Abstract
1. Voltage-dependent inactivating single-channel potassium currents were recorded in cell-attached and inside-out patches from embryonic Xenopus myocytes differentiating in culture. 2. Channels with rapid inactivation (time constants < 25 ms) and with slow inactivation (time constants > 80 ms) recorded after one day in vitro appear to belong to two functionally different classes. Rapidly and slowly inactivating channels show steady-state inactivation with potentials of half-inactivation of -74 +/- 7 and -44 +/- 9 mV. They exhibit voltage-dependent activation, with times to half-maximal activation of 0.79 +/- 0.09 and 1.17 +/- 0.22 ms when stepped from -120 to +40 mV. Rapidly inactivating channels also have a lower open probability than slowly inactivating ones. The channels have similar conductances of 23 +/- 6 and 17 +/- 4 pS and extrapolated reversal potentials close to the potassium equilibrium potential. 3. In cell-attached patches, inactivation behaviours of channels with rapid or slow inactivation do not change during recording. After patch excision, rapidly inactivating channels usually switch to a slow inactivation mode. Slowly inactivating channels derived from rapidly inactivating channels after patch excision retain their conductance and extrapolated reversal potential, but are not distinguishable from native slowly inactivating channels with respect to steady-state inactivation, activation and inactivation times, as well as open probabilities. 