Abstract
1. Direct observations of perfused cerebral arterioles in vivo and in vitro have demonstrated that alkalinization of blood or cerebrospinal fluid (CSF) causes arteriolar constriction. Inasmuch as such alkalinizations lead to increases in intracellular pH (pHi) as well as interstitial pH (pHo), it is possible that increases in either pHi or pHo (or both) underlie alkalinization-induced cerebral vasoconstriction. In order to test the hypothesis that changes in pHi alone underline alkalinization-induced cerebral vasoconstriction, we simultaneously measured vessel diameter and pHi (using the pH-sensitive dye, SNAFL) in isolated cerebral arterioles from adult rats during imposed alterations in pHo and pHi. 2. Penetrating cerebral arterioles from the distribution of the middle cerebral artery were hand dissected, cannulated on one end and occluded distally. Vessels were inflated hydrostatically to 60 cmH2O under no-flow conditions. Confocal microscopy verified specific pH-sensitivity dye staining of the vascular smooth muscle cells within the vessel wall. 3. Extracellular alkalinization from pH 7.3 to 7.8 caused pHi to increase by 0.06 +/- 0.01 of a pH unit, and vessel diameter to decrease by 21.8 +/- 1.8% (mean +/- S.E.M.). 4. Intracellular alkalinization at constant pHo was produced by exposure to weak bases, including NH3 and trimethylamine, or by exposure to, followed by withdrawal of, weak acids, including CO2 and acetic acid. None of these treatments evoked vasoconstriction even though each of them caused increases in pHi greater than those observed in the same vessels during exposure to the pHo 7.8 solution. 5. We conclude that, at least in cerebral arterioles, alkalinization-induced vasoconstriction is mediated by an increase in pHo, not pHi [corrected].
Full text
PDF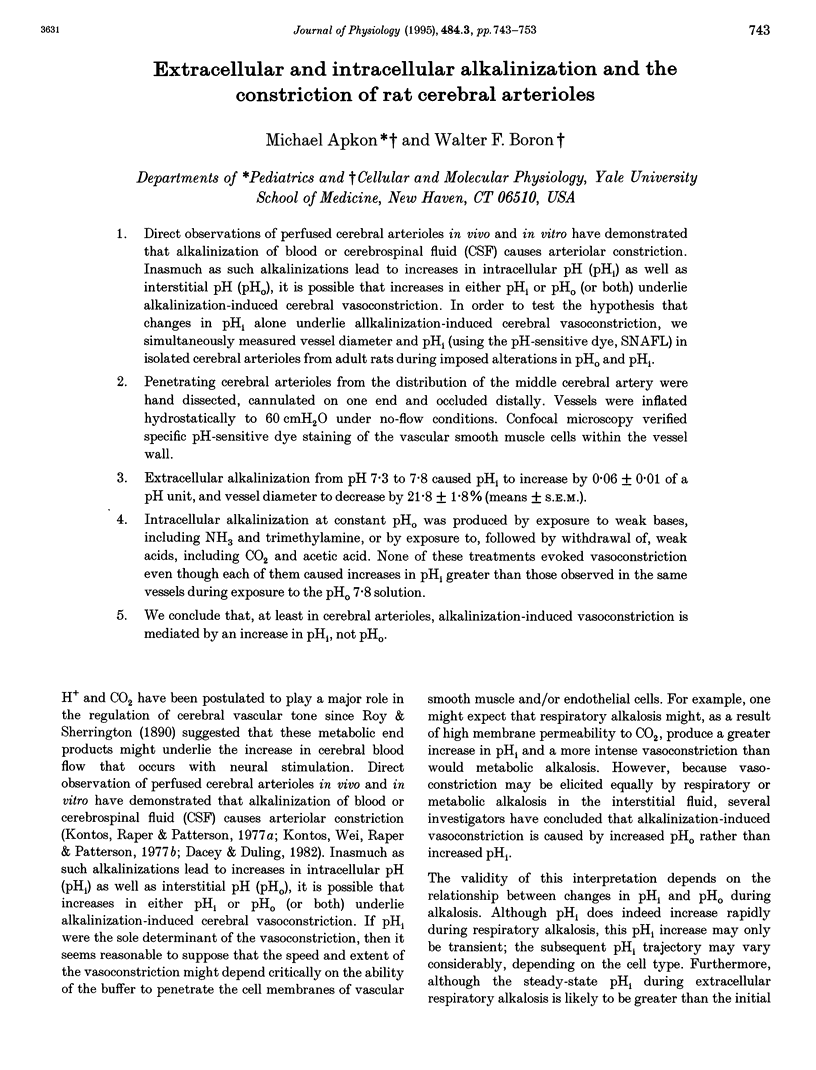
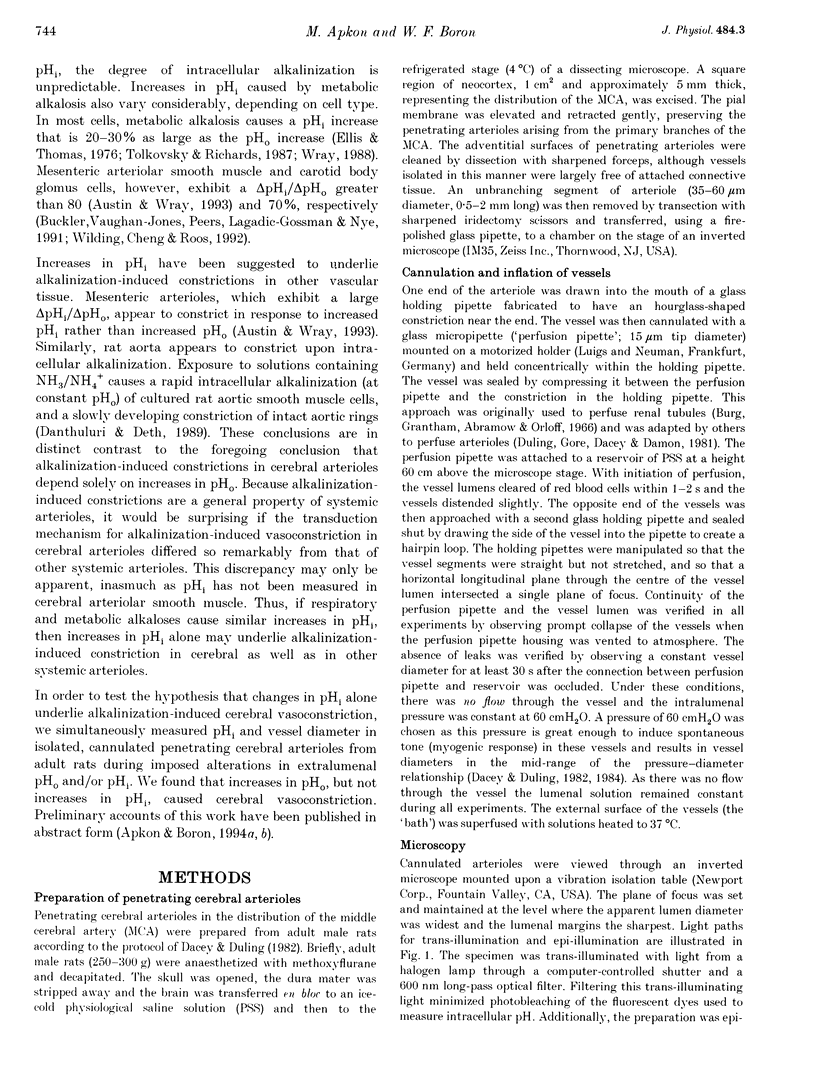
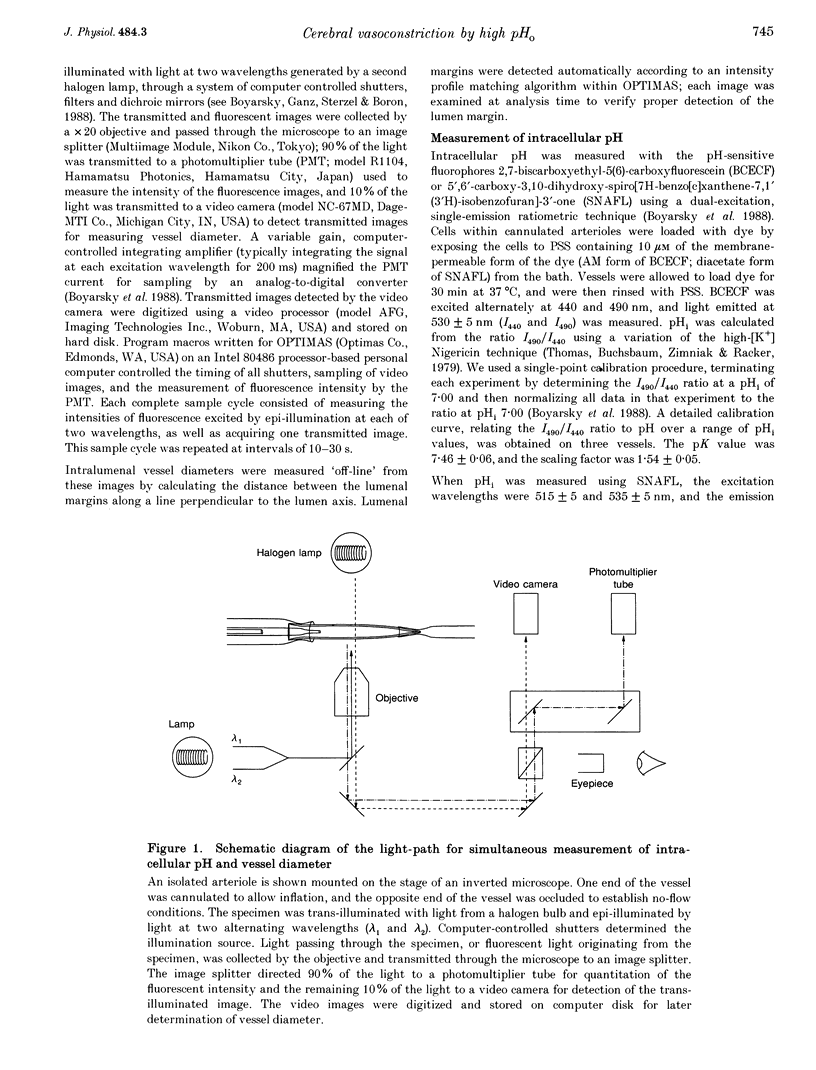
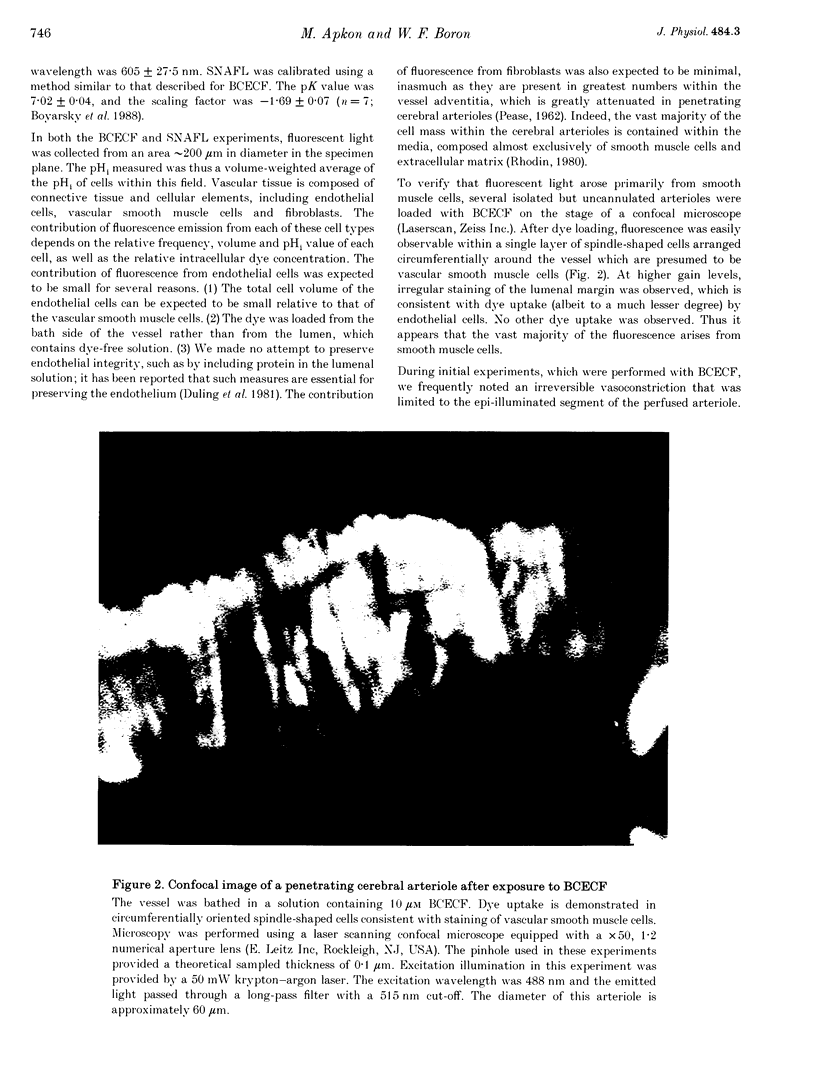
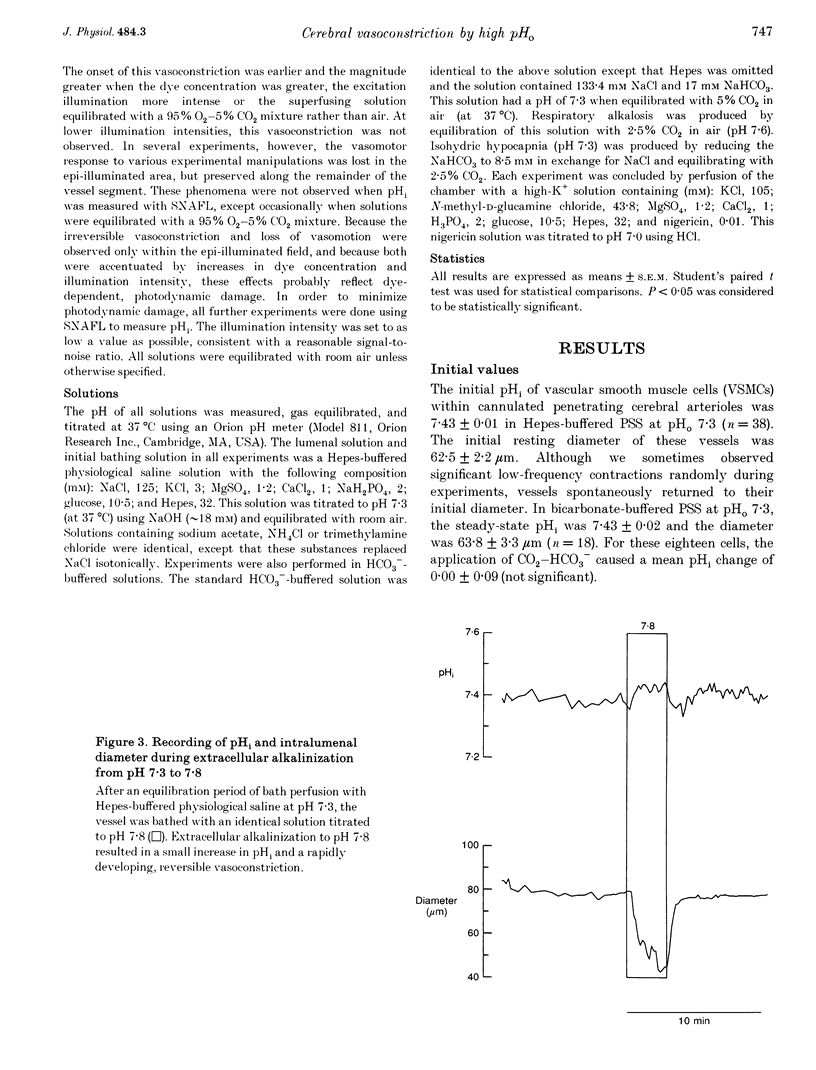
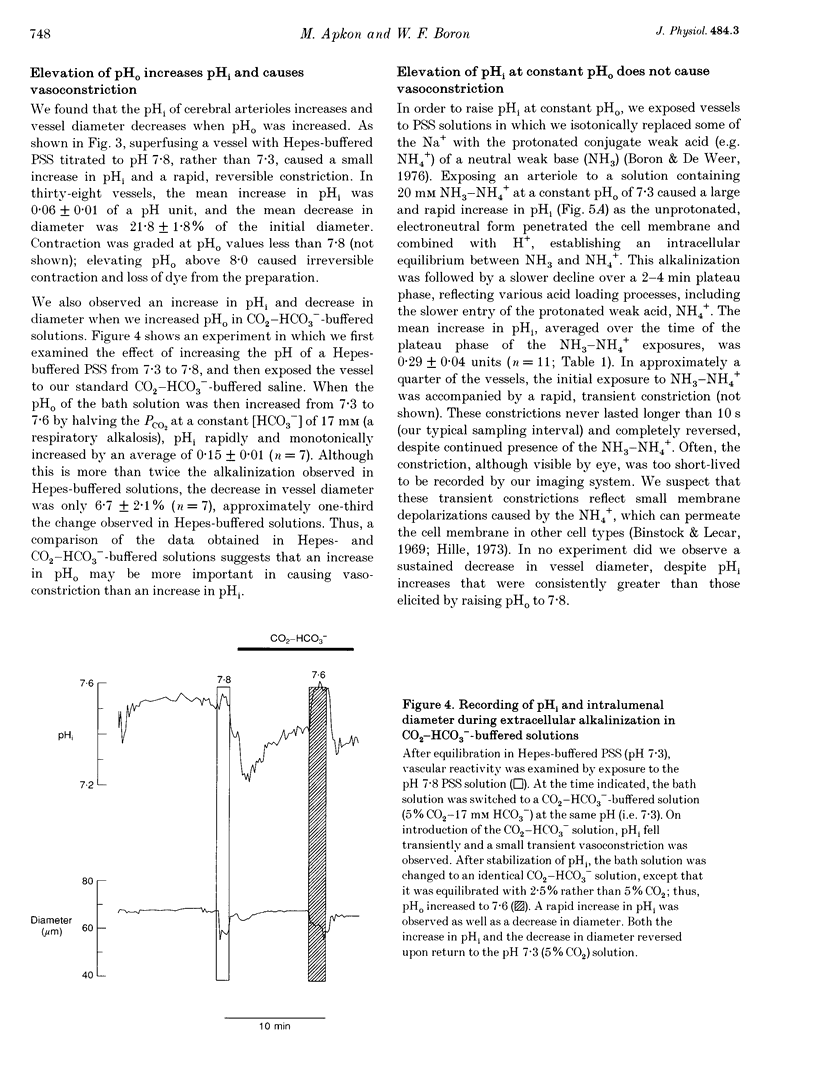
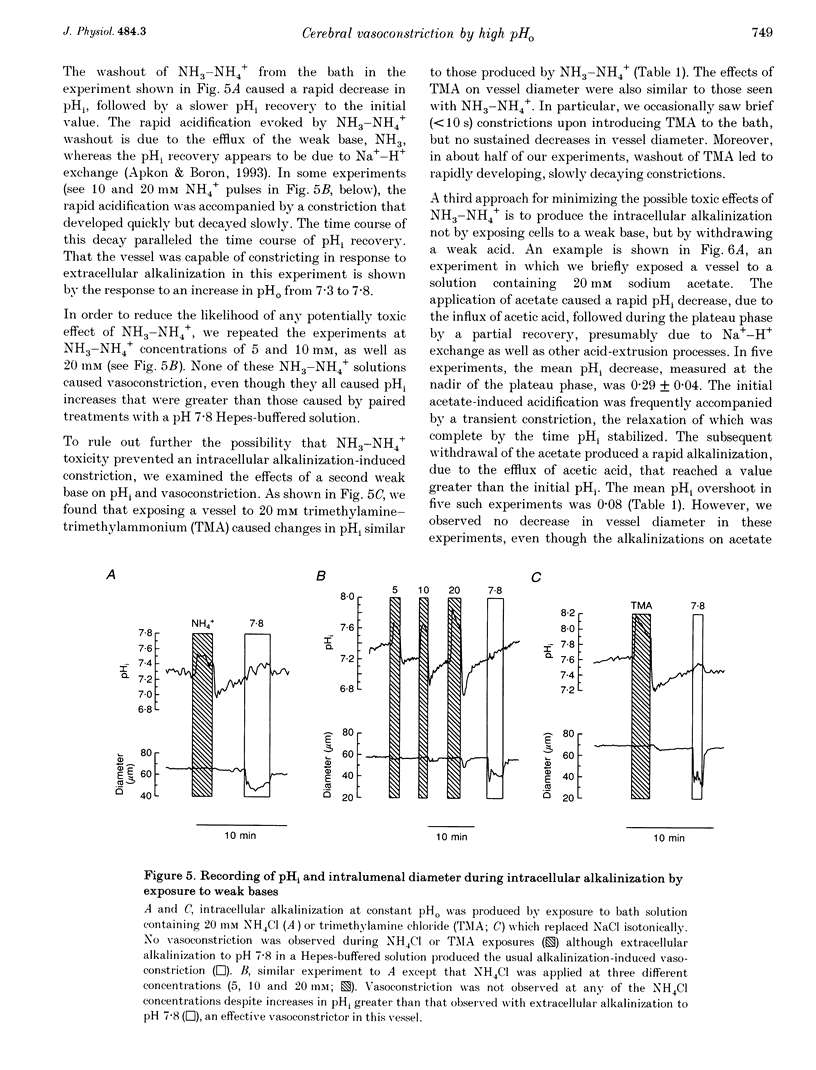
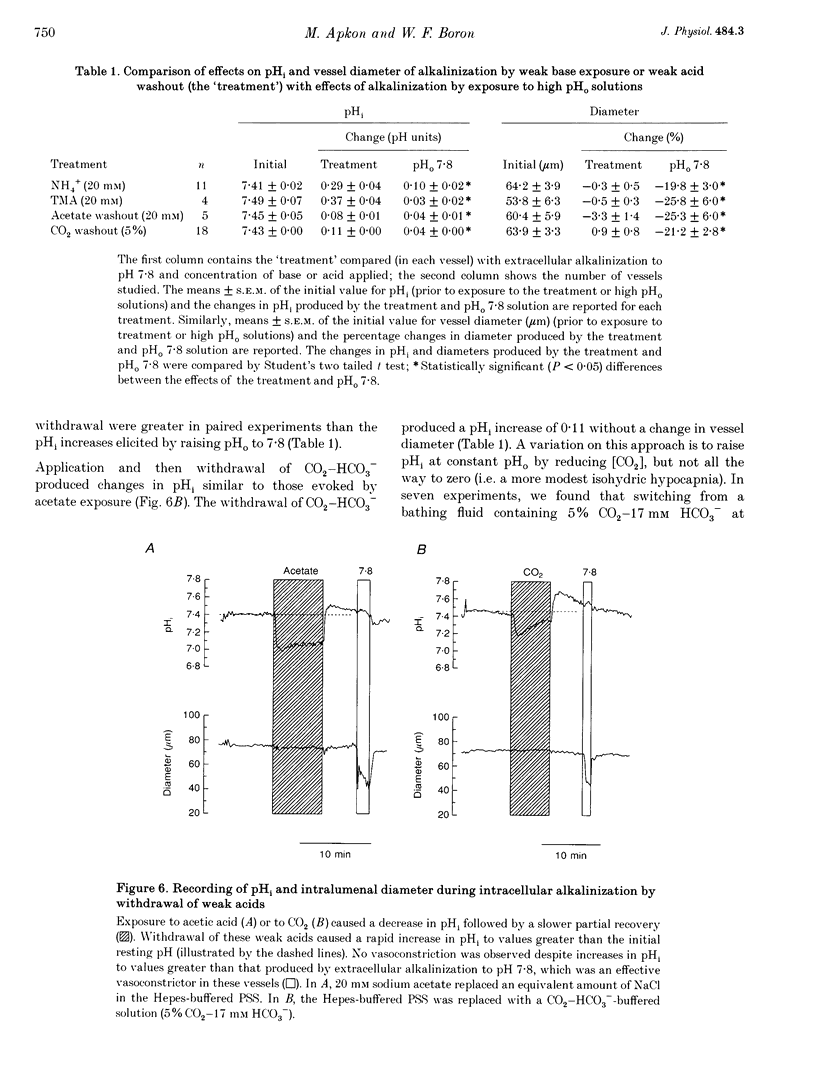
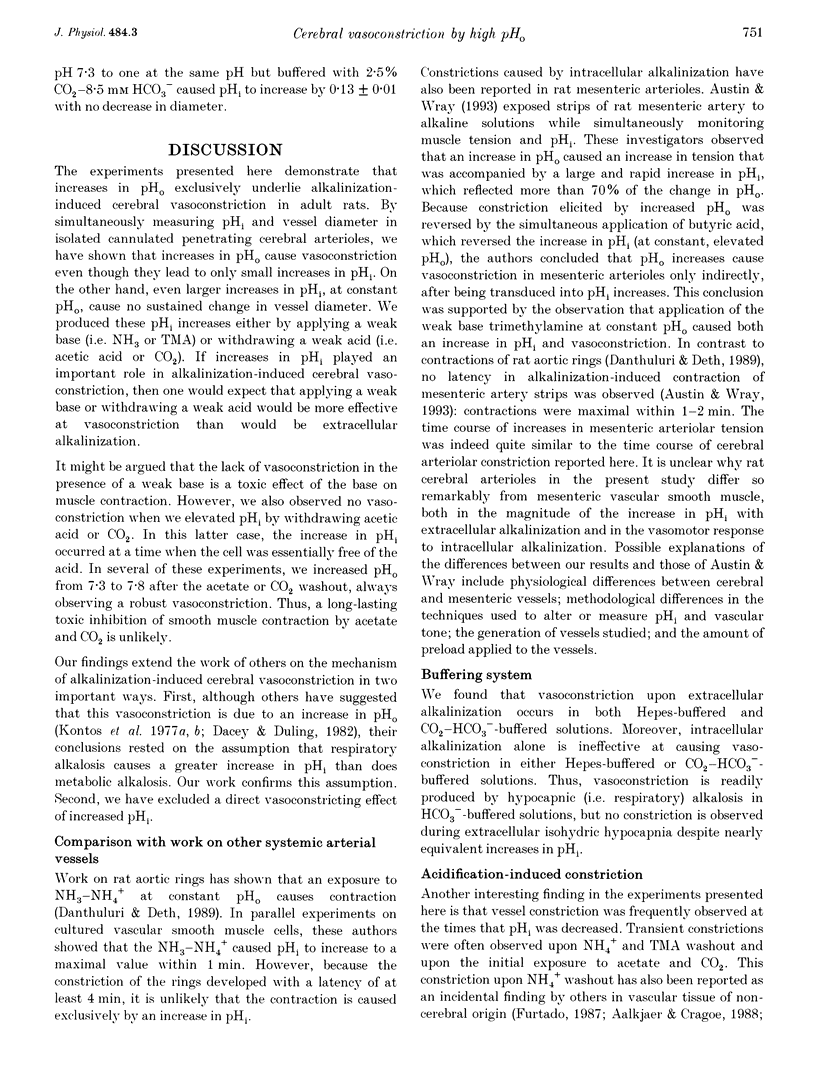
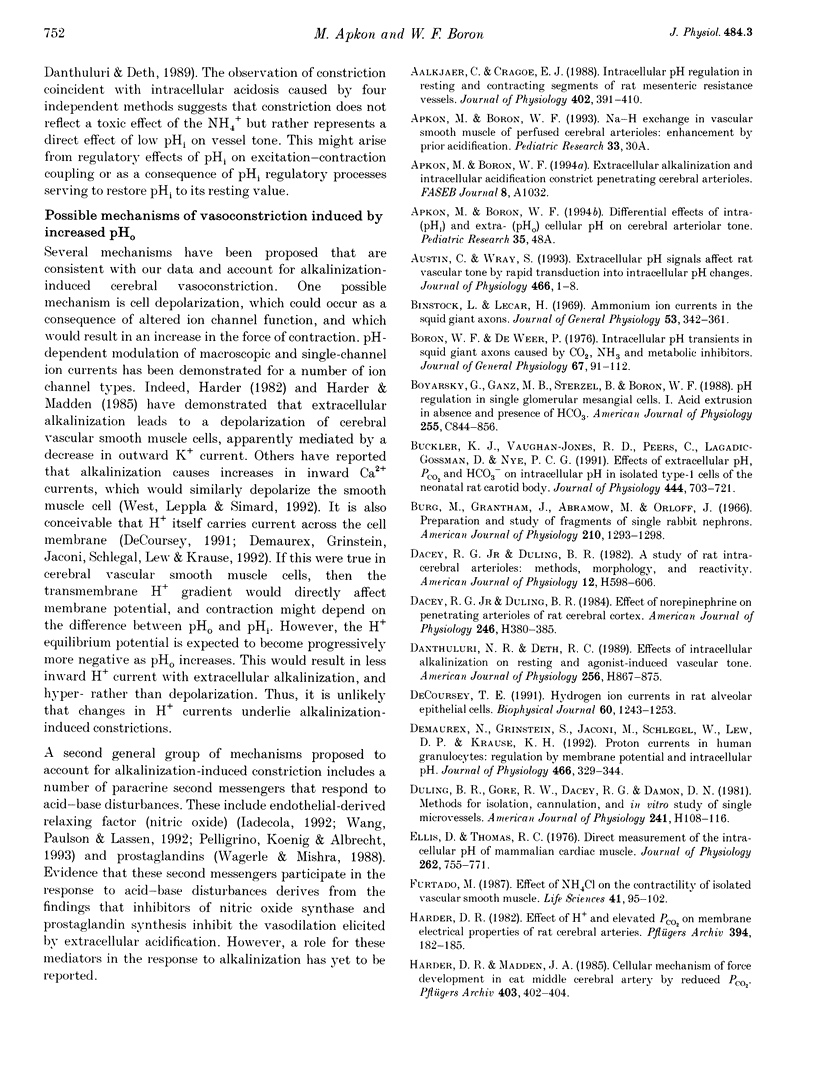
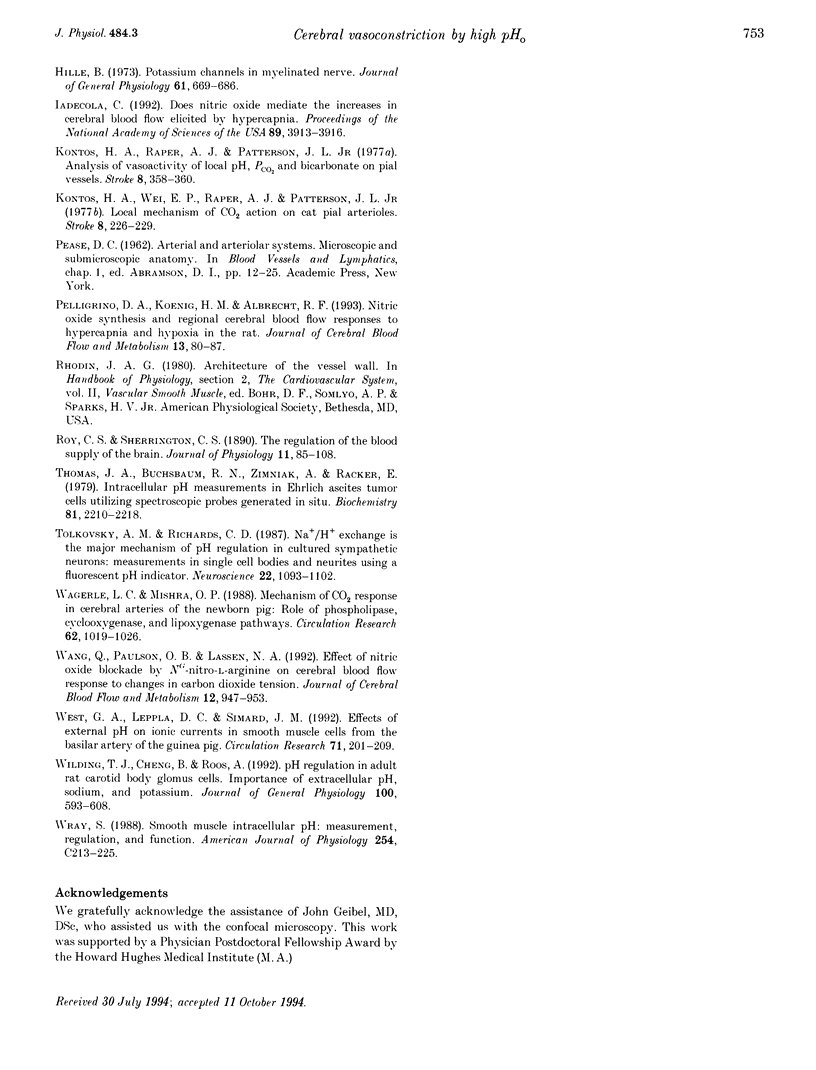
Images in this article
Selected References
