Abstract
1. We have measured intracellular free calcium ion concentration ([Ca2+]i) with fura-2, and intracellular chloride with chloride-sensitive microelectrodes, in voltage-clamped snail neurones. By making iontophoretic injections of CaCl2 we have investigated calcium muffling, the sum of the processes which minimize the calcium transient, at different values of [Ca2+]i. 2. By injection of calcium into cell-sized droplets of buffer we measured the calcium transport index. It was stable over the range pCa 6-7.4 (0.48 +/- 0.06 measured at pCa 6.70 +/- 0.12, n = 5). 3. Measurement of intracellular chloride activity during a series of fura-2-KCl pressure injections revealed a nearly linear relationship between fura-2 Ca(2+)-insensitive fluorescence and the sum of the increments in intracellular chloride. This allowed us to calculate the intracellular fura-2 concentration ([fura-2]i). 4. The rate of recovery of [Ca2+]i following a depolarization-induced load was increased by low [fura-2]i (10-20 microM) but decreased by higher [fura-2]i (40-80 microM). These effects are consistent with the addition of a mobile buffer to the cytoplasm. 5. Iontophoresis of Ca2+ at various membrane potentials allowed us to calculate the intracellular calcium muffling power (the amount of calcium required to cause a transient tenfold increase in [Ca2+]i per unit volume) and calcium muffling ratio (number of Ca2+ ions injected divided by the maximum increase in [Ca2+]i per unit volume) at different values of [Ca2+]i. 6. Calcium muffling power at resting [Ca2+]i was approximately 40 microM Ca2+ (pCa unit)-1, (about 250 times less than for hydrogen ions). It increased linearly about