Abstract
The membrane potential (ΔΨ) and the pH gradient (ΔpH) across the membrane of the insulin-secretory granule were determined in studies in vitro from the uptake of the permeant anion thio[14C]cyanate or the permeant base [14C]methylamine. Freshly prepared granules incubated in iso-osmotic medium containing sucrose and low concentrations of buffer salts exhibited an acidic internal pH and a ΔΨ positive inside. Addition of MgATP2− under these conditions did not alter the ΔpH, but produced a marked increase in the ΔΨ. Conversely, when a permeant anion was also included, ATP produced a marked increase in the ΔpH and a lesser increment in the ΔΨ. NH4+ salts reduced the ΔpH across granule membranes. In the presence of ATP this effect was accompanied by a reciprocal increase in the ΔΨ. A similar reciprocity was evident when nigericin was added together with K+ or on decreasing the medium pH, suggesting that these gradients were linked by a common electrogenic process. The effects of ATP were reversed by the protonophore carbonyl cyanide p-trifluoromethoxyphenylhydrazone, the combination of valinomycin, nigericin and K+, and by the Mg2+-dependent ATPase inhibitor tributyltin. Uptakes of 14C-labelled tracer molecules were also markedly reduced by cryogenic disruption of the granule membrane or hypo-osmotic incubation conditions. These results were readily interpreted within a chemiosmotic hypothesis, which proposed that the insulin granules possess an inwardly-directed electrogenic proton-translocating Mg2+-dependent ATPase with the additional postulate that the membrane has a low proton permeability. The intragranular pH was estimated as being between 5 and 6 in vivo. Such a value corresponds to optimal conditions for the crystallization of zinc–insulin hexamers. Several other functions related to chemiosmotic processes within insulin granules, however, may be envisaged.