These references are in PubMed. This may not be the complete list of references from this article.
- Aalkjaer C., Cragoe E. J., Jr Intracellular pH regulation in resting and contracting segments of rat mesenteric resistance vessels. J Physiol. 1988 Aug;402:391–410. doi: 10.1113/jphysiol.1988.sp017211. [DOI] [PMC free article] [PubMed] [Google Scholar]
- Austin C., Wray S. Extracellular pH signals affect rat vascular tone by rapid transduction into intracellular pH changes. J Physiol. 1993 Jul;466:1–8. [PMC free article] [PubMed] [Google Scholar]
- Binstock L., Lecar H. Ammonium ion currents in the squid giant axon. J Gen Physiol. 1969 Mar;53(3):342–361. doi: 10.1085/jgp.53.3.342. [DOI] [PMC free article] [PubMed] [Google Scholar]
- Boron W. F., De Weer P. Intracellular pH transients in squid giant axons caused by CO2, NH3, and metabolic inhibitors. J Gen Physiol. 1976 Jan;67(1):91–112. doi: 10.1085/jgp.67.1.91. [DOI] [PMC free article] [PubMed] [Google Scholar]
- Boyarsky G., Ganz M. B., Sterzel R. B., Boron W. F. pH regulation in single glomerular mesangial cells. I. Acid extrusion in absence and presence of HCO3-. Am J Physiol. 1988 Dec;255(6 Pt 1):C844–C856. doi: 10.1152/ajpcell.1988.255.6.C844. [DOI] [PubMed] [Google Scholar]