4. The change in inactivation behaviour of rapidly inactivating channels after patch excision is reversed by application of reduced dithiothreitol (DTT). In contrast, channels with slow inactivation in the cell-attached mode do not change in to rapidly inactivating channels after application of DTT in the excised configuration, suggesting that these channels belong to a structurally different class. 5. Frequent observation of superposing channel openings indicates clustering of inactivating potassium channels in the myocyte membrane, since many patches lack channel activity. Clustering does not depend on the presence of differentiating neurones. 6. Channels with rapid inactivation increase 6-fold in density during the first day in culture in the presence of neurones; channel density decreases in their absence. Channels with slow inactivation increase 2-fold in density in the presence or absence of differentiating neurones during this period. 7. Channels with rapid or slow inactivation in cell-attached membrane belong to functionally distinct classes that are developmentally regulated differently. Reversible changes from rapid to slow inactivation mode after patch excision suggest that the channels may be structurally related.
Full text
PDF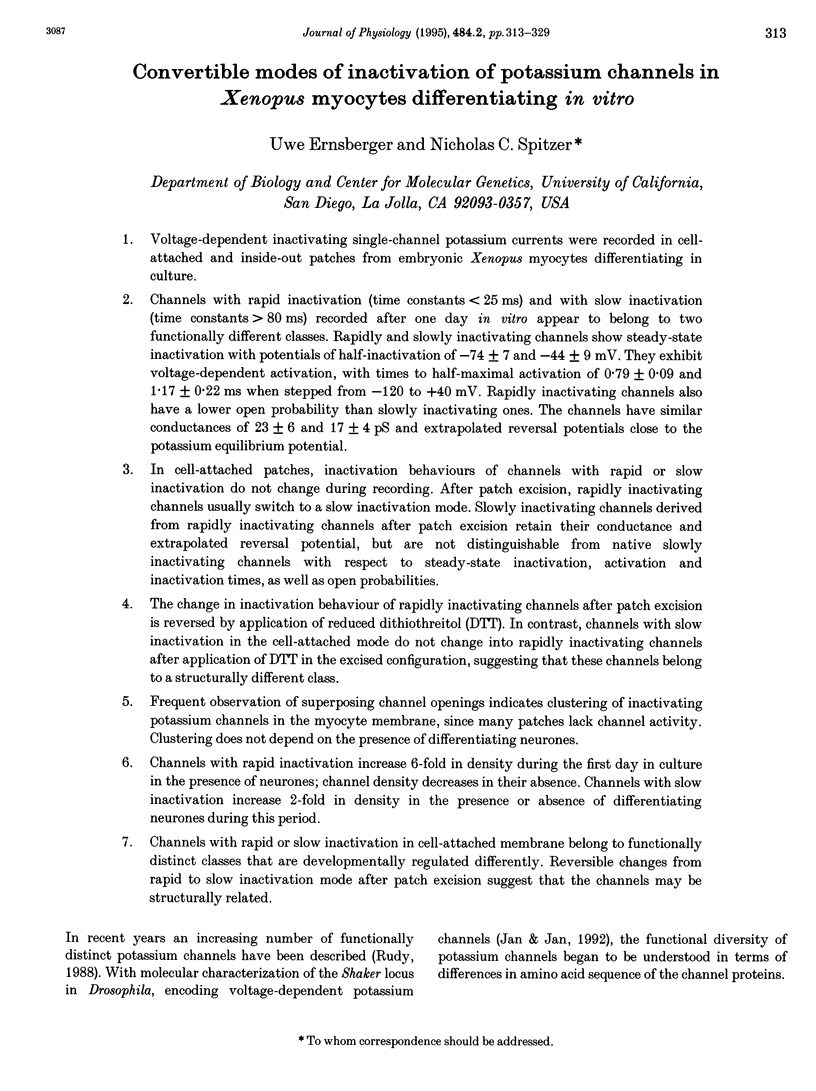
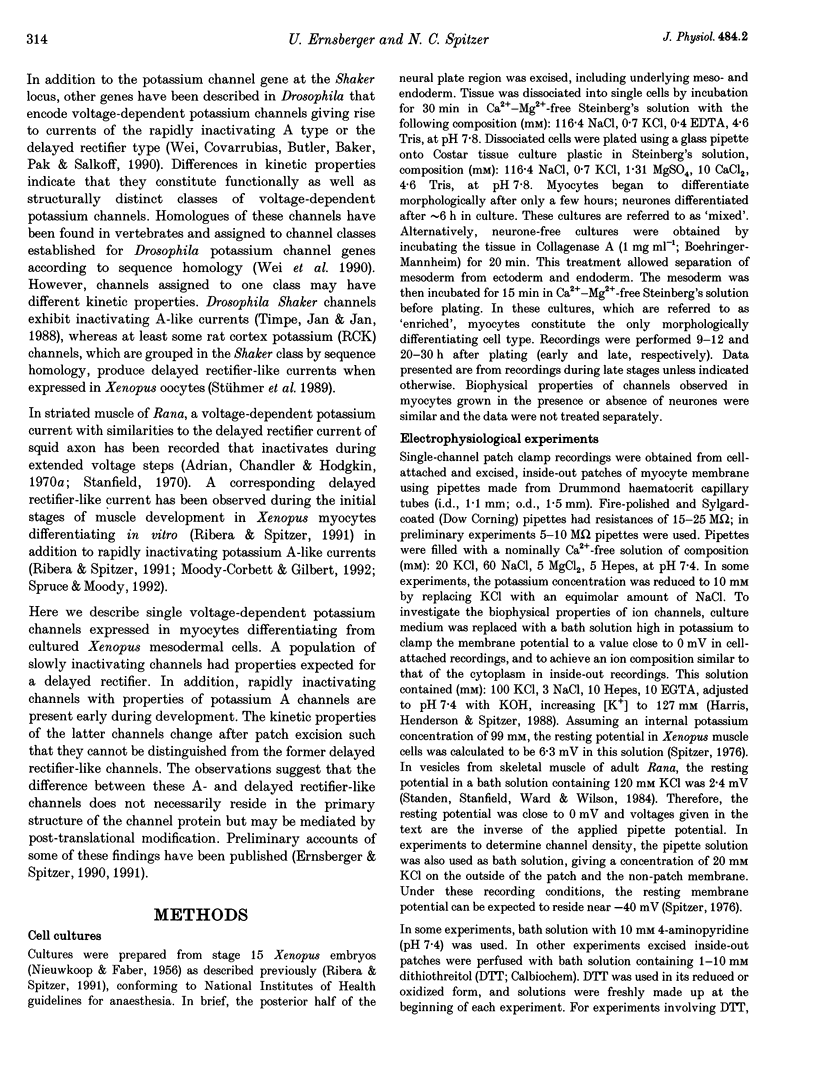
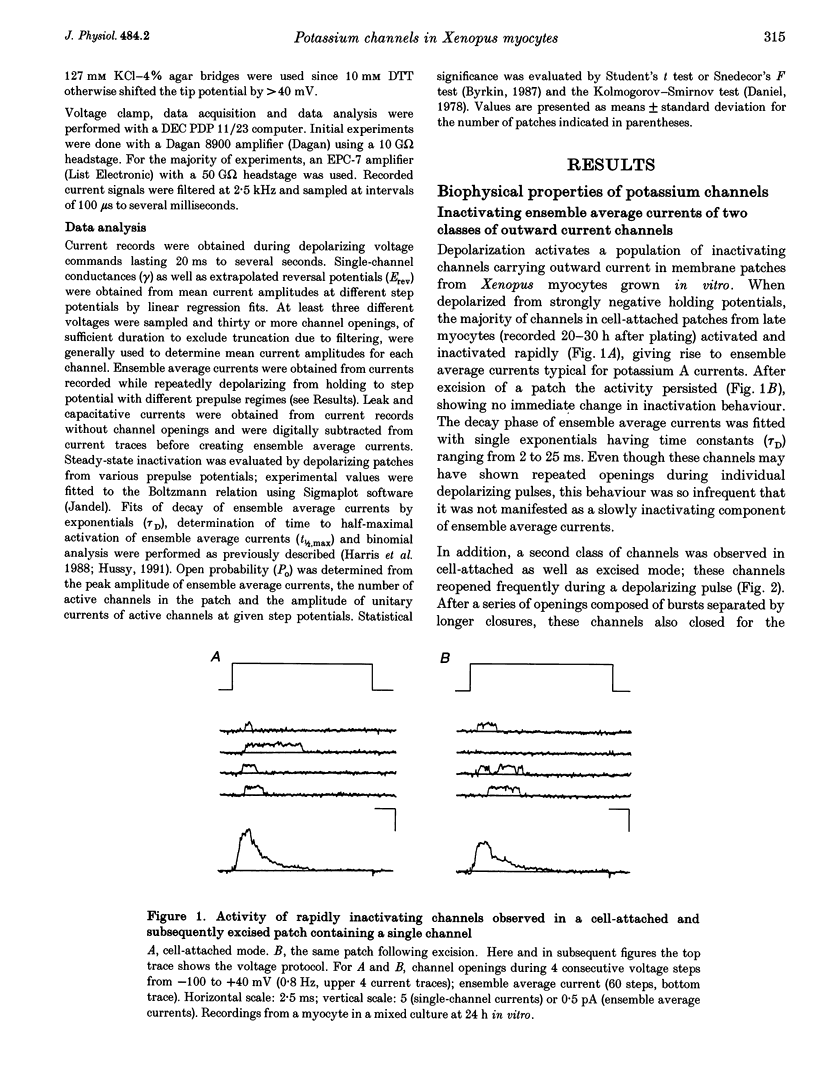
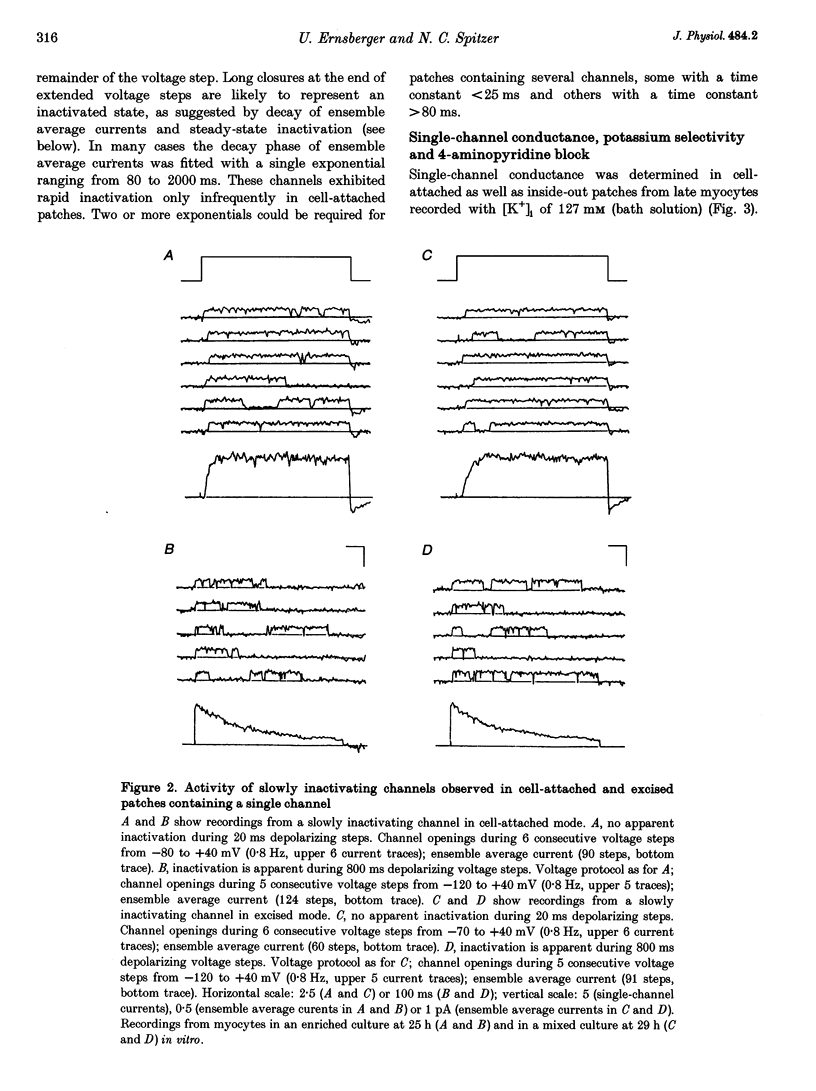
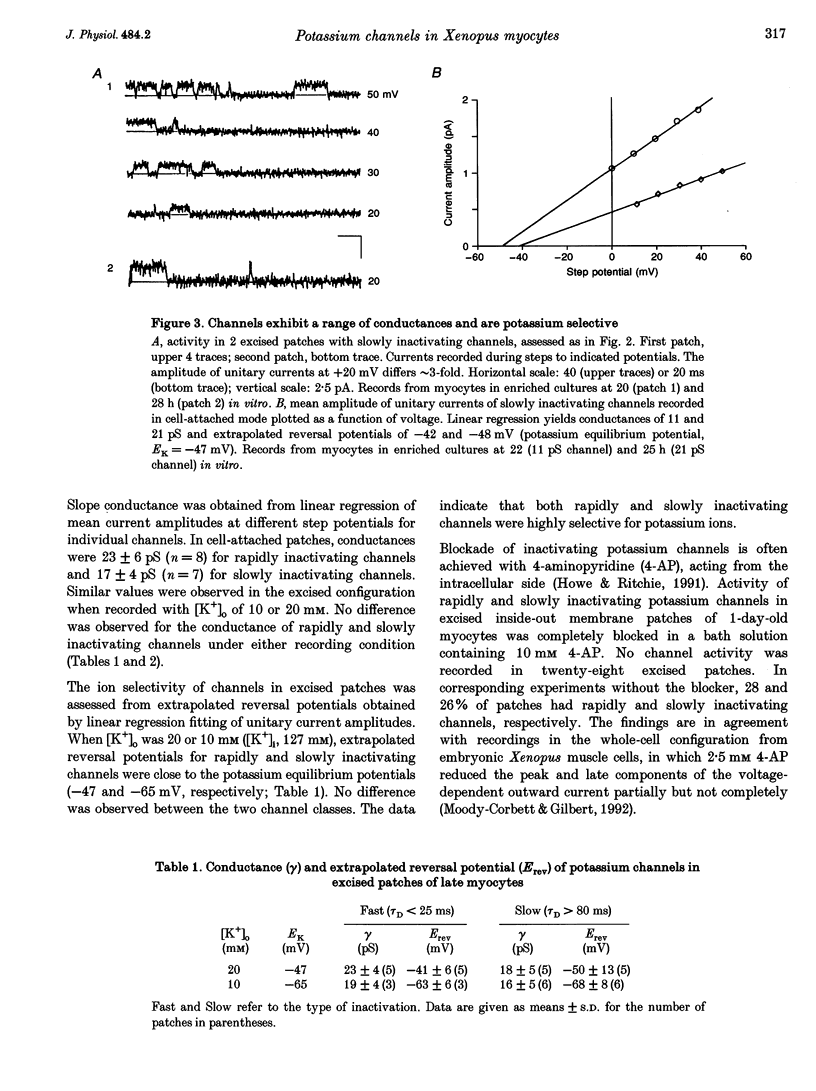
