Abstract
1. Excitatory postsynaptic currents (EPSCs) were recorded in rat submandibular ganglion cells in vitro using the two-electrode voltage clamp technique. 2. The peak amplitude of EPSCs evoked by nerve impulses in single presynaptic fibres varied between 1.2 and 9.8 nA in different cells (mean = 4.6 +/- 2.6 nA; n = 23; -80 mV membrane potential; 22-25 degrees C). 3. Experiments were performed to re-investigate a previous hypothesis that different mechanisms underlie the generation of evoked versus spontaneous quantal EPSCs in submandibular cells. This hypothesis was based on the observation of different time courses of evoked and spontaneous EPSCs. 4. In agreement with previous studies, the time course of the decay phase of evoked EPSCs was described by the sum of two exponentials, with time constants tau 1 and tau 2 of 6.9 +/- 0.7 and 34.4 +/- 7.7 ms, respectively (n = 23; -80 mV membrane potential). 5. The double-exponential decay of evoked EPSCs persisted when transmitter release was reduced by bath addition of 100 microM cadmium chloride to the level of failures, one or several quanta. 6. Spontaneous EPSCs exhibited mean amplitudes of 81 +/- 24 pA (n = 5 cells; -80 mV membrane potential), and displayed an extremely wide range of peak amplitudes in the same cell (mean coefficient of variation (c.v.) = 0.37 +/- 0.09; n = 5 cells). In contrast to a previous report (see