- Buckler K. J., Vaughan-Jones R. D., Peers C., Lagadic-Gossmann D., Nye P. C. Effects of extracellular pH, PCO2 and HCO3- on intracellular pH in isolated type-I cells of the neonatal rat carotid body. J Physiol. 1991 Dec;444:703–721. doi: 10.1113/jphysiol.1991.sp018902. [DOI] [PMC free article] [PubMed] [Google Scholar]
- Burg M., Grantham J., Abramow M., Orloff J. Preparation and study of fragments of single rabbit nephrons. Am J Physiol. 1966 Jun;210(6):1293–1298. doi: 10.1152/ajplegacy.1966.210.6.1293. [DOI] [PubMed] [Google Scholar]
- Dacey R. G., Jr, Duling B. R. A study of rat intracerebral arterioles: methods, morphology, and reactivity. Am J Physiol. 1982 Oct;243(4):H598–H606. doi: 10.1152/ajpheart.1982.243.4.H598. [DOI] [PubMed] [Google Scholar]
- Dacey R. G., Jr, Duling B. R. Effect of norepinephrine on penetrating arterioles of rat cerebral cortex. Am J Physiol. 1984 Mar;246(3 Pt 2):H380–H385. doi: 10.1152/ajpheart.1984.246.3.H380. [DOI] [PubMed] [Google Scholar]