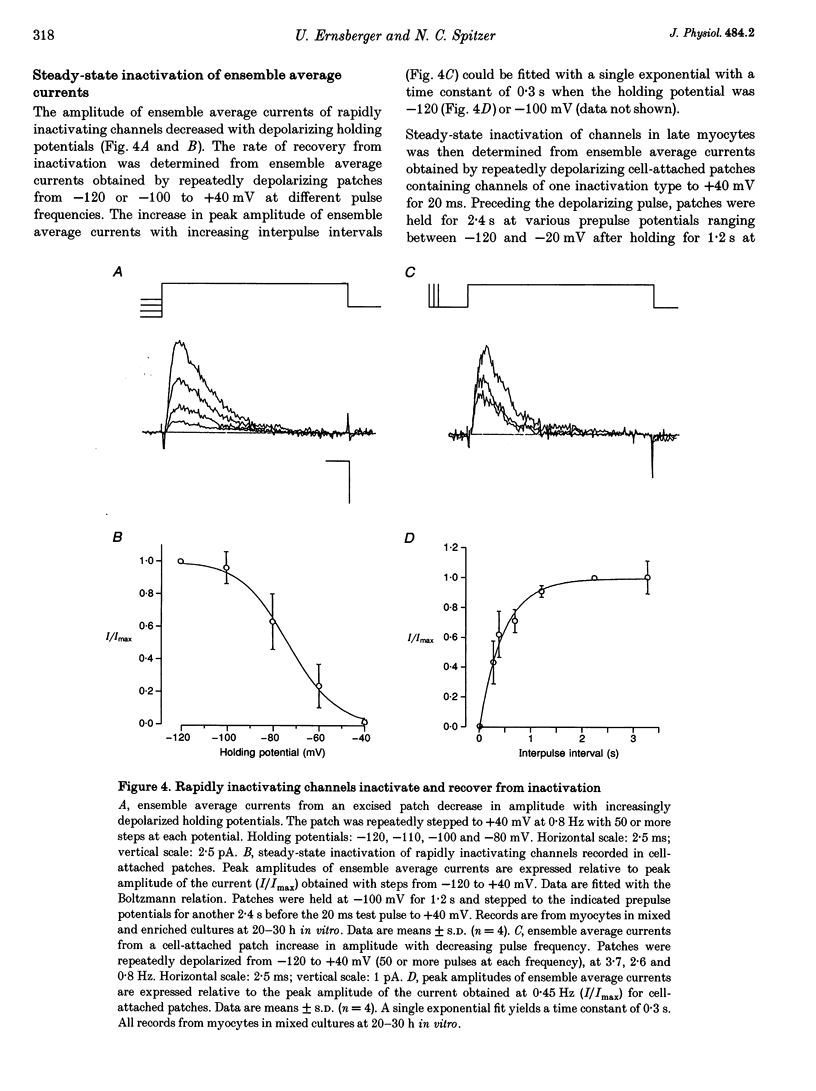
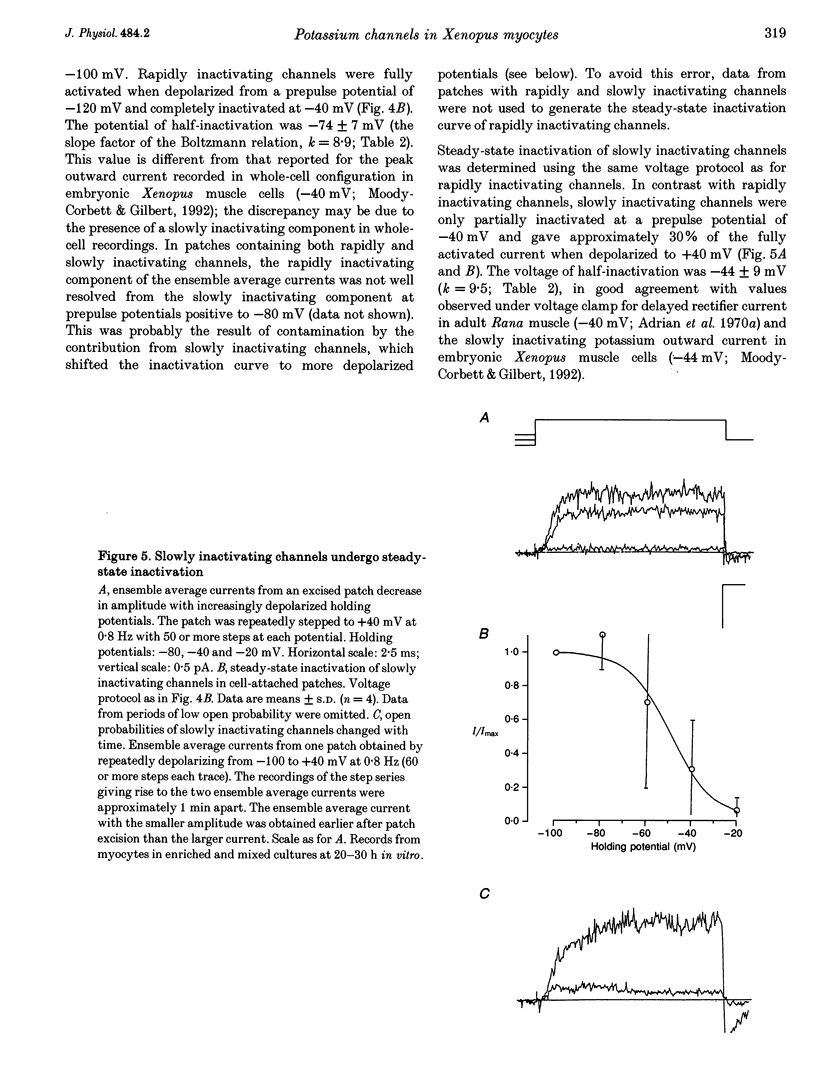
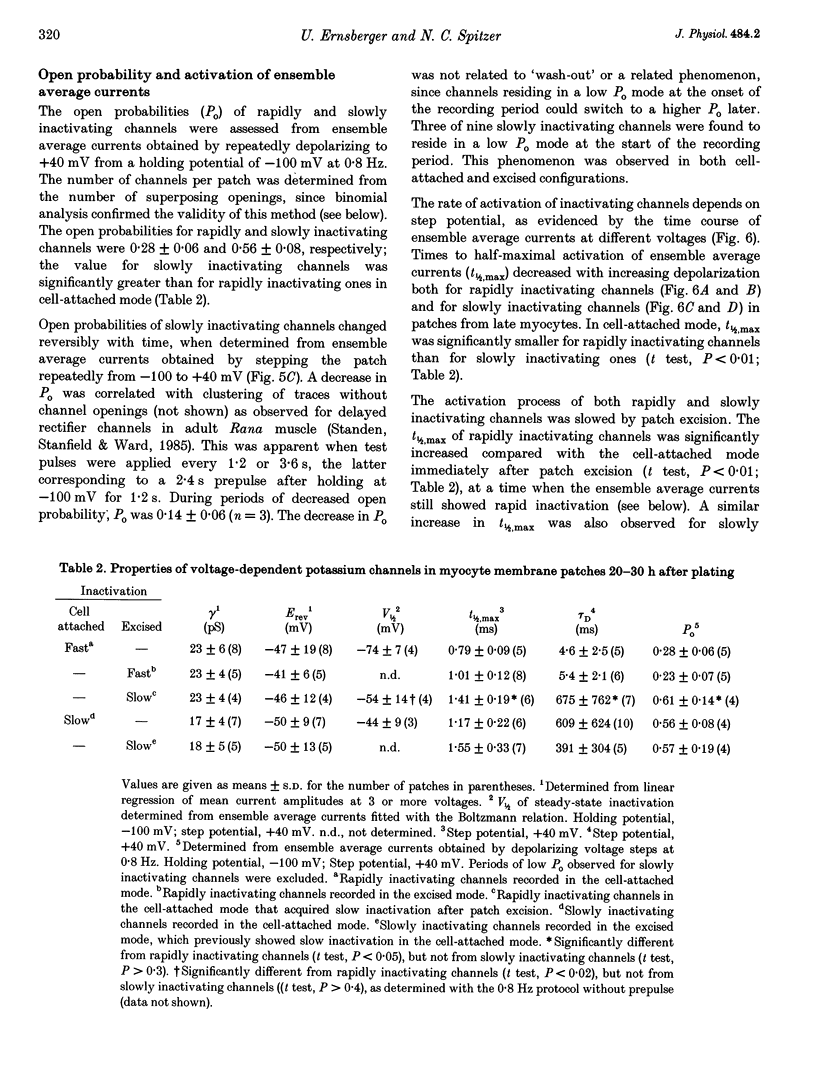
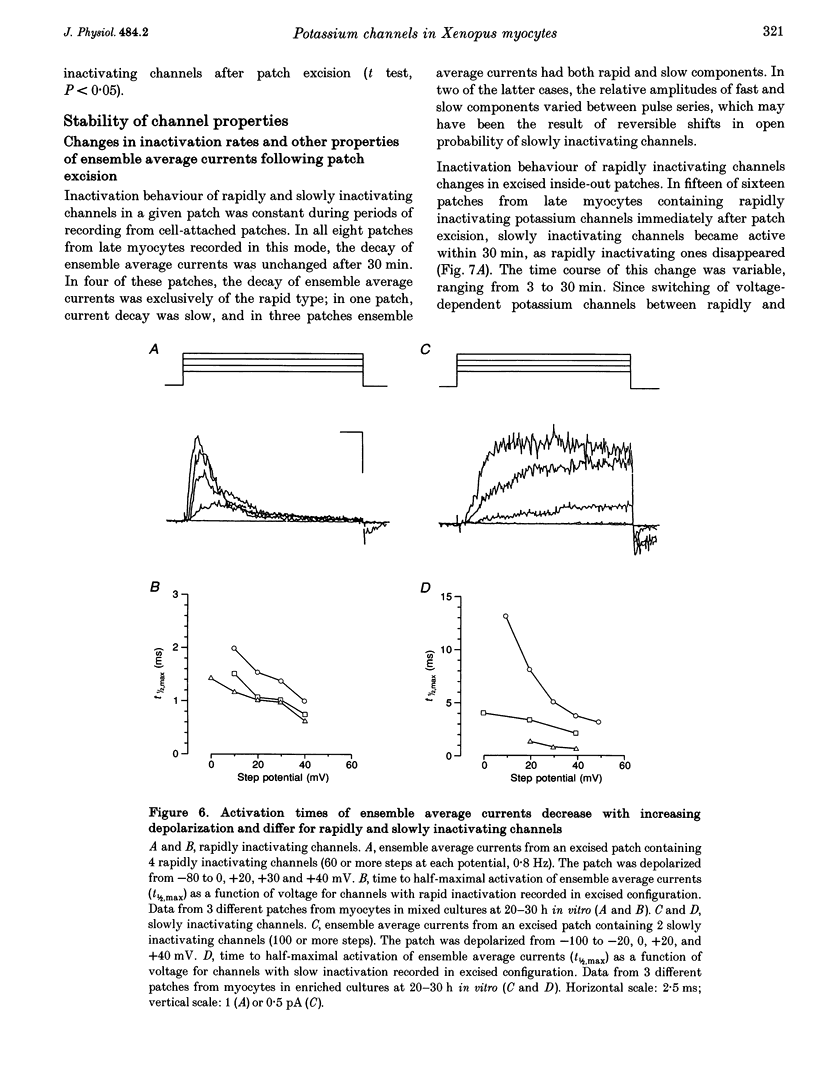
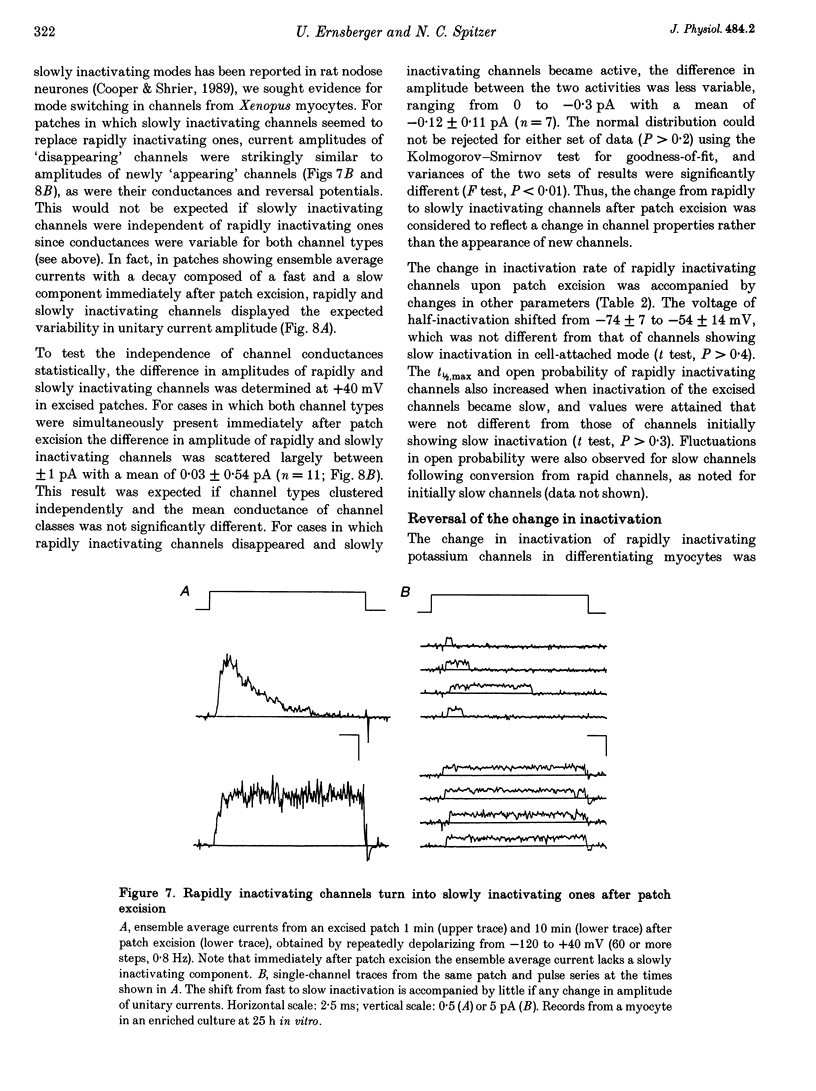
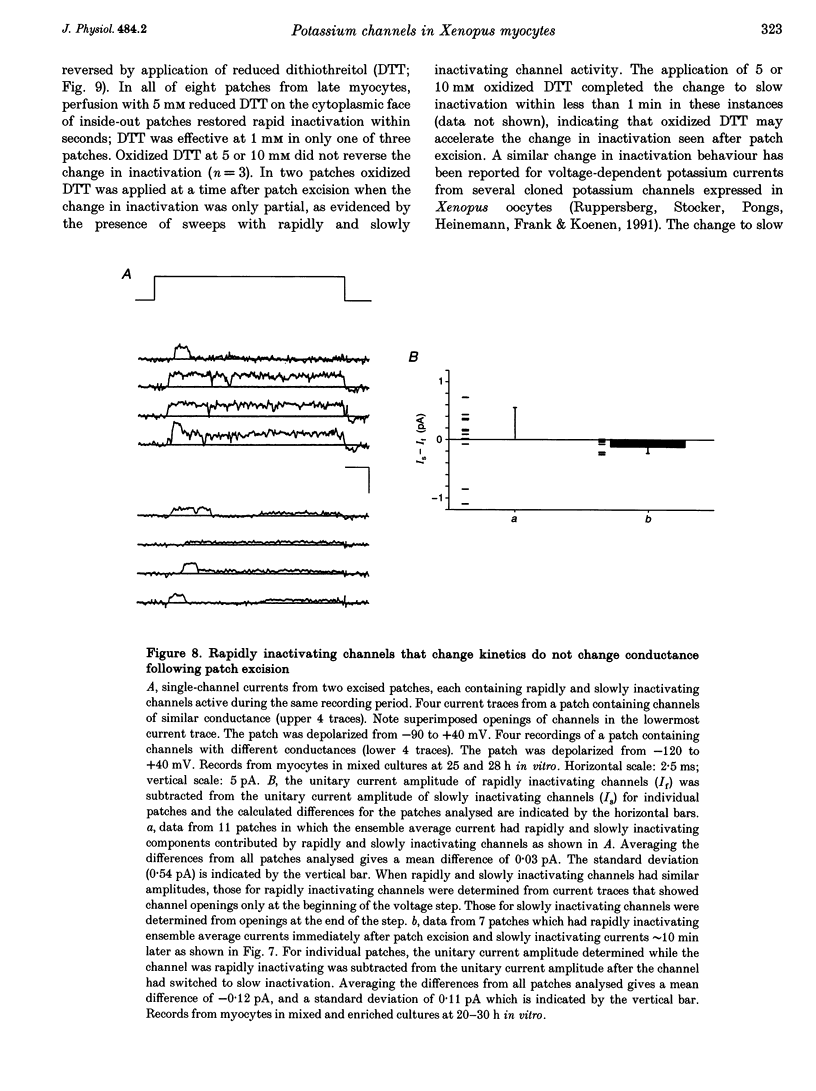
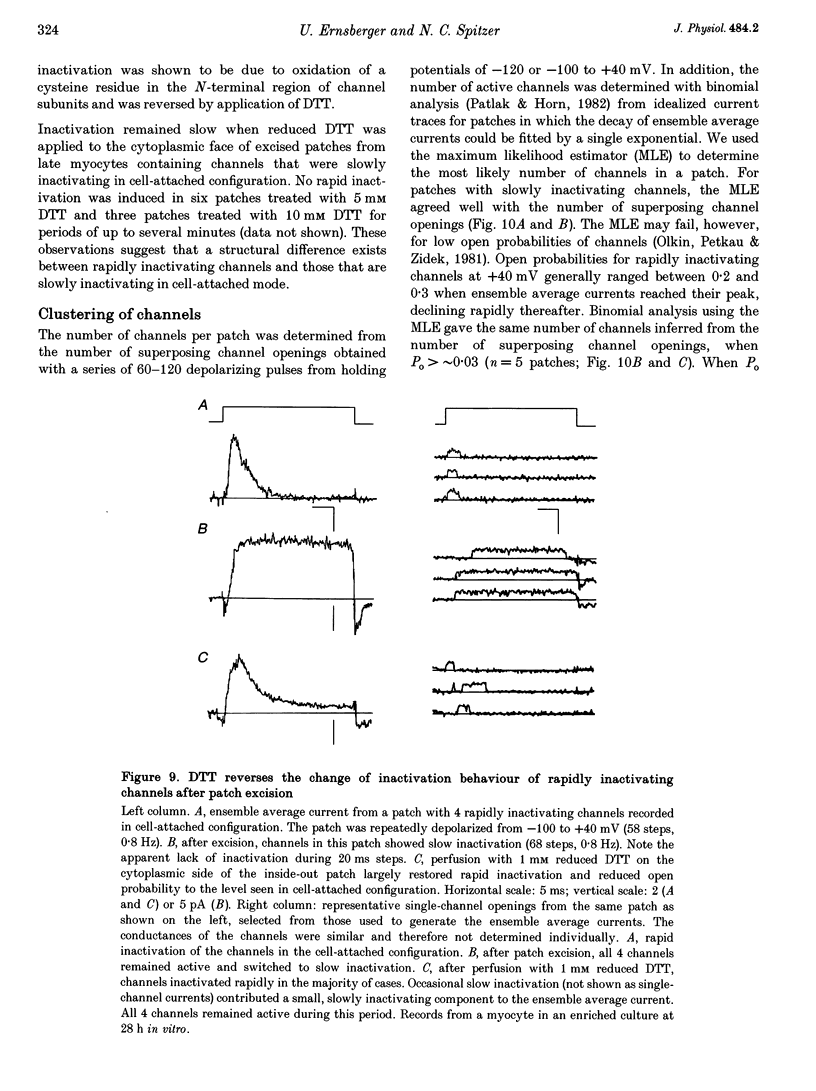
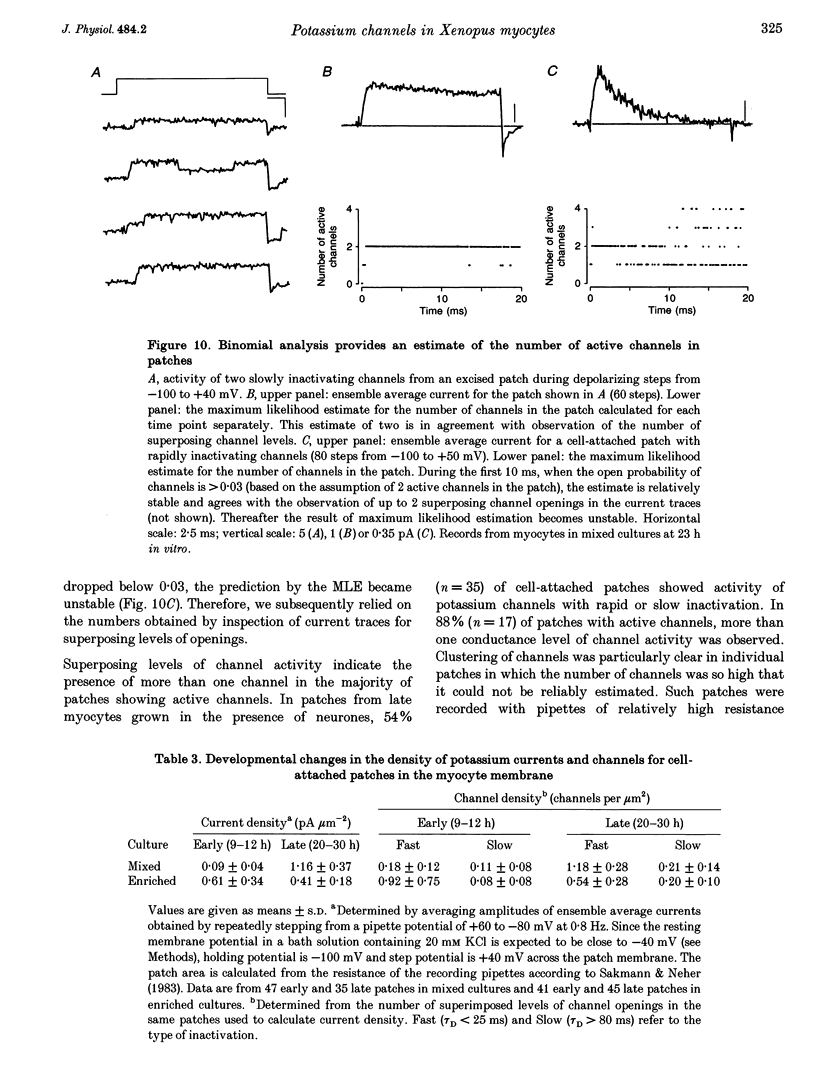
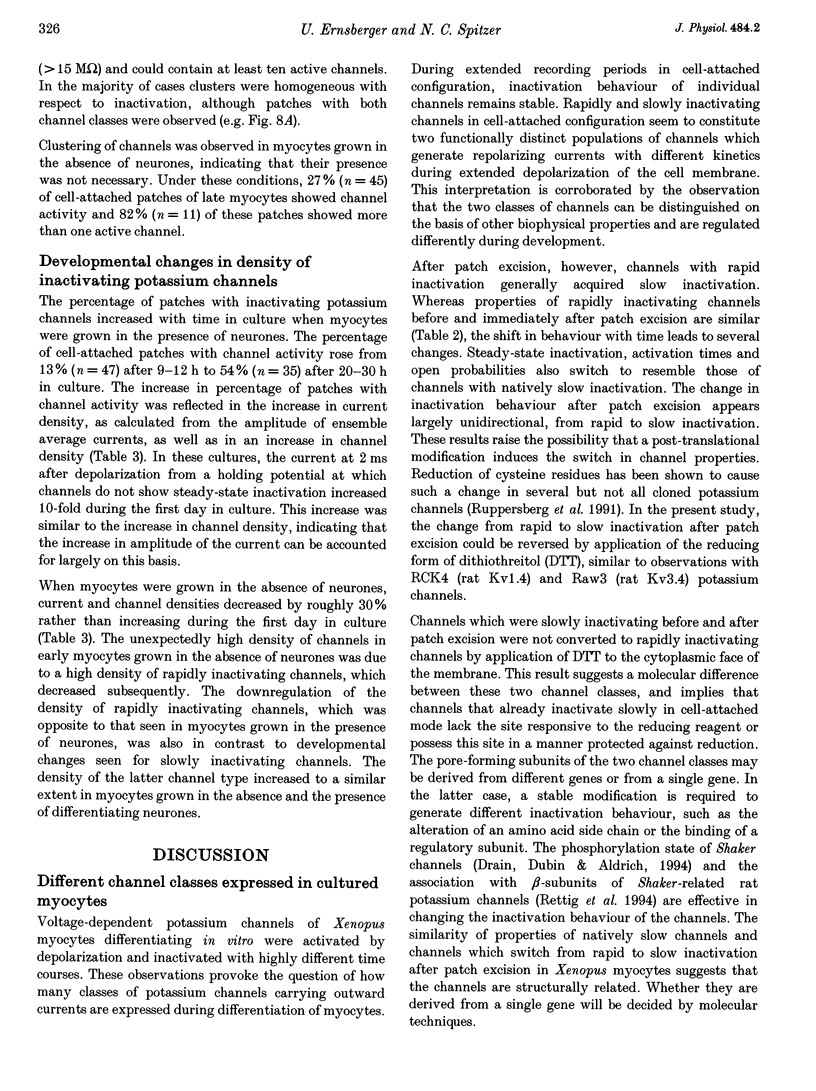
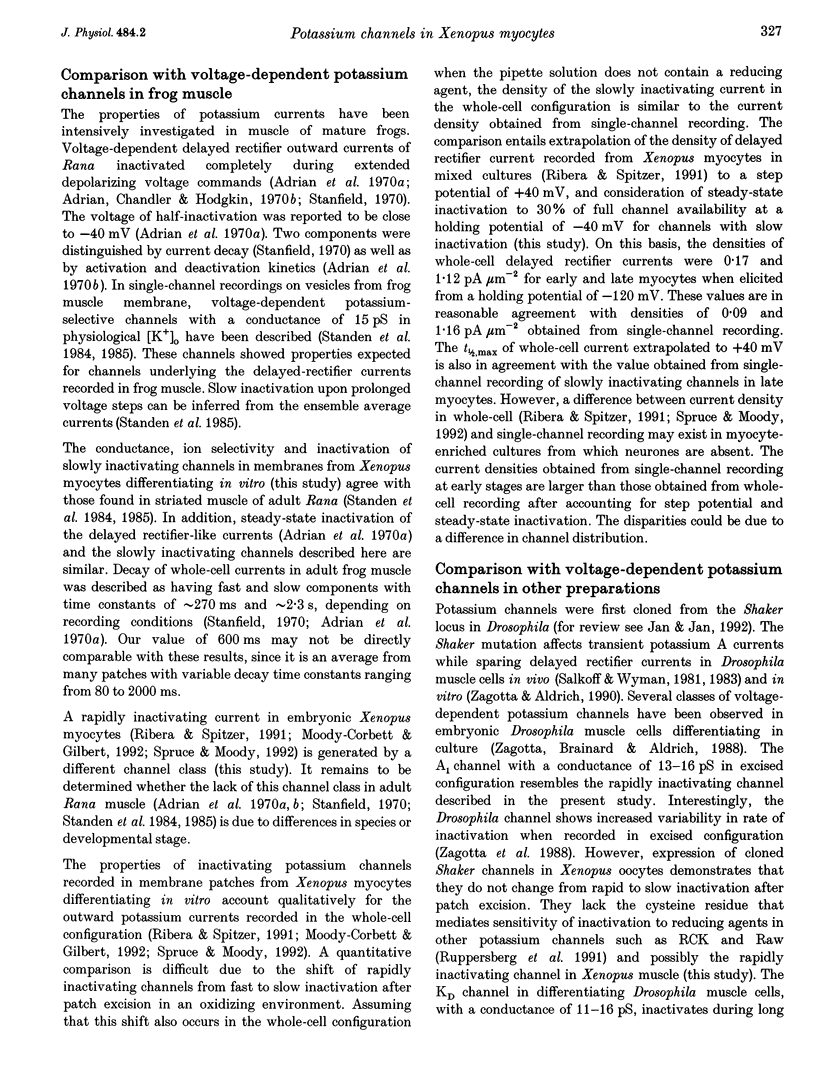
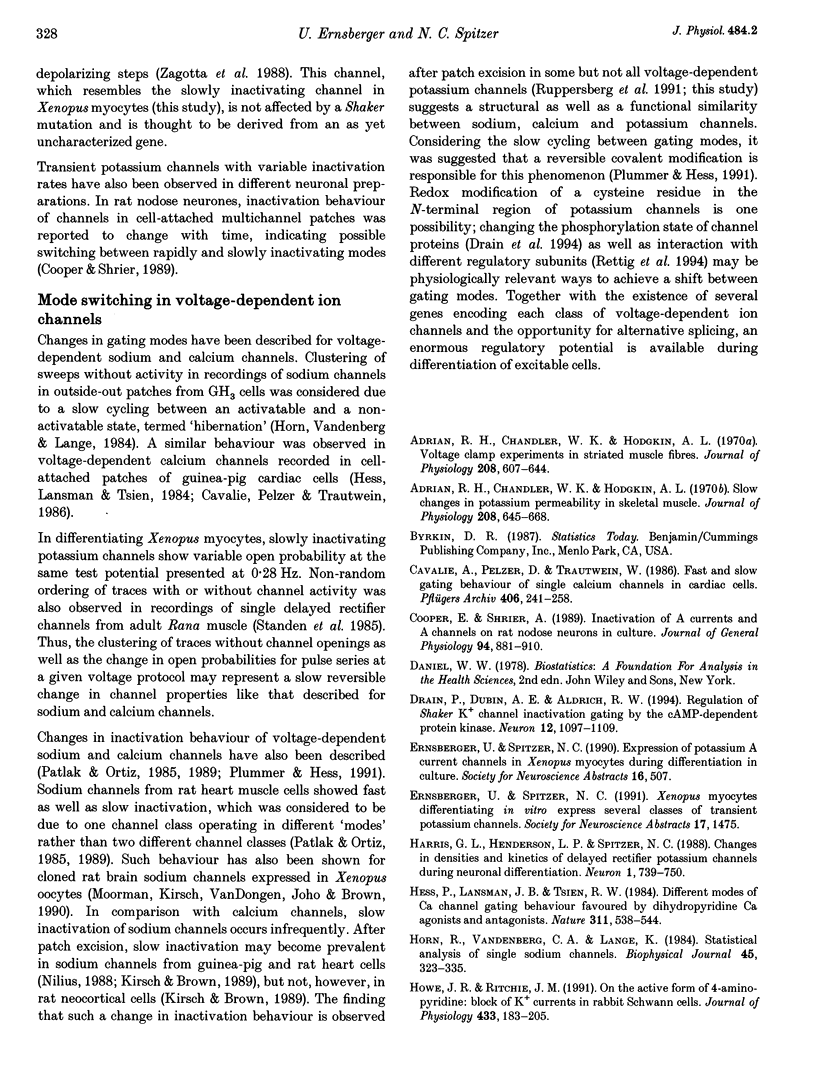
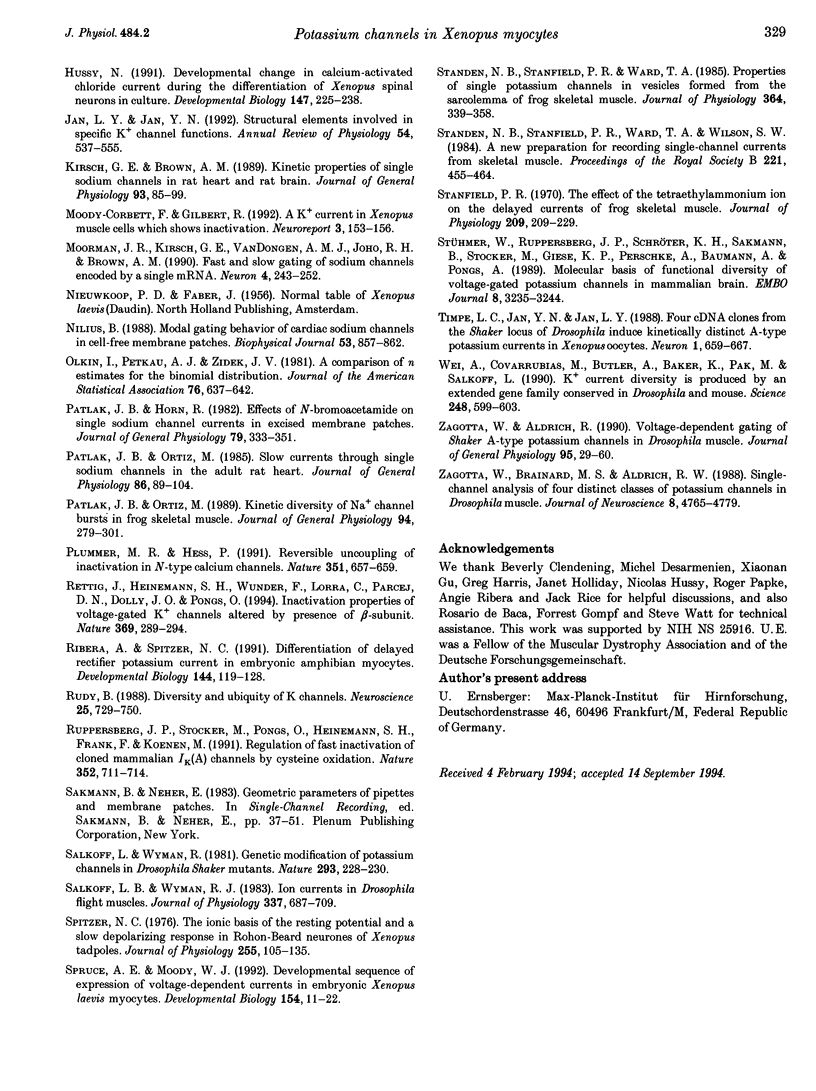
Selected References
These references are in PubMed. This may not be the complete list of references from this article.
- Adrian R. H., Chandler W. K., Hodgkin A. L. Slow changes in potassium permeability in skeletal muscle. J Physiol. 1970 Jul;208(3):645–668. doi: 10.1113/jphysiol.1970.sp009140. [DOI] [PMC free article] [PubMed] [Google Scholar]
- Adrian R. H., Chandler W. K., Hodgkin A. L. Voltage clamp experiments in striated muscle fibres. J Physiol. 1970 Jul;208(3):607–644. doi: 10.1113/jphysiol.1970.sp009139. [DOI] [PMC free article] [PubMed] [Google Scholar]
- Cavalié A., Pelzer D., Trautwein W. Fast and slow gating behaviour of single calcium channels in cardiac cells. Relation to activation and inactivation of calcium-channel current. Pflugers Arch. 1986 Mar;406(3):241–258. doi: 10.1007/BF00640910. [DOI] [PubMed] [Google Scholar]