Full text
PDF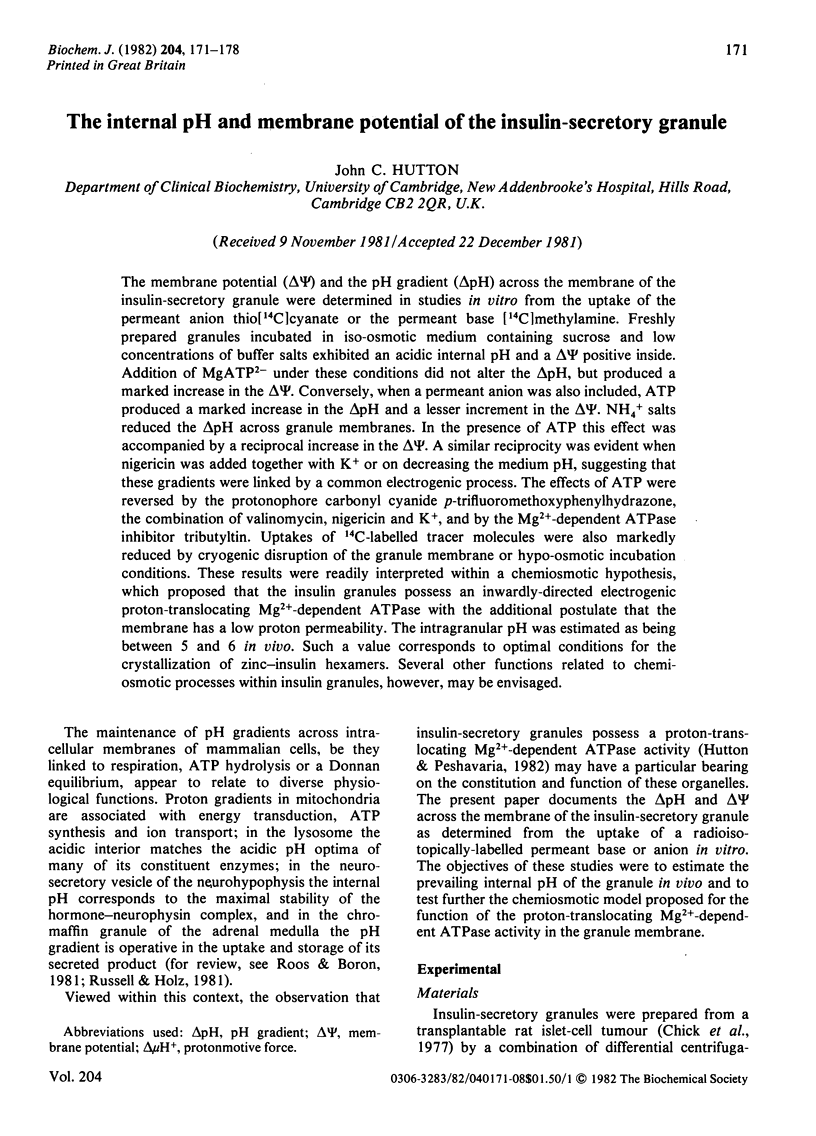
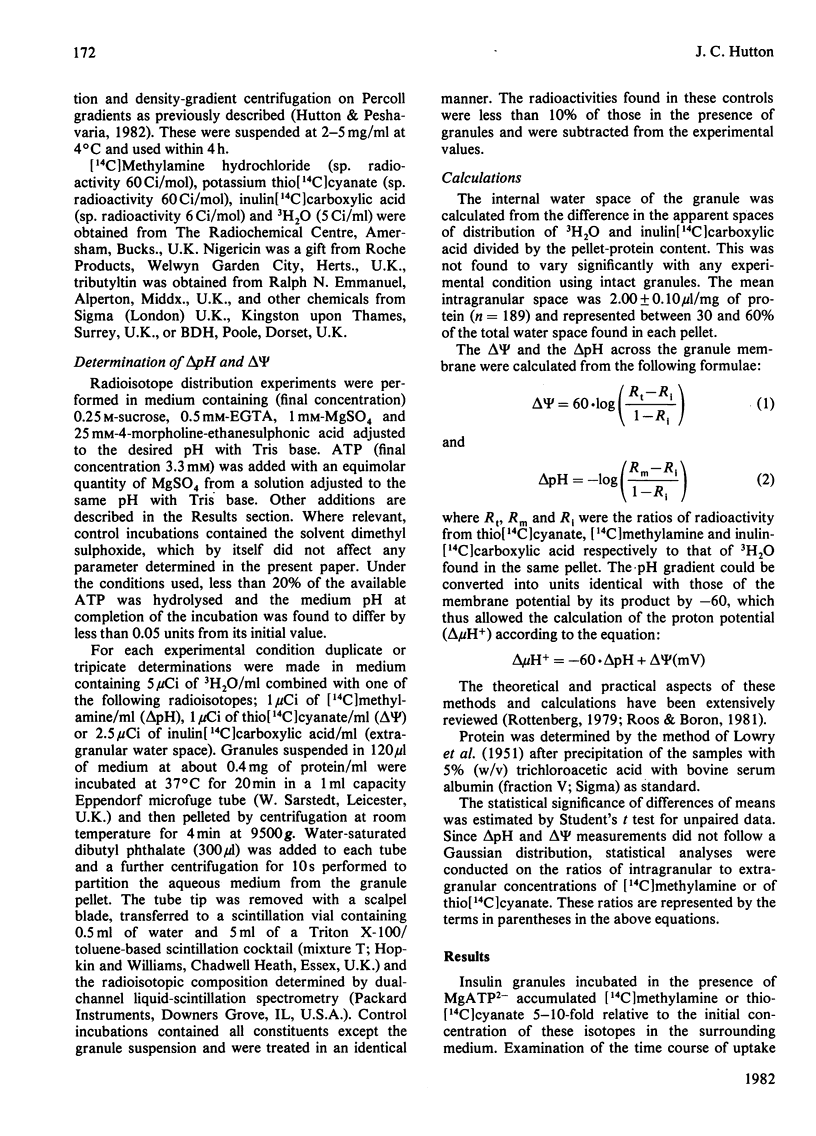
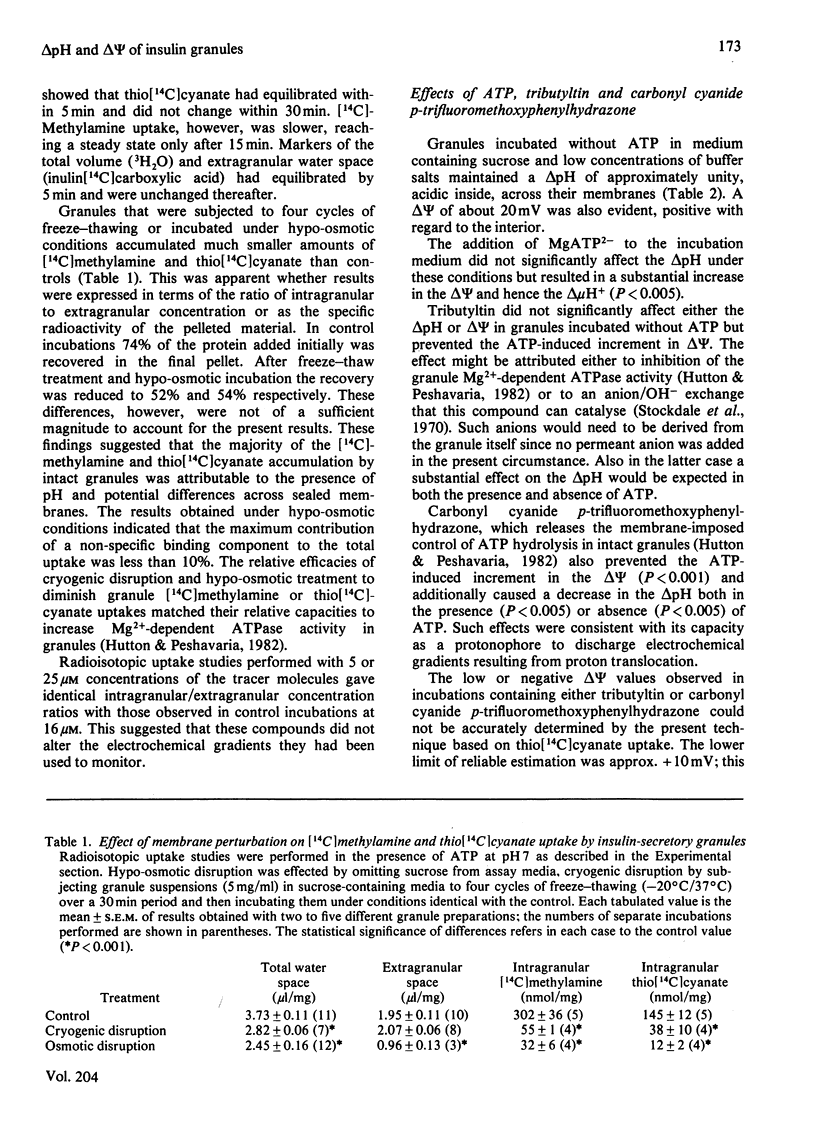
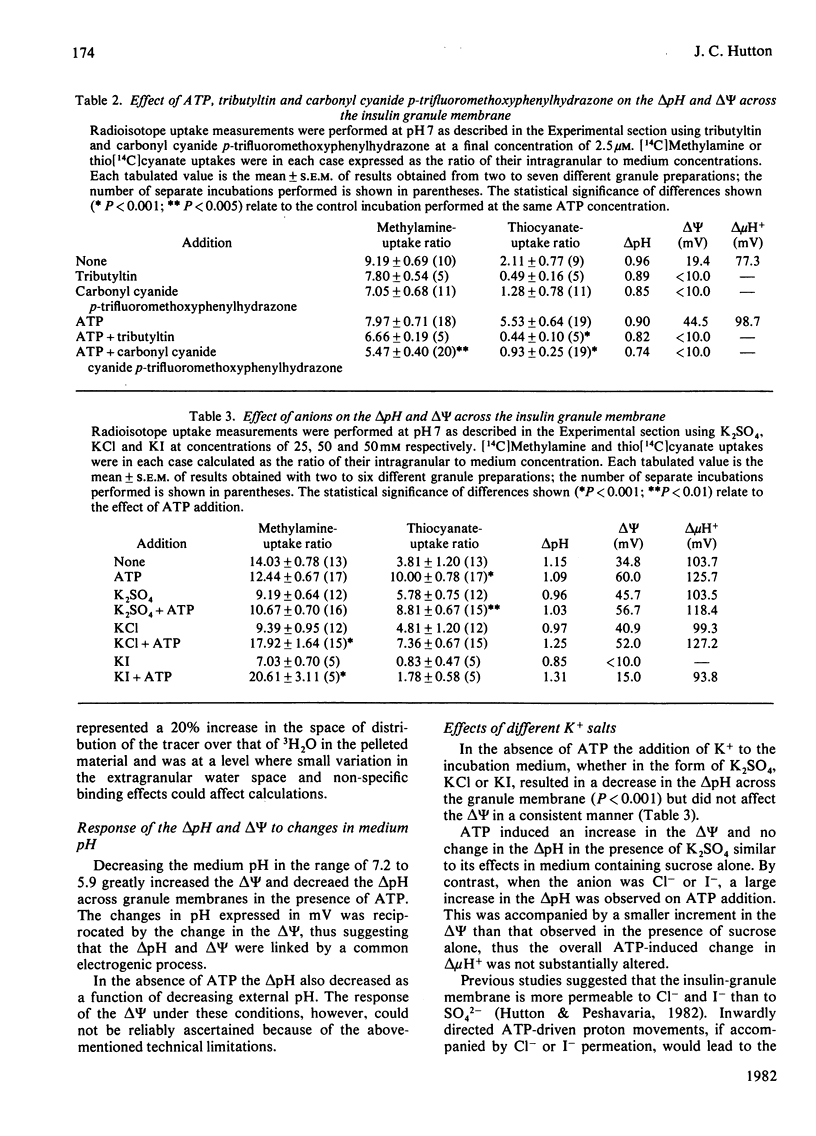
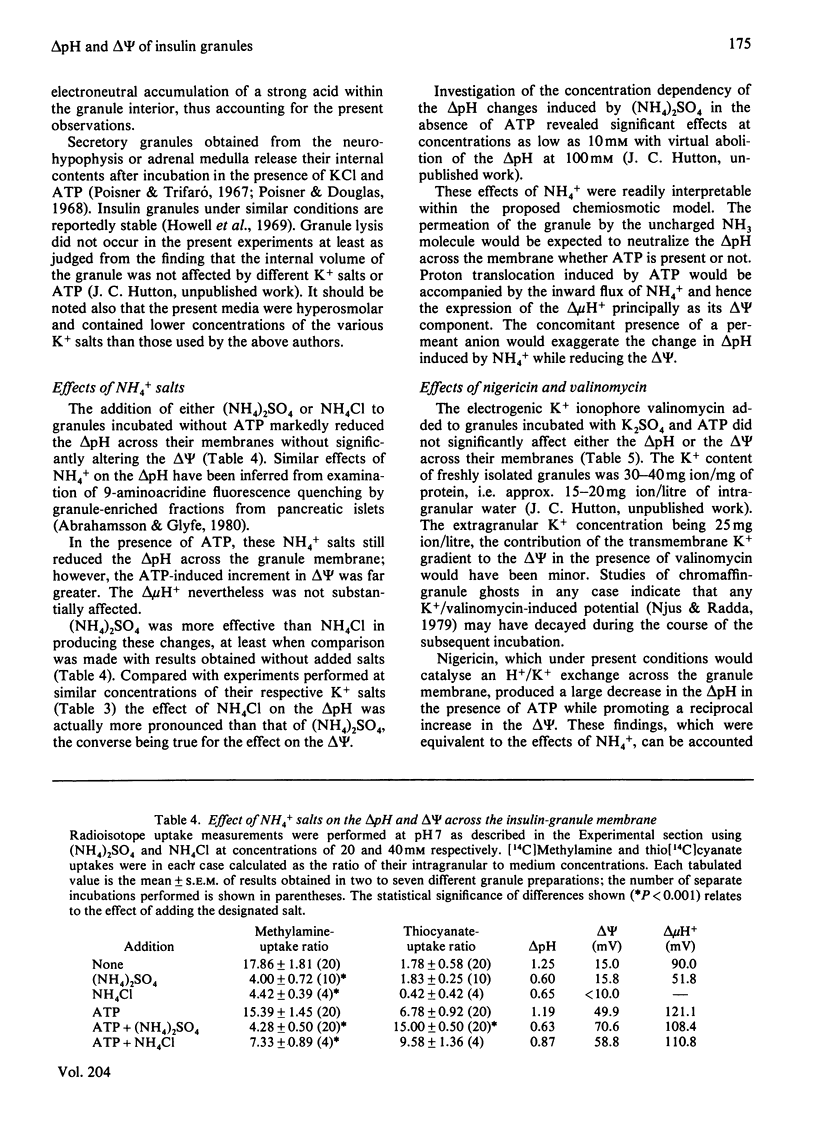
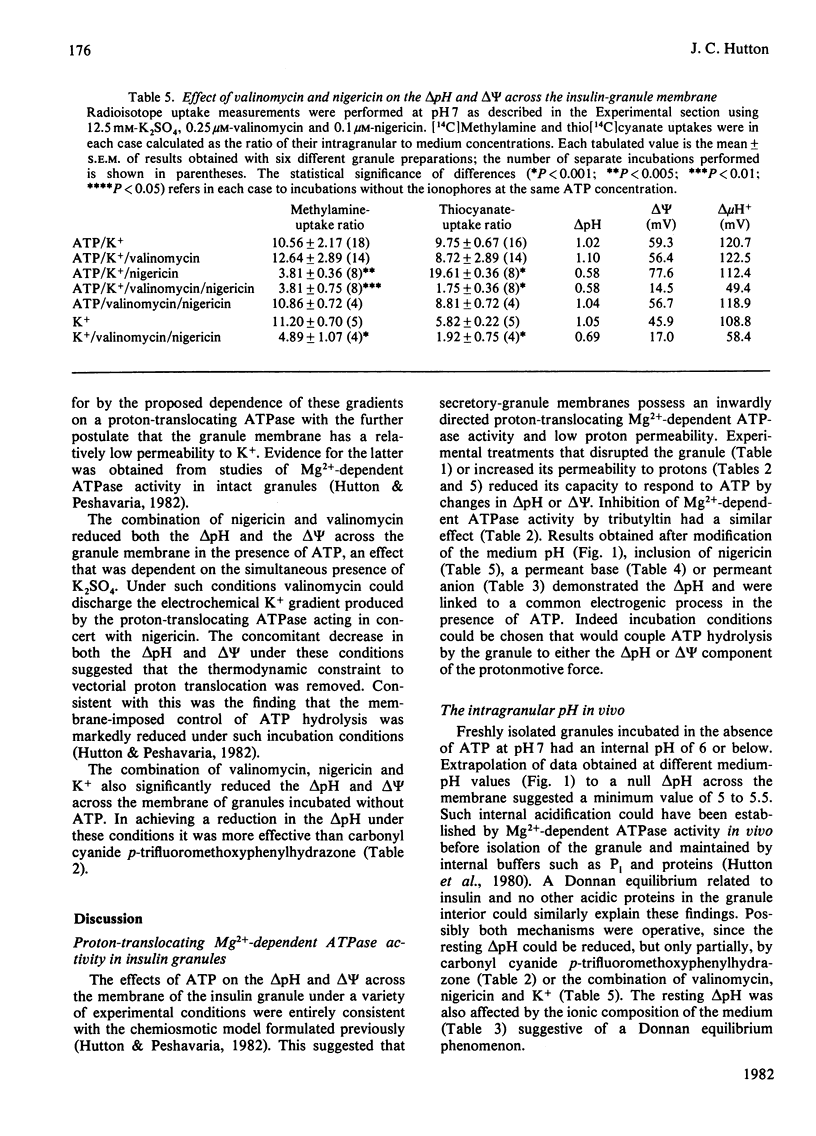
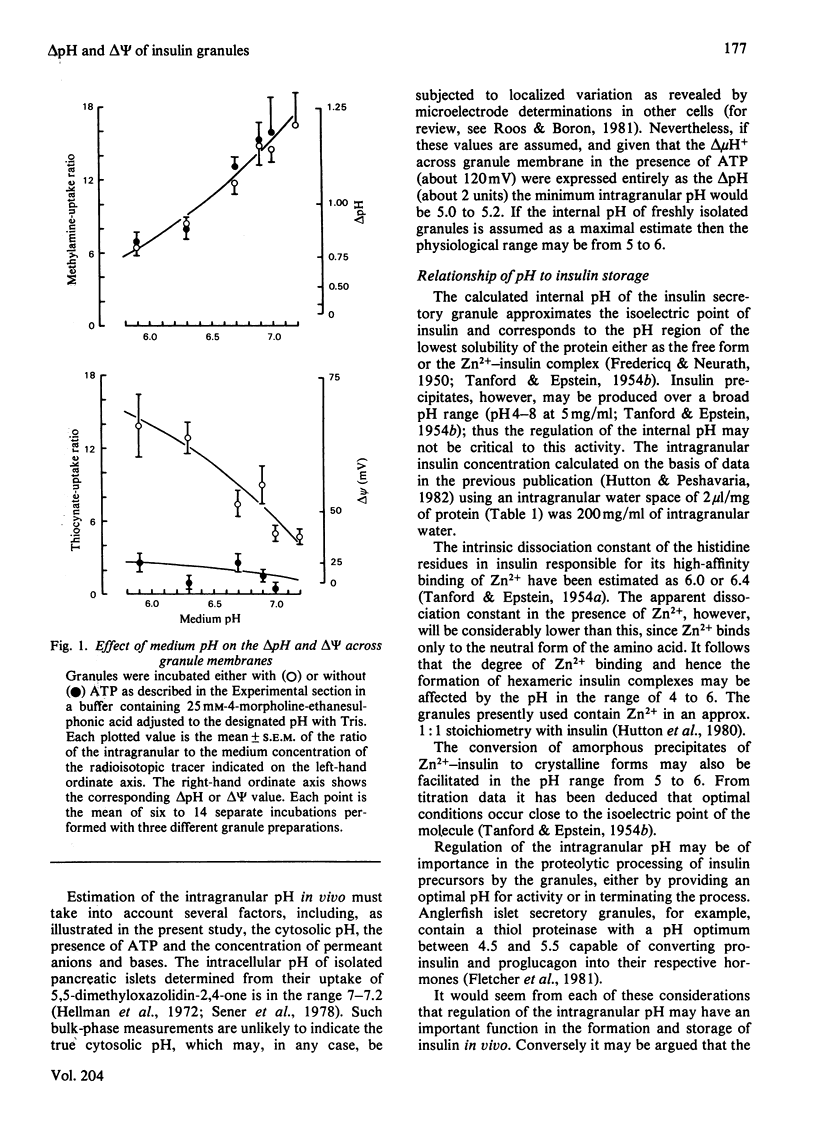
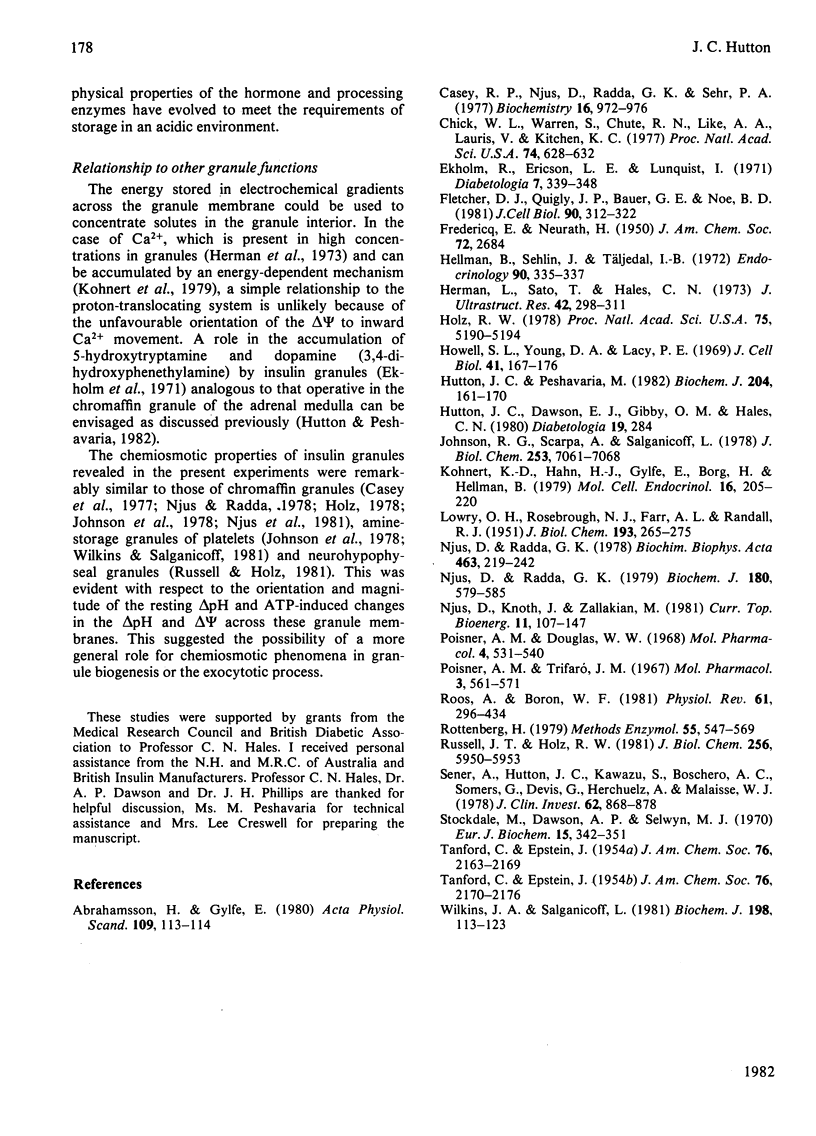
Selected References
These references are in PubMed. This may not be the complete list of references from this article.
- Abrahamsson H., Gylfe E. Demonstration of a proton gradient across the insulin granule membrane. Acta Physiol Scand. 1980 May;109(1):113–114. doi: 10.1111/j.1748-1716.1980.tb06573.x. [DOI] [PubMed] [Google Scholar]
- Casey R. P., Njus D., Radda G. K., Sehr P. A. Active proton uptake by chromaffin granules: observation by amine distribution and phosphorus-31 nuclear magnetic resonance techniques. Biochemistry. 1977 Mar 8;16(5):972–977. doi: 10.1021/bi00624a025. [DOI] [PubMed] [Google Scholar]