- Danthuluri N. R., Deth R. C. Effects of intracellular alkalinization on resting and agonist-induced vascular tone. Am J Physiol. 1989 Mar;256(3 Pt 2):H867–H875. doi: 10.1152/ajpheart.1989.256.3.H867. [DOI] [PubMed] [Google Scholar]
- DeCoursey T. E. Hydrogen ion currents in rat alveolar epithelial cells. Biophys J. 1991 Nov;60(5):1243–1253. doi: 10.1016/S0006-3495(91)82158-0. [DOI] [PMC free article] [PubMed] [Google Scholar]
- Demaurex N., Grinstein S., Jaconi M., Schlegel W., Lew D. P., Krause K. H. Proton currents in human granulocytes: regulation by membrane potential and intracellular pH. J Physiol. 1993 Jul;466:329–344. [PMC free article] [PubMed] [Google Scholar]
- Duling B. R., Gore R. W., Dacey R. G., Jr, Damon D. N. Methods for isolation, cannulation, and in vitro study of single microvessels. Am J Physiol. 1981 Jul;241(1):H108–H116. doi: 10.1152/ajpheart.1981.241.1.H108. [DOI] [PubMed] [Google Scholar]
- Ellis D., Thomas R. C. Direct measurement of the intracellular pH of mammalian cardiac muscle. J Physiol. 1976 Nov;262(3):755–771. doi: 10.1113/jphysiol.1976.sp011619. [DOI] [PMC free article] [PubMed] [Google Scholar]
- Furtado M. R. Effect of NH4Cl on the contractility of isolated vascular smooth muscle. Life Sci. 1987 Jul 6;41(1):95–102. doi: 10.1016/0024-3205(87)90561-3. [DOI] [PubMed] [Google Scholar]