- Cooper E., Shrier A. Inactivation of A currents and A channels on rat nodose neurons in culture. J Gen Physiol. 1989 Nov;94(5):881–910. doi: 10.1085/jgp.94.5.881. [DOI] [PMC free article] [PubMed] [Google Scholar]
- Drain P., Dubin A. E., Aldrich R. W. Regulation of Shaker K+ channel inactivation gating by the cAMP-dependent protein kinase. Neuron. 1994 May;12(5):1097–1109. doi: 10.1016/0896-6273(94)90317-4. [DOI] [PubMed] [Google Scholar]
- Harris G. L., Henderson L. P., Spitzer N. C. Changes in densities and kinetics of delayed rectifier potassium channels during neuronal differentiation. Neuron. 1988 Oct;1(8):739–750. doi: 10.1016/0896-6273(88)90172-9. [DOI] [PubMed] [Google Scholar]
- Hess P., Lansman J. B., Tsien R. W. Different modes of Ca channel gating behaviour favoured by dihydropyridine Ca agonists and antagonists. Nature. 1984 Oct 11;311(5986):538–544. doi: 10.1038/311538a0. [DOI] [PubMed] [Google Scholar]
- Horn R., Vandenberg C. A., Lange K. Statistical analysis of single sodium channels. Effects of N-bromoacetamide. Biophys J. 1984 Jan;45(1):323–335. doi: 10.1016/S0006-3495(84)84158-2. [DOI] [PMC free article] [PubMed] [Google Scholar]
- Howe J. R., Ritchie J. M. On the active form of 4-aminopyridine: block of K+ currents in rabbit Schwann cells. J Physiol. 1991 Feb;433:183–205. doi: 10.1113/jphysiol.1991.sp018421. [DOI] [PMC free article] [PubMed] [Google Scholar]
- Hussy N. Developmental change in calcium-activated chloride current during the differentiation of Xenopus spinal neurons in culture. Dev Biol. 1991 Sep;147(1):225–238. doi: 10.1016/s0012-1606(05)80020-x. [DOI] [PubMed] [Google Scholar]