- Chick W. L., Warren S., Chute R. N., Like A. A., Lauris V., Kitchen K. C. A transplantable insulinoma in the rat. Proc Natl Acad Sci U S A. 1977 Feb;74(2):628–632. doi: 10.1073/pnas.74.2.628. [DOI] [PMC free article] [PubMed] [Google Scholar]
- Duggan D. E., Pua K. H., Elfenbein G. Purine metabolism in the chick embryo; effects of uricogenesis and xanthine oxidase inhibition. Mol Pharmacol. 1968 Jan;4(1):53–60. [PubMed] [Google Scholar]
- Ekholm R., Ericson L. E., Lundquist I. Monoamines in the pancreatic islets of the mouse. Subcellular localization of 5-hydroxytryptamine by electron microscopic autoradiography. Diabetologia. 1971 Oct;7(5):339–348. doi: 10.1007/BF01219468. [DOI] [PubMed] [Google Scholar]
- Fletcher D. J., Quigley J. P., Bauer G. E., Noe B. D. Characterization of proinsulin- and proglucagon-converting activities in isolated islet secretory granules. J Cell Biol. 1981 Aug;90(2):312–322. doi: 10.1083/jcb.90.2.312. [DOI] [PMC free article] [PubMed] [Google Scholar]
- Herman L., Sato T., Hales C. N. The electron microscopic localization of cations to pancreatic islets of Langerhans and their possible tole in insulin secretion. J Ultrastruct Res. 1973 Feb;42(3):298–311. doi: 10.1016/s0022-5320(73)90058-0. [DOI] [PubMed] [Google Scholar]
- Holz R. W. Evidence that catecholamine transport into chromaffin vesicles is coupled to vesicle membrane potential. Proc Natl Acad Sci U S A. 1978 Oct;75(10):5190–5194. doi: 10.1073/pnas.75.10.5190. [DOI] [PMC free article] [PubMed] [Google Scholar]
- Howell S. L., Young D. A., Lacy P. E. Isolation and properties of secretory granules from rat islets of Langerhans. 3. Studies of the stability of the isolated beta granules. J Cell Biol. 1969 Apr;41(1):167–176. doi: 10.1083/jcb.41.1.167. [DOI] [PMC free article] [PubMed] [Google Scholar]