- Harder D. R. Effect of H+ and elevated PCO2 on membrane electrical properties of rat cerebral arteries. Pflugers Arch. 1982 Aug;394(2):182–185. doi: 10.1007/BF00582922. [DOI] [PubMed] [Google Scholar]
- Harder D. R., Madden J. A. Cellular mechanism of force development in cat middle cerebral artery by reduced PCO2. Pflugers Arch. 1985 Apr;403(4):402–406. doi: 10.1007/BF00589253. [DOI] [PubMed] [Google Scholar]
- Hille B. Potassium channels in myelinated nerve. Selective permeability to small cations. J Gen Physiol. 1973 Jun;61(6):669–686. doi: 10.1085/jgp.61.6.669. [DOI] [PMC free article] [PubMed] [Google Scholar]
- Iadecola C. Does nitric oxide mediate the increases in cerebral blood flow elicited by hypercapnia? Proc Natl Acad Sci U S A. 1992 May 1;89(9):3913–3916. doi: 10.1073/pnas.89.9.3913. [DOI] [PMC free article] [PubMed] [Google Scholar]
- Kontos H. A., Raper A. J., Patterson J. L. Analysis of vasoactivity of local pH, PCO2 and bicarbonate on pial vessels. Stroke. 1977 May-Jun;8(3):358–360. doi: 10.1161/01.str.8.3.358. [DOI] [PubMed] [Google Scholar]