fivefold with [Ca2+]i over the range 20-120 nM (10 cells, 153 measurements) and therefore exponentially with decreasing pCa. 7. The calcium muffling ratio appeared to be constant (361 +/- 14, n = 10 cells, 130 measurements) over the range 20-120 nM Ca2+. 8. In three experiments we modelled the additional calcium buffering power produced by multiple pressure injections of fura-2 into voltage-clamped snail neurones. Back-extrapolation of the increases in calcium buffering power allowed us to calculate the calcium muffling power of the neurones. 9. Small increases in [fura-2]i (approximately 10 microM) significantly increased intracellular calcium muffling power in individual experiments. However, the variability among neurones in intracellular calcium muffling power was large enough to obscure the additional buffering produced by fura-2 in pooled experiments.
Full text
PDF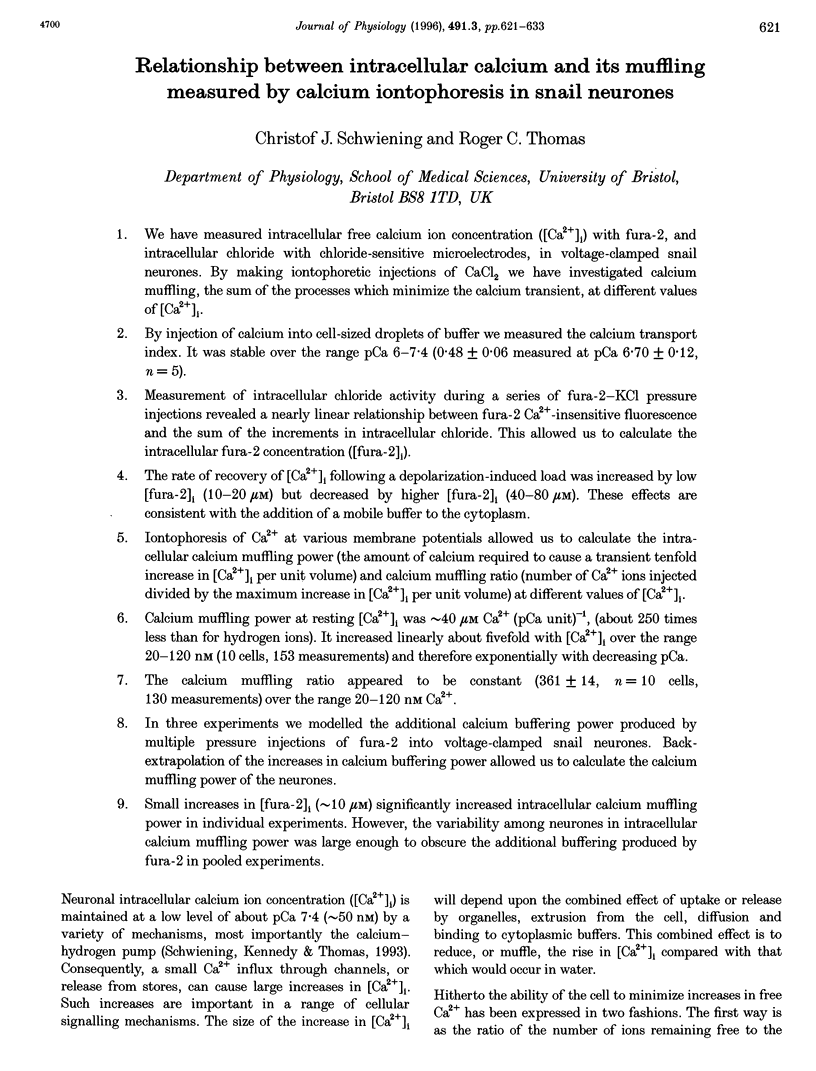
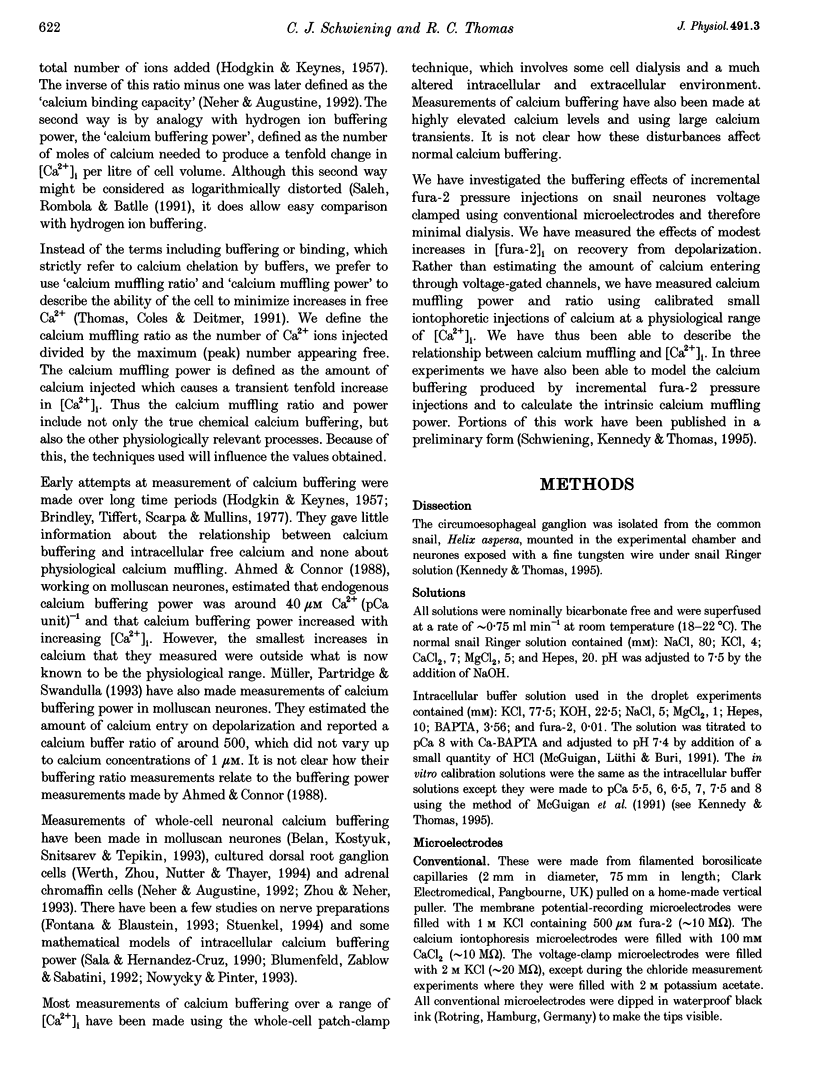
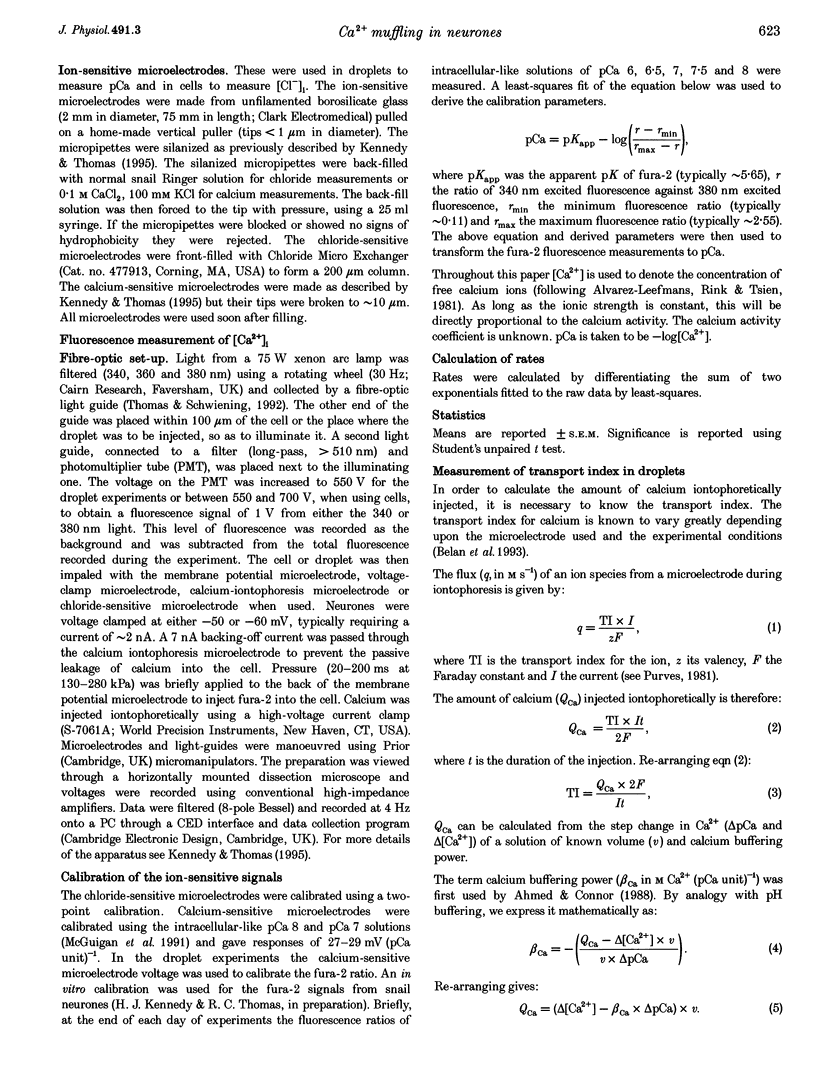
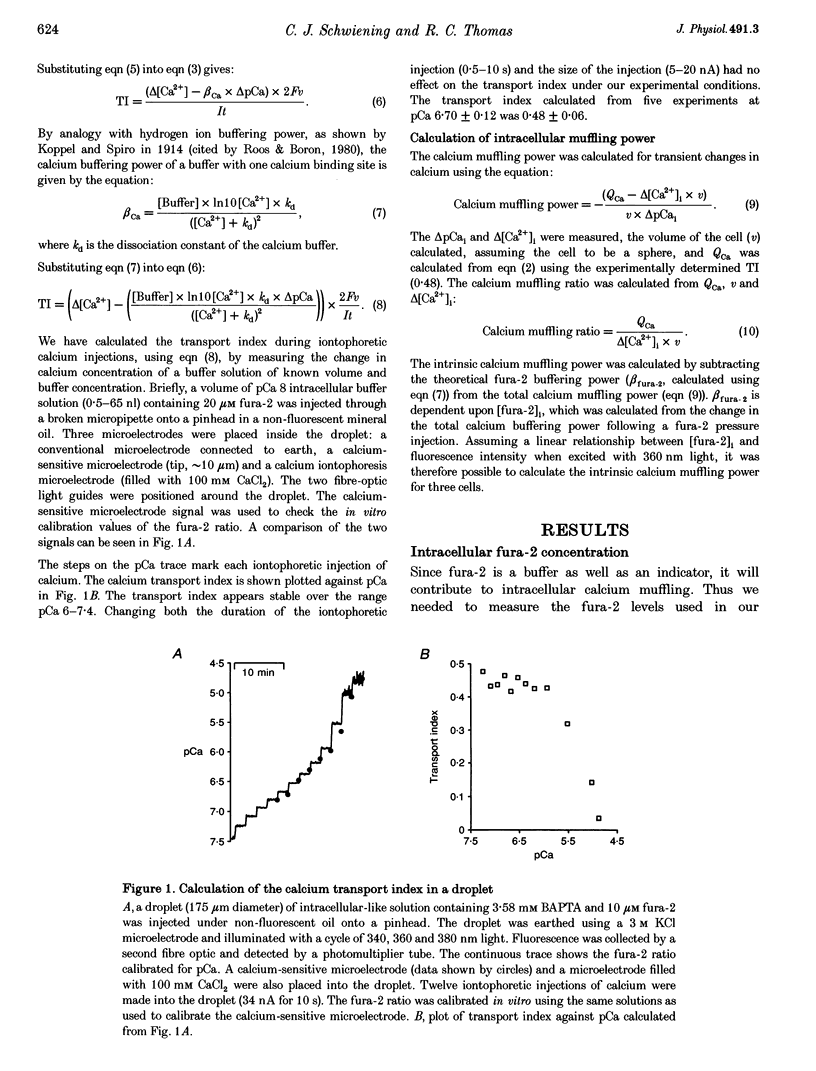
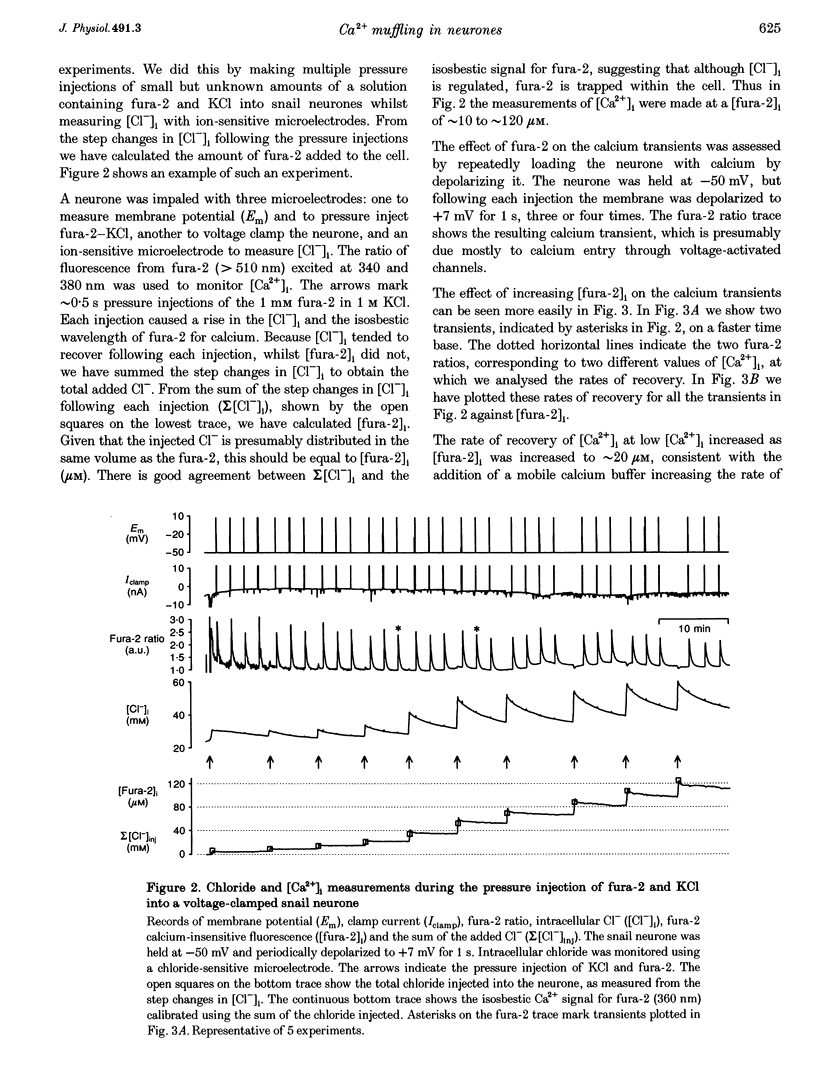
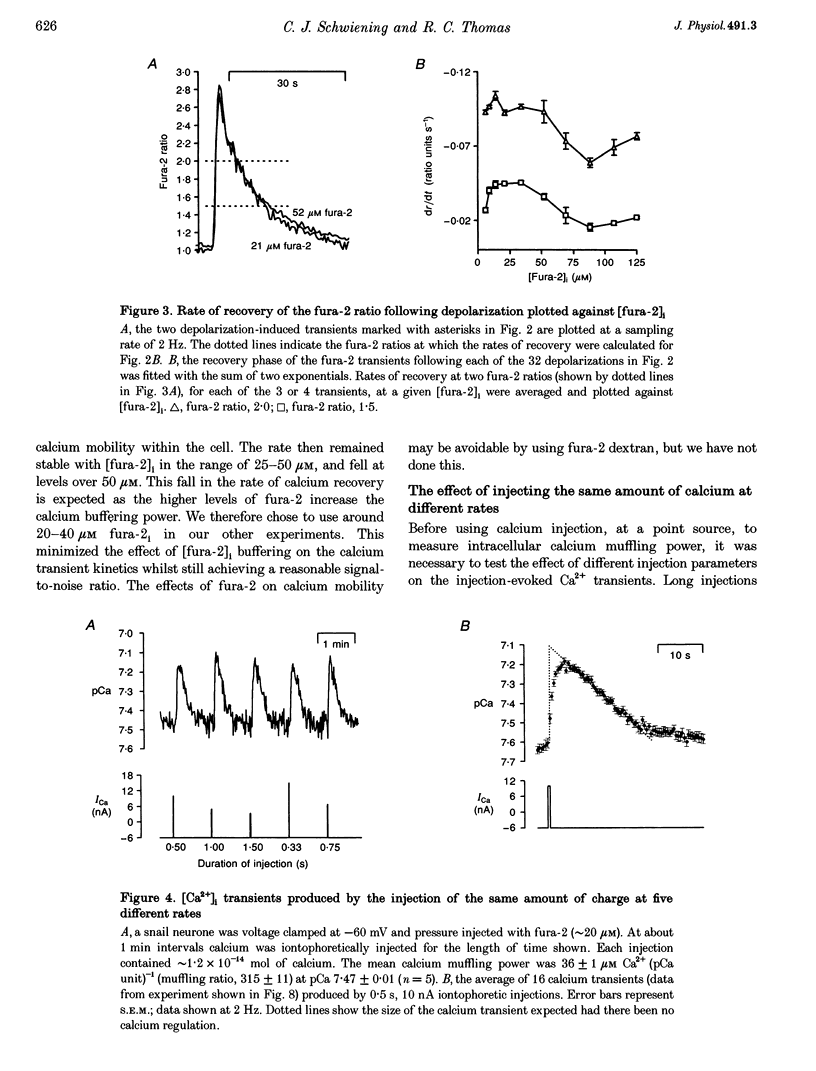
