988.sp017155. [DOI] [PMC free article] [PubMed] [Google Scholar]
- Catterall W. A. Cellular and molecular biology of voltage-gated sodium channels. Physiol Rev. 1992 Oct;72(4 Suppl):S15–S48. doi: 10.1152/physrev.1992.72.suppl_4.S15. [DOI] [PubMed] [Google Scholar]
- Chahine K. G., Walke W., Goldman D. A 102 base pair sequence of the nicotinic acetylcholine receptor delta-subunit gene confers regulation by muscle electrical activity. Development. 1992 May;115(1):213–219. doi: 10.1242/dev.115.1.213. [DOI] [PubMed] [Google Scholar]
- Cooperman S. S., Grubman S. A., Barchi R. L., Goodman R. H., Mandel G. Modulation of sodium-channel mRNA levels in rat skeletal muscle. Proc Natl Acad Sci U S A. 1987 Dec;84(23):8721–8725. doi: 10.1073/pnas.84.23.8721. [DOI] [PMC free article] [PubMed] [Google Scholar]
- Corfas G., Fischbach G. D. The number of Na+ channels in cultured chick muscle is increased by ARIA, an acetylcholine receptor-inducing activity. J Neurosci. 1993 May;13(5):2118–2125. doi: 10.1523/JNEUROSCI.13-05-02118.1993. [DOI] [PMC free article] [PubMed] [Google Scholar]
- Falls D. L., Harris D. A., Johnson F. A., Morgan M. M., Corfas G., Fischbach G. D. Mr 42,000 ARIA: a protein that may regulate the accumulation of acetylcholine receptors at developing chick neuromuscular junctions. Cold Spring Harb Symp Quant Biol. 1990;55:397–406. doi: 10.1101/sqb.1990.055.01.040. [DOI] [PubMed] [Google Scholar]
- Flucher B. E., Daniels M. P. Distribution of Na+ channels and ankyrin in neuromuscular junctions is complementary to that of acetylcholine receptors and the 43 kd protein. Neuron. 1989 Aug;3(2):163–175. doi: 10.1016/0896-6273(89)90029-9. [DOI] [PubMed] [Google Scholar]
- Frank E., Gautvik K., Sommerschild H. Cholinergic receptors at denervated mammalian motor end-plates. Acta Physiol Scand. 1975 Sep;95(1):66–76. doi: 10.1111/j.1748-1716.1975.tb10026.x. [DOI] [PubMed] [Google Scholar]
- Froehner S. C. The submembrane machinery for nicotinic acetylcholine receptor clustering. J Cell Biol. 1991 Jul;114(1):1–7. doi: 10.1083/jcb.114.1.1. [DOI] [PMC free article] [PubMed] [Google Scholar]
- Fumagalli G., Balbi S., Cangiano A., Lømo T. Regulation of turnover and number of acetylcholine receptors at neuromuscular junctions. Neuron. 1990 Apr;4(4):563–569. doi: 10.1016/0896-6273(90)90114-u. [DOI] [PubMed] [Google Scholar]
- Kallen R. G., Sheng Z. H., Yang J., Chen L. Q., Rogart R. B., Barchi R. L. Primary structure and expression of a sodium channel characteristic of denervated and immature rat skeletal muscle. Neuron. 1990 Feb;4(2):233–242. doi: 10.1016/0896-6273(90)90098-z. [DOI] [PubMed] [Google Scholar]
- Laird N. M., Ware J. H. Random-effects models for longitudinal data. Biometrics. 1982 Dec;38(4):963–974. [PubMed] [Google Scholar]
- Laufer R., Changeux J. P. Calcitonin gene-related peptide elevates cyclic AMP levels in chick skeletal muscle: possible neurotrophic role for a coexisting neuronal messenger. EMBO J. 1987 Apr;6(4):901–906. doi: 10.1002/j.1460-2075.1987.tb04836.x. [DOI] [PMC free article] [PubMed] [Google Scholar]
- Libelius R., Tågerud S. Uptake of horseradish peroxidase in denervated skeletal muscle occurs primarily at the endplate region. J Neurol Sci. 1984 Nov-Dec;66(2-3):273–281. doi: 10.1016/0022-510x(84)90016-9. [DOI] [PubMed] [Google Scholar]
- Lupa M. T., Caldwell J. H. Effect of agrin on the distribution of acetylcholine receptors and sodium channels on adult skeletal muscle fibers in culture. J Cell Biol. 1991 Nov;115(3):765–778. doi: 10.1083/jcb.115.3.765. [DOI] [PMC free article] [PubMed] [Google Scholar]
- Lupa M. T., Caldwell J. H. Sodium channels aggregate at former synaptic sites in innervated and denervated regenerating muscles. J Cell Biol. 1994 Jan;124(1-2):139–147. doi: 10.1083/jcb.124.1.139. [DOI] [PMC free article] [PubMed] [Google Scholar]