below), the decay phase of spontaneous EPSCs was found to exhibit a double-exponential time course with time constants similar to those of the evoked EPSC recorded in the same cell. 7. These results indicate that evoked and spontaneously released quanta of transmitter most probably act on the same population of postsynaptic receptors in submandibular ganglion cells. There is a large variability in the peak amplitudes of quantal EPSCs recorded in the same cell. This large variability is not due to electrotonic effects, since these cells lack dendrites.
Full text
PDF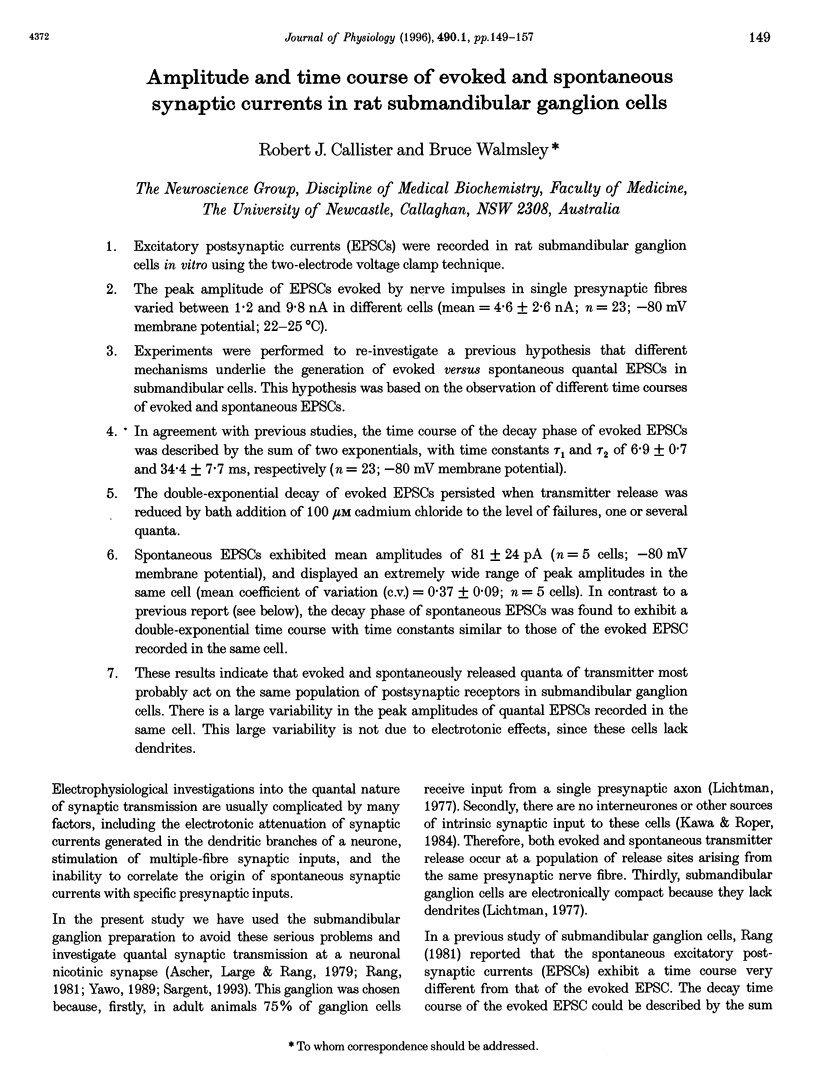
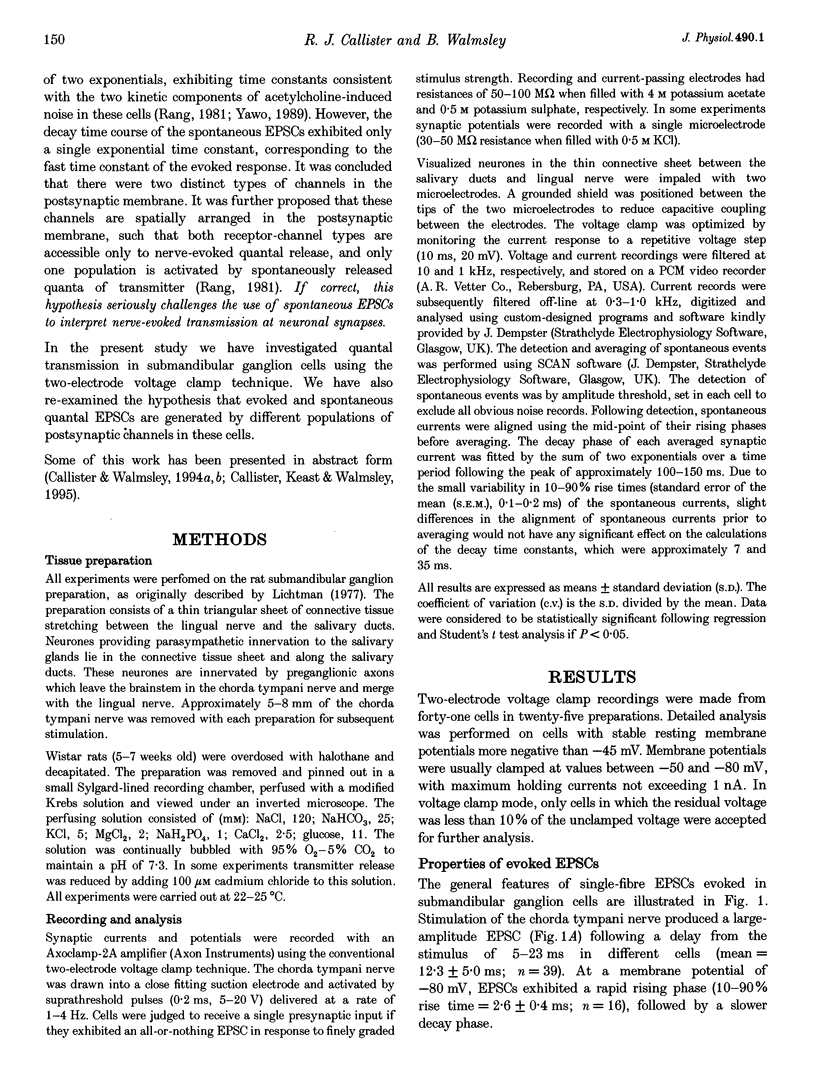
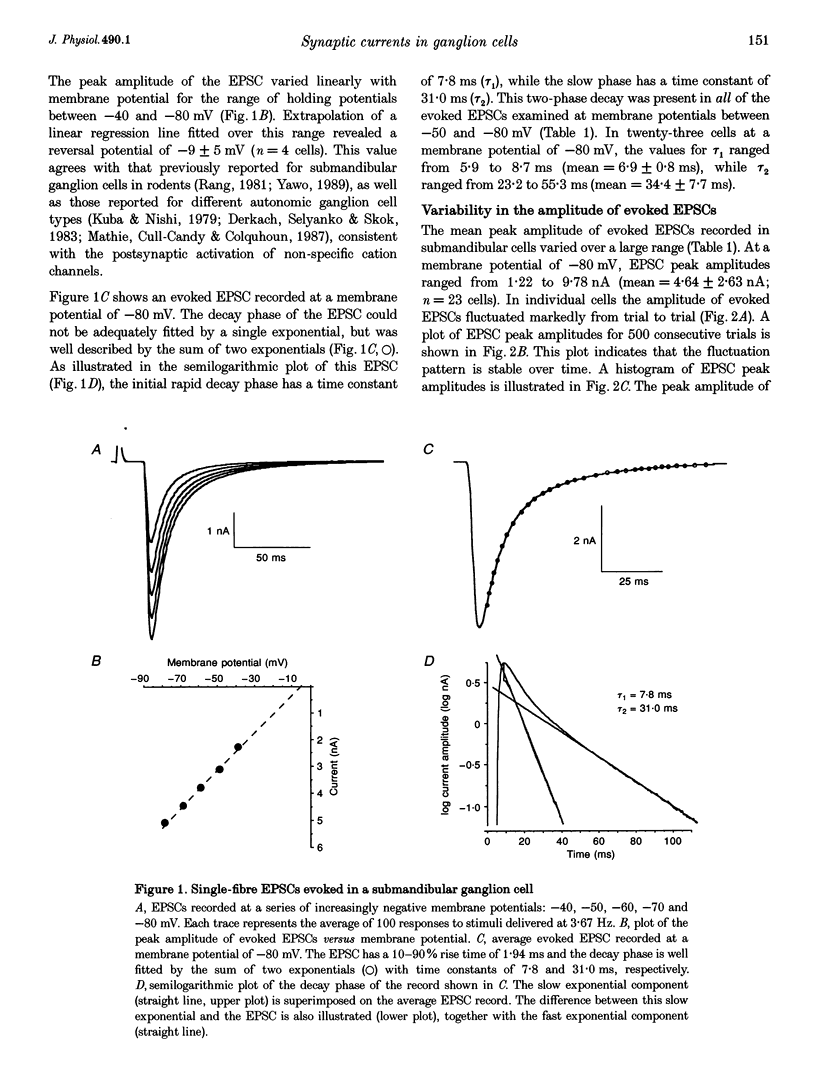