- Kontos H. A., Wei E. P., Raper A. J., Patterson J. L., Jr Local mechanism of CO2 action of cat pial arterioles. Stroke. 1977 Mar-Apr;8(2):226–229. doi: 10.1161/01.str.8.2.226. [DOI] [PubMed] [Google Scholar]
- Pelligrino D. A., Koenig H. M., Albrecht R. F. Nitric oxide synthesis and regional cerebral blood flow responses to hypercapnia and hypoxia in the rat. J Cereb Blood Flow Metab. 1993 Jan;13(1):80–87. doi: 10.1038/jcbfm.1993.10. [DOI] [PubMed] [Google Scholar]
- Roy C. S., Sherrington C. S. On the Regulation of the Blood-supply of the Brain. J Physiol. 1890 Jan;11(1-2):85–158.17. doi: 10.1113/jphysiol.1890.sp000321. [DOI] [PMC free article] [PubMed] [Google Scholar]
- Thomas J. A., Buchsbaum R. N., Zimniak A., Racker E. Intracellular pH measurements in Ehrlich ascites tumor cells utilizing spectroscopic probes generated in situ. Biochemistry. 1979 May 29;18(11):2210–2218. doi: 10.1021/bi00578a012. [DOI] [PubMed] [Google Scholar]
- Tolkovsky A. M., Richards C. D. Na+/H+ exchange is the major mechanism of pH regulation in cultured sympathetic neurons: measurements in single cell bodies and neurites using a fluorescent pH indicator. Neuroscience. 1987 Sep;22(3):1093–1102. doi: 10.1016/0306-4522(87)92984-8. [DOI] [PubMed] [Google Scholar]