- Lupa M. T., Krzemien D. M., Schaller K. L., Caldwell J. H. Aggregation of sodium channels during development and maturation of the neuromuscular junction. J Neurosci. 1993 Mar;13(3):1326–1336. doi: 10.1523/JNEUROSCI.13-03-01326.1993. [DOI] [PMC free article] [PubMed] [Google Scholar]
- Matsuda Y., Oki S., Kitaoka K., Nagano Y., Nojima M., Desaki J. Scanning electron microscopic study of denervated and reinnervated neuromuscular junction. Muscle Nerve. 1988 Dec;11(12):1266–1271. doi: 10.1002/mus.880111211. [DOI] [PubMed] [Google Scholar]
- Maue R. A., Kraner S. D., Goodman R. H., Mandel G. Neuron-specific expression of the rat brain type II sodium channel gene is directed by upstream regulatory elements. Neuron. 1990 Feb;4(2):223–231. doi: 10.1016/0896-6273(90)90097-y. [DOI] [PubMed] [Google Scholar]
- Melton D. A., Krieg P. A., Rebagliati M. R., Maniatis T., Zinn K., Green M. R. Efficient in vitro synthesis of biologically active RNA and RNA hybridization probes from plasmids containing a bacteriophage SP6 promoter. Nucleic Acids Res. 1984 Sep 25;12(18):7035–7056. doi: 10.1093/nar/12.18.7035. [DOI] [PMC free article] [PubMed] [Google Scholar]
- Pappone P. A. Voltage-clamp experiments in normal and denervated mammalian skeletal muscle fibres. J Physiol. 1980 Sep;306:377–410. doi: 10.1113/jphysiol.1980.sp013403. [DOI] [PMC free article] [PubMed] [Google Scholar]
- Redfern P., Thesleff S. Action potential generation in denervated rat skeletal muscle. II. The action of tetrodotoxin. Acta Physiol Scand. 1971 May;82(1):70–78. doi: 10.1111/j.1748-1716.1971.tb04943.x. [DOI] [PubMed] [Google Scholar]
- Roberts W. M. Sodium channels near end-plates and nuclei of snake skeletal muscle. J Physiol. 1987 Jul;388:213–232. doi: 10.1113/jphysiol.1987.sp016611. [DOI] [PMC free article] [PubMed] [Google Scholar]
- Rogart R. B., Cribbs L. L., Muglia L. K., Kephart D. D., Kaiser M. W. Molecular cloning of a putative tetrodotoxin-resistant rat heart Na+ channel isoform. Proc Natl Acad Sci U S A. 1989 Oct;86(20):8170–8174. doi: 10.1073/pnas.86.20.8170. [DOI] [PMC free article] [PubMed] [Google Scholar]
- Ruff R. L. Na current density at and away from end plates on rat fast- and slow-twitch skeletal muscle fibers. Am J Physiol. 1992 Jan;262(1 Pt 1):C229–C234. doi: 10.1152/ajpcell.1992.262.1.C229. [DOI] [PubMed] [Google Scholar]
- Sellin L. C., Libelius R., Lundquist I., Tågerud S., Thesleff S. Membrane and biochemical alterations after denervation and during reinnervation of mouse skeletal muscle. Acta Physiol Scand. 1980 Oct;110(2):181–186. doi: 10.1111/j.1748-1716.1980.tb06649.x. [DOI] [PubMed] [Google Scholar]
- Sellin L. C., Thesleff S. Alterations in membrane electrical properties during long-term denervation of rat skeletal muscles. Acta Physiol Scand. 1980 Mar;108(3):243–246. doi: 10.1111/j.1748-1716.1980.tb06529.x. [DOI] [PubMed] [Google Scholar]
- Stühmer W., Almers W. Photobleaching through glass micropipettes: sodium channels without lateral mobility in the sarcolemma of frog skeletal muscle. Proc Natl Acad Sci U S A. 1982 Feb;79(3):946–950. doi: 10.1073/pnas.79.3.946. [DOI] [PMC free article] [PubMed] [Google Scholar]
- Trimmer J. S., Cooperman S. S., Agnew W. S., Mandel G. Regulation of muscle sodium channel transcripts during development and in response to denervation. Dev Biol. 1990 Dec;142(2):360–367. doi: 10.1016/0012-1606(90)90356-n. [DOI] [PubMed] [Google Scholar]
- Usdin T. B., Fischbach G. D. Purification and characterization of a polypeptide from chick brain that promotes the accumulation of acetylcholine receptors in chick myotubes. J Cell Biol. 1986 Aug;103(2):493–507. doi: 10.1083/jcb.103.2.493. [DOI] [PMC free article] [PubMed] [Google Scholar]
- Yang J. S., Sladky J. T., Kallen R. G., Barchi R. L. TTX-sensitive and TTX-insensitive sodium channel mRNA transcripts are independently regulated in adult skeletal muscle after denervation. Neuron. 1991 Sep;7(3):421–427. doi: 10.1016/0896-6273(91)90294-a. [DOI] [PubMed] [Google Scholar]