- Hutton J. C., Peshavaria M. Proton-translocating Mg2+-dependent ATPase activity in insulin-secretory granules. Biochem J. 1982 Apr 15;204(1):161–170. doi: 10.1042/bj2040161. [DOI] [PMC free article] [PubMed] [Google Scholar]
- Johnson R. G., Scarpa A., Salganicoff L. The internal pH of isolated serotonin containing granules of pig platelets. J Biol Chem. 1978 Oct 10;253(19):7061–7068. [PubMed] [Google Scholar]
- Kohnert K. D., Hahn H. J., Gylfe E., Borg H., Hellman B. Calcium and pancreatic beta-cell function. 6. Glucose and intracellular 45Ca distribution. Mol Cell Endocrinol. 1979 Dec;16(3):205–220. doi: 10.1016/0303-7207(79)90027-3. [DOI] [PubMed] [Google Scholar]
- Njus D., Radda G. K. A potassium ion diffusion potential causes adrenaline uptake in chromaffin-granule 'ghosts'. Biochem J. 1979 Jun 15;180(3):579–585. doi: 10.1042/bj1800579. [DOI] [PMC free article] [PubMed] [Google Scholar]
- Njus D., Radda G. K. Bioenergetic processes in chromaffin granules a new perspective on some old problems. Biochim Biophys Acta. 1978 Mar 10;463(3-4):219–244. doi: 10.1016/0304-4173(78)90001-0. [DOI] [PubMed] [Google Scholar]
- Poisner A. M., Trifaró J. M. The role of ATP and ATPase in the release of catecholamines from the adrenal medulla. I. ATP-evoked release of catecholamines, ATP, and protein from isolated chromaffin granules. Mol Pharmacol. 1967 Nov;3(6):561–571. [PubMed] [Google Scholar]
- Roos A., Boron W. F. Intracellular pH. Physiol Rev. 1981 Apr;61(2):296–434. doi: 10.1152/physrev.1981.61.2.296. [DOI] [PubMed] [Google Scholar]
- Rottenberg H. The measurement of membrane potential and deltapH in cells, organelles, and vesicles. Methods Enzymol. 1979;55:547–569. doi: 10.1016/0076-6879(79)55066-6. [DOI] [PubMed] [Google Scholar]
- Russell J. T., Holz R. W. Measurement of delta pH and membrane potential in isolated neurosecretory vesicles from bovine neurohypophyses. J Biol Chem. 1981 Jun 25;256(12):5950–5953. [PubMed] [Google Scholar]
- Sener A., Hutton J. C., Kawazu S., Boschero A. C., Somers G., Devis G., Herchuelz A., Malaisse W. J. The stimulus-secretion coupling of glucose-induced insulin release. Metabolic and functional effects of NH4+ in rat islets. J Clin Invest. 1978 Oct;62(4):868–878. doi: 10.1172/JCI109199. [DOI] [PMC free article] [PubMed] [Google Scholar]
- Stockdale M., Dawson A. P., Selwyn M. J. Effects of trialkyltin and triphenyltin compounds on mitochondrial respiration. Eur J Biochem. 1970 Aug;15(2):342–351. doi: 10.1111/j.1432-1033.1970.tb01013.x. [DOI] [PubMed] [Google Scholar]
- Wilkins J. A., Salganicoff L. Participation of a transmembrane proton gradient in 5-hydroxytryptamine transport by platelet dense granules and dense-granule ghosts. Biochem J. 1981 Jul 15;198(1):113–123. doi: 10.1042/bj1980113. [DOI] [PMC free article] [PubMed] [Google Scholar]