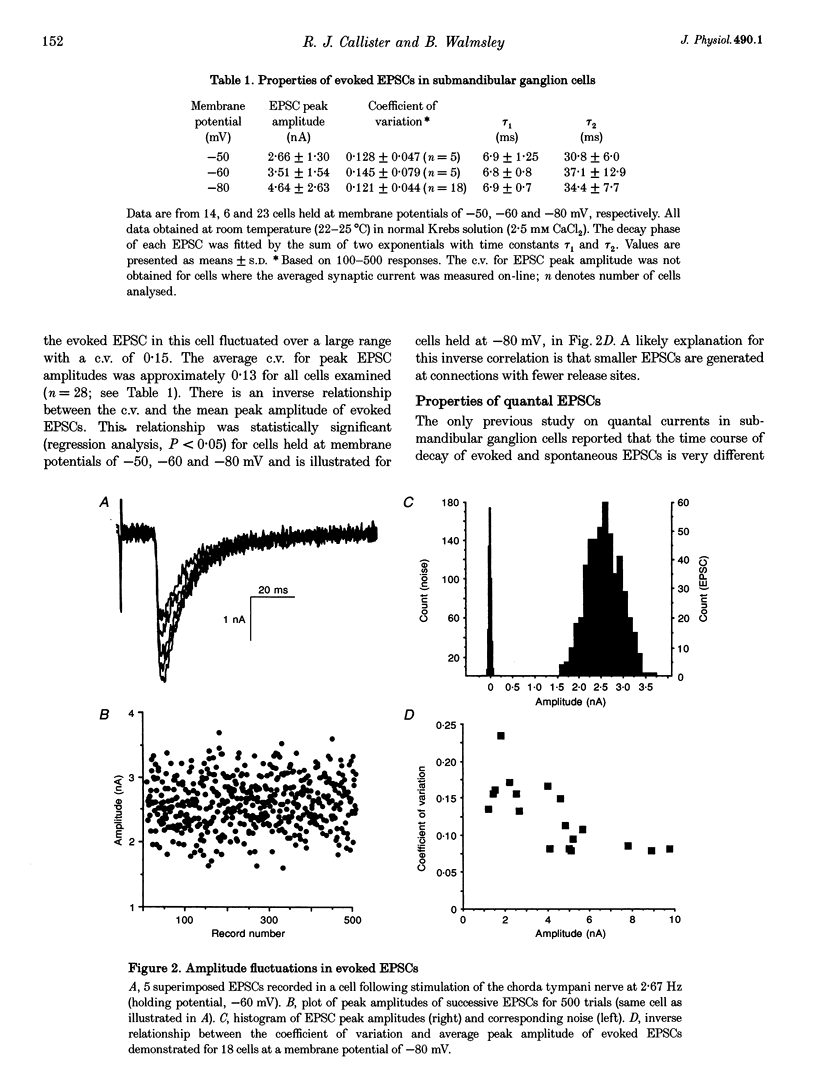
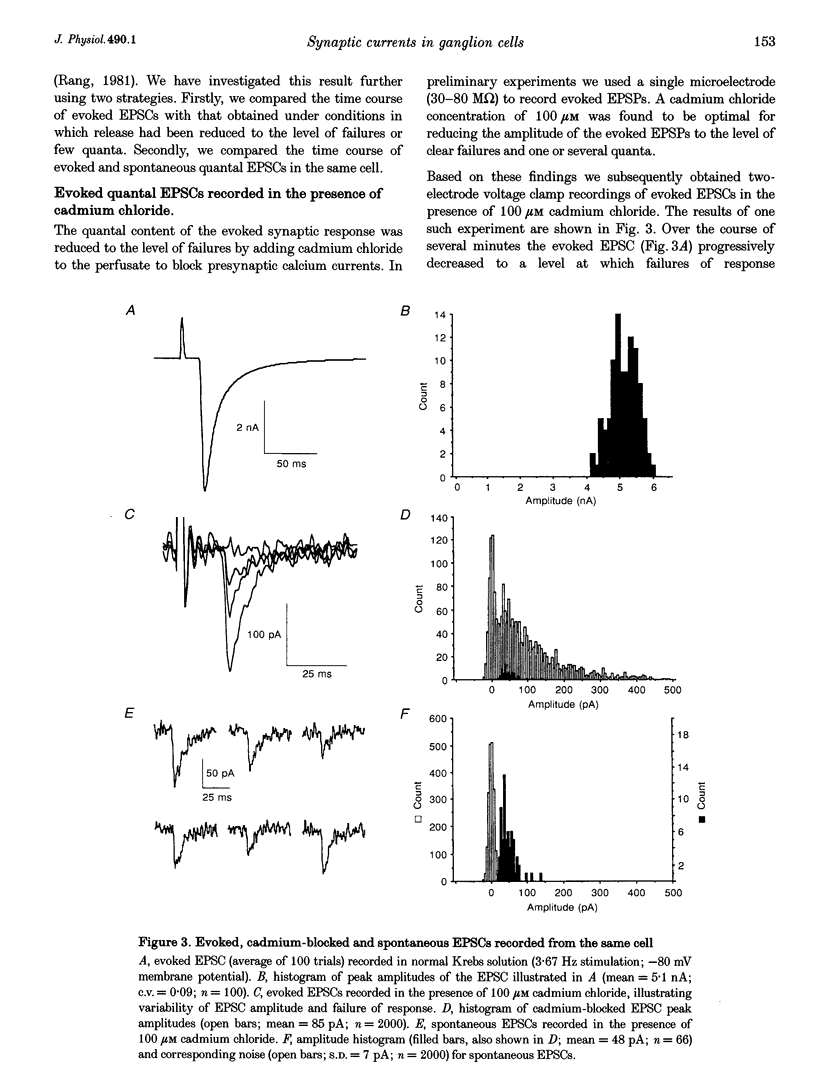
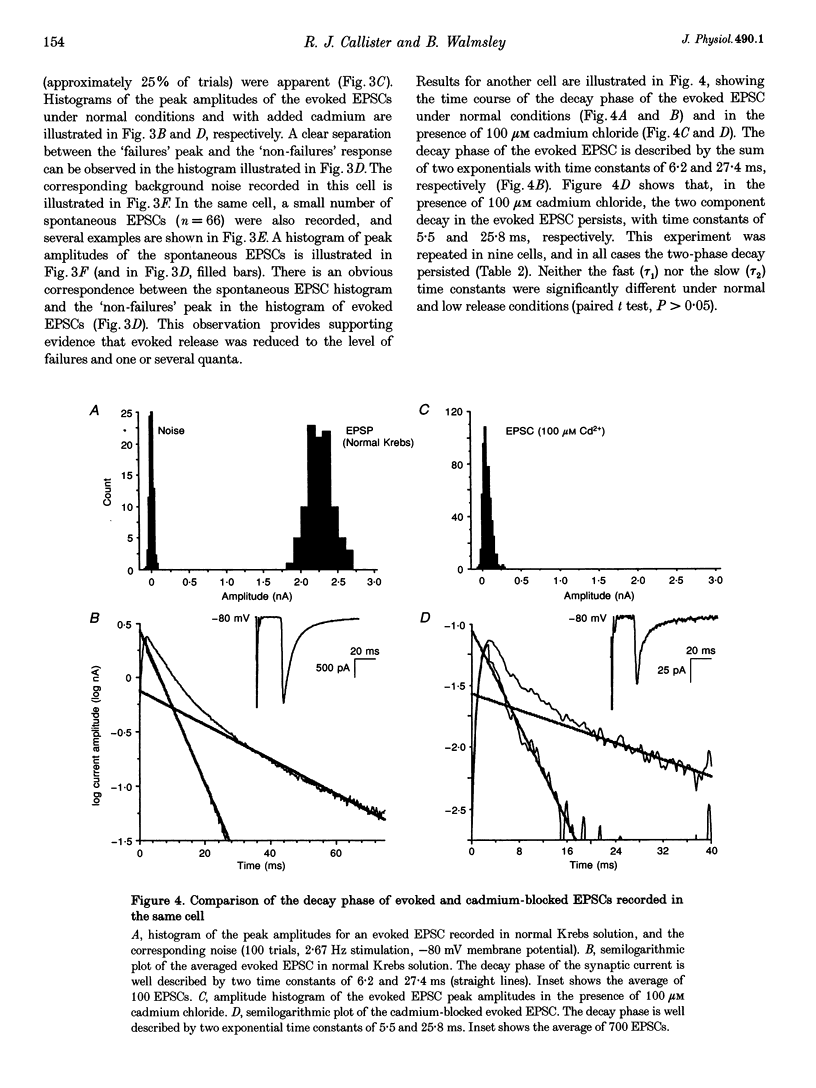
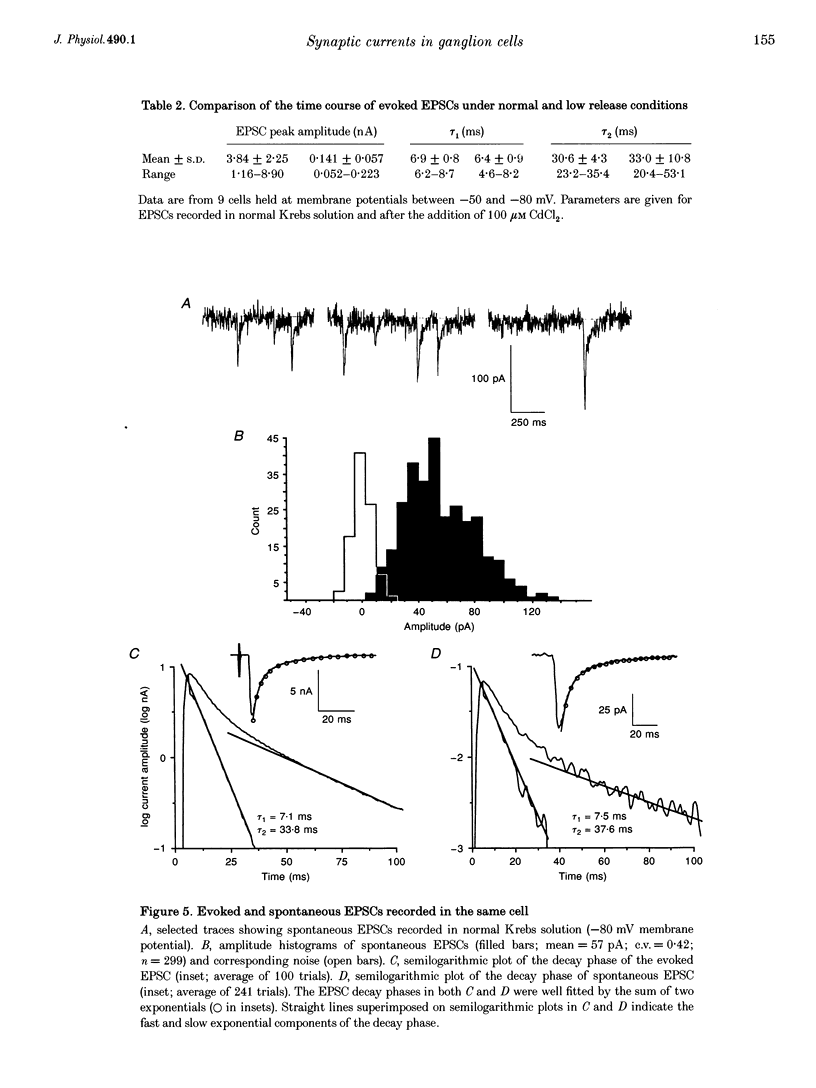
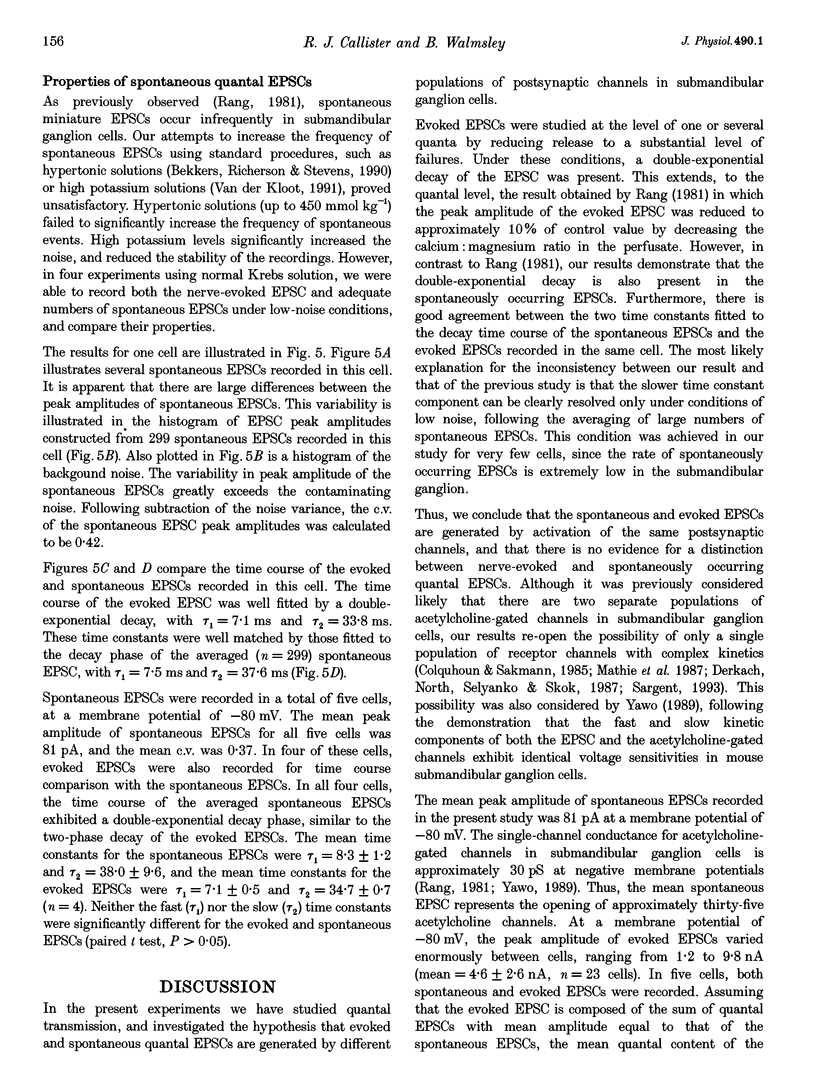
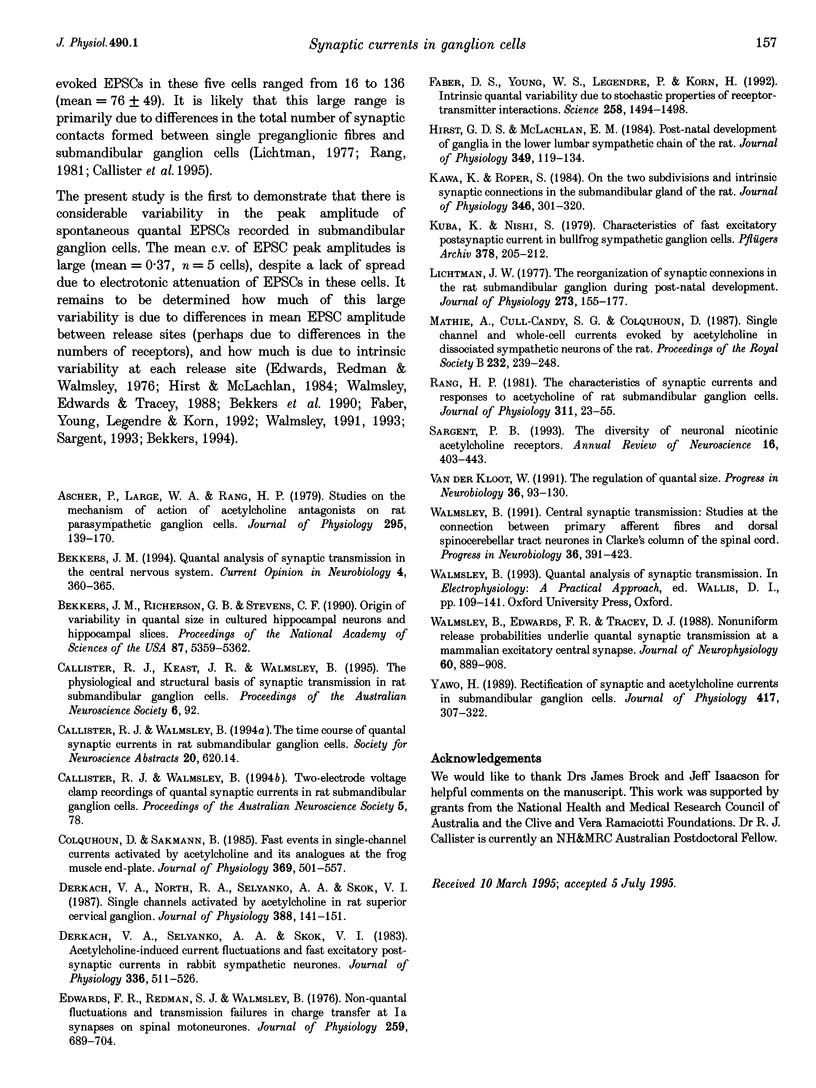
Selected References
These references are in PubMed. This may not be the complete list of references from this article.