- Jan L. Y., Jan Y. N. Structural elements involved in specific K+ channel functions. Annu Rev Physiol. 1992;54:537–555. doi: 10.1146/annurev.ph.54.030192.002541. [DOI] [PubMed] [Google Scholar]
- Kirsch G. E., Brown A. M. Kinetic properties of single sodium channels in rat heart and rat brain. J Gen Physiol. 1989 Jan;93(1):85–99. doi: 10.1085/jgp.93.1.85. [DOI] [PMC free article] [PubMed] [Google Scholar]
- Moody-Corbett F., Gilbert R. A K+ current in Xenopus muscle cells which shows inactivation. Neuroreport. 1992 Feb;3(2):153–156. doi: 10.1097/00001756-199202000-00008. [DOI] [PubMed] [Google Scholar]
- Moorman J. R., Kirsch G. E., VanDongen A. M., Joho R. H., Brown A. M. Fast and slow gating of sodium channels encoded by a single mRNA. Neuron. 1990 Feb;4(2):243–252. doi: 10.1016/0896-6273(90)90099-2. [DOI] [PubMed] [Google Scholar]
- Nilius B. Modal gating behavior of cardiac sodium channels in cell-free membrane patches. Biophys J. 1988 Jun;53(6):857–862. doi: 10.1016/S0006-3495(88)83166-7. [DOI] [PMC free article] [PubMed] [Google Scholar]
- Patlak J. B., Ortiz M. Kinetic diversity of Na+ channel bursts in frog skeletal muscle. J Gen Physiol. 1989 Aug;94(2):279–301. doi: 10.1085/jgp.94.2.279. [DOI] [PMC free article] [PubMed] [Google Scholar]
- Patlak J. B., Ortiz M. Slow currents through single sodium channels of the adult rat heart. J Gen Physiol. 1985 Jul;86(1):89–104. doi: 10.1085/jgp.86.1.89. [DOI] [PMC free article] [PubMed] [Google Scholar]