- Wagerle L. C., Mishra O. P. Mechanism of CO2 response in cerebral arteries of the newborn pig: role of phospholipase, cyclooxygenase, and lipoxygenase pathways. Circ Res. 1988 May;62(5):1019–1026. doi: 10.1161/01.res.62.5.1019. [DOI] [PubMed] [Google Scholar]
- Wang Q., Paulson O. B., Lassen N. A. Effect of nitric oxide blockade by NG-nitro-L-arginine on cerebral blood flow response to changes in carbon dioxide tension. J Cereb Blood Flow Metab. 1992 Nov;12(6):947–953. doi: 10.1038/jcbfm.1992.131. [DOI] [PubMed] [Google Scholar]
- West G. A., Leppla D. C., Simard J. M. Effects of external pH on ionic currents in smooth muscle cells from the basilar artery of the guinea pig. Circ Res. 1992 Jul;71(1):201–209. doi: 10.1161/01.res.71.1.201. [DOI] [PubMed] [Google Scholar]
- Wilding T. J., Cheng B., Roos A. pH regulation in adult rat carotid body glomus cells. Importance of extracellular pH, sodium, and potassium. J Gen Physiol. 1992 Oct;100(4):593–608. doi: 10.1085/jgp.100.4.593. [DOI] [PMC free article] [PubMed] [Google Scholar]