- Ascher P., Large W. A., Rang H. P. Studies on the mechanism of action of acetylcholine antagonists on rat parasympathetic ganglion cells. J Physiol. 1979 Oct;295:139–170. doi: 10.1113/jphysiol.1979.sp012958. [DOI] [PMC free article] [PubMed] [Google Scholar]
- Bekkers J. M. Quantal analysis of synaptic transmission in the central nervous system. Curr Opin Neurobiol. 1994 Jun;4(3):360–365. doi: 10.1016/0959-4388(94)90097-3. [DOI] [PubMed] [Google Scholar]
- Bekkers J. M., Richerson G. B., Stevens C. F. Origin of variability in quantal size in cultured hippocampal neurons and hippocampal slices. Proc Natl Acad Sci U S A. 1990 Jul;87(14):5359–5362. doi: 10.1073/pnas.87.14.5359. [DOI] [PMC free article] [PubMed] [Google Scholar]
- Colquhoun D., Sakmann B. Fast events in single-channel currents activated by acetylcholine and its analogues at the frog muscle end-plate. J Physiol. 1985 Dec;369:501–557. doi: 10.1113/jphysiol.1985.sp015912. [DOI] [PMC free article] [PubMed] [Google Scholar]
- Derkach V. A., North R. A., Selyanko A. A., Skok V. I. Single channels activated by acetylcholine in rat superior cervical ganglion. J Physiol. 1987 Jul;388:141–151. doi: 10.1113/jphysiol.1987.sp016606. [DOI] [PMC free article] [PubMed] [Google Scholar]
- Derkach V. A., Selyanko A. A., Skok V. I. Acetylcholine-induced current fluctuations and fast excitatory post-synaptic currents in rabbit sympathetic neurones. J Physiol. 1983 Mar;336:511–526. doi: 10.1113/jphysiol.1983.sp014595. [DOI] [PMC free article] [PubMed] [Google Scholar]
- Edwards F. R., Redman S. J., Walmsley B. Non-quantal fluctuations and transmission failures in charge transfer at Ia synapses on spinal motoneurones. J Physiol. 1976 Aug;259(3):689–704. doi: 10.1113/jphysiol.1976.sp011489. [DOI] [PMC free article] [PubMed] [Google Scholar]
- Faber D. S., Young W. S., Legendre P., Korn H. Intrinsic quantal variability due to stochastic properties of receptor-transmitter interactions. Science. 1992 Nov 27;258(5087):1494–1498. doi: 10.1126/science.1279813. [DOI] [PubMed] [Google Scholar]
- Kawa K., Roper S. On the two subdivisions and intrinsic synaptic connexions in the submandibular ganglion of the rat. J Physiol. 1984 Jan;346:301–320. doi: 10.1113/jphysiol.1984.sp015023. [DOI] [PMC free article] [PubMed] [Google Scholar]
- Kuba K., Nishi S. Characteristics of fast excitatory postsynaptic current in bullfrog sympathetic ganglion cells. Effects of membrane potential, temperature and Ca ions. Pflugers Arch. 1979 Jan 31;378(3):205–212. doi: 10.1007/BF00592737. [DOI] [PubMed] [Google Scholar]
- Lichtman J. W. The reorganization of synaptic connexions in the rat submandibular ganglion during post-natal development. J Physiol. 1977 Dec;273(1):155–177. doi: 10.1113/jphysiol.1977.sp012087. [DOI] [PMC free article] [PubMed] [Google Scholar]
- Mathie A., Cull-Candy S. G., Colquhoun D. Single-channel and whole-cell currents evoked by acetylcholine in dissociated sympathetic neurons of the rat. Proc R Soc Lond B Biol Sci. 1987 Nov 23;232(1267):239–248. doi: 10.1098/rspb.1987.0072. [DOI] [PubMed] [Google Scholar]
- Rang H. P. The characteristics of synaptic currents and responses to acetylcholine of rat submandibular ganglion cells. J Physiol. 1981 Feb;311:23–55. doi: 10.1113/jphysiol.1981.sp013571. [DOI] [PMC free article] [PubMed] [Google Scholar]
- Sargent P. B. The diversity of neuronal nicotinic acetylcholine receptors. Annu Rev Neurosci. 1993;16:403–443. doi: 10.1146/annurev.ne.16.030193.002155. [DOI] [PubMed] [Google Scholar]
- Van der Kloot W. The regulation of quantal size. Prog Neurobiol. 1991;36(2):93–130. doi: 10.1016/0301-0082(91)90019-w. [DOI] [PubMed] [Google Scholar]
- Walmsley B. Central synaptic transmission: studies at the connection between primary afferent fibres and dorsal spinocerebellar tract (DSCT) neurones in Clarke's column of the spinal cord. Prog Neurobiol. 1991;36(5):391–423. doi: 10.1016/0301-0082(91)90017-u. [DOI] [PubMed] [Google Scholar]
- Walmsley B., Edwards F. R., Tracey D. J. Nonuniform release probabilities underlie quantal synaptic transmission at a mammalian excitatory central synapse. J Neurophysiol. 1988 Sep;60(3):889–908. doi: 10.1152/jn.1988.60.3.889. [DOI] [PubMed] [Google Scholar]
- Yawo H. Rectification of synaptic and acetylcholine currents in the mouse submandibular ganglion cells. J Physiol. 1989 Oct;417:307–322. doi: 10.1113/jphysiol.1989.sp017803. [DOI] [PMC free article] [PubMed] [Google Scholar]