- Patlak J., Horn R. Effect of N-bromoacetamide on single sodium channel currents in excised membrane patches. J Gen Physiol. 1982 Mar;79(3):333–351. doi: 10.1085/jgp.79.3.333. [DOI] [PMC free article] [PubMed] [Google Scholar]
- Plummer M. R., Hess P. Reversible uncoupling of inactivation in N-type calcium channels. Nature. 1991 Jun 20;351(6328):657–659. doi: 10.1038/351657a0. [DOI] [PubMed] [Google Scholar]
- Rettig J., Heinemann S. H., Wunder F., Lorra C., Parcej D. N., Dolly J. O., Pongs O. Inactivation properties of voltage-gated K+ channels altered by presence of beta-subunit. Nature. 1994 May 26;369(6478):289–294. doi: 10.1038/369289a0. [DOI] [PubMed] [Google Scholar]
- Ribera A. B., Spitzer N. C. Differentiation of delayed rectifier potassium current in embryonic amphibian myocytes. Dev Biol. 1991 Mar;144(1):119–128. doi: 10.1016/0012-1606(91)90484-k. [DOI] [PubMed] [Google Scholar]
- Rudy B. Diversity and ubiquity of K channels. Neuroscience. 1988 Jun;25(3):729–749. doi: 10.1016/0306-4522(88)90033-4. [DOI] [PubMed] [Google Scholar]
- Ruppersberg J. P., Stocker M., Pongs O., Heinemann S. H., Frank R., Koenen M. Regulation of fast inactivation of cloned mammalian IK(A) channels by cysteine oxidation. Nature. 1991 Aug 22;352(6337):711–714. doi: 10.1038/352711a0. [DOI] [PubMed] [Google Scholar]
- Salkoff L. B., Wyman R. J. Ion currents in Drosophila flight muscles. J Physiol. 1983 Apr;337:687–709. doi: 10.1113/jphysiol.1983.sp014649. [DOI] [PMC free article] [PubMed] [Google Scholar]
- Salkoff L., Wyman R. Genetic modification of potassium channels in Drosophila Shaker mutants. Nature. 1981 Sep 17;293(5829):228–230. doi: 10.1038/293228a0. [DOI] [PubMed] [Google Scholar]
- Spitzer N. C. The ionic basis of the resting potential and a slow depolarizing response in Rohon-Beard neurones of Xenopus tadpoles. J Physiol. 1976 Feb;255(1):105–135. doi: 10.1113/jphysiol.1976.sp011272. [DOI] [PMC free article] [PubMed] [Google Scholar]
- Spruce A. E., Moody W. J. Developmental sequence of expression of voltage-dependent currents in embryonic Xenopus laevis myocytes. Dev Biol. 1992 Nov;154(1):11–22. doi: 10.1016/0012-1606(92)90043-g. [DOI] [PubMed] [Google Scholar]
- Standen N. B., Stanfield P. R., Ward T. A. Properties of single potassium channels in vesicles formed from the sarcolemma of frog skeletal muscle. J Physiol. 1985 Jul;364:339–358. doi: 10.1113/jphysiol.1985.sp015749. [DOI] [PMC free article] [PubMed] [Google Scholar]
- Standen N. B., Stanfield P. R., Ward T. A., Wilson S. W. A new preparation for recording single-channel currents from skeletal muscle. Proc R Soc Lond B Biol Sci. 1984 Jun 22;221(1225):455–464. doi: 10.1098/rspb.1984.0044. [DOI] [PubMed] [Google Scholar]
- Stanfield P. R. The effect of the tetraethylammonium ion on the delayed currents of frog skeletal muscle. J Physiol. 1970 Jul;209(1):209–229. doi: 10.1113/jphysiol.1970.sp009163. [DOI] [PMC free article] [PubMed] [Google Scholar]
- Stühmer W., Ruppersberg J. P., Schröter K. H., Sakmann B., Stocker M., Giese K. P., Perschke A., Baumann A., Pongs O. Molecular basis of functional diversity of voltage-gated potassium channels in mammalian brain. EMBO J. 1989 Nov;8(11):3235–3244. doi: 10.1002/j.1460-2075.1989.tb08483.x. [DOI] [PMC free article] [PubMed] [Google Scholar]
- Timpe L. C., Jan Y. N., Jan L. Y. Four cDNA clones from the Shaker locus of Drosophila induce kinetically distinct A-type potassium currents in Xenopus oocytes. Neuron. 1988 Oct;1(8):659–667. doi: 10.1016/0896-6273(88)90165-1. [DOI] [PubMed] [Google Scholar]
- Wei A., Covarrubias M., Butler A., Baker K., Pak M., Salkoff L. K+ current diversity is produced by an extended gene family conserved in Drosophila and mouse. Science. 1990 May 4;248(4955):599–603. doi: 10.1126/science.2333511. [DOI] [PubMed] [Google Scholar]
- Zagotta W. N., Aldrich R. W. Voltage-dependent gating of Shaker A-type potassium channels in Drosophila muscle. J Gen Physiol. 1990 Jan;95(1):29–60. doi: 10.1085/jgp.95.1.29. [DOI] [PMC free article] [PubMed] [Google Scholar]
- Zagotta W. N., Brainard M. S., Aldrich R. W. Single-channel analysis of four distinct classes of potassium channels in Drosophila muscle. J Neurosci. 1988 Dec;8(12):4765–4779. doi: 10.1523/JNEUROSCI.08-12-04765.1988. [DOI] [PMC free article] [PubMed] [Google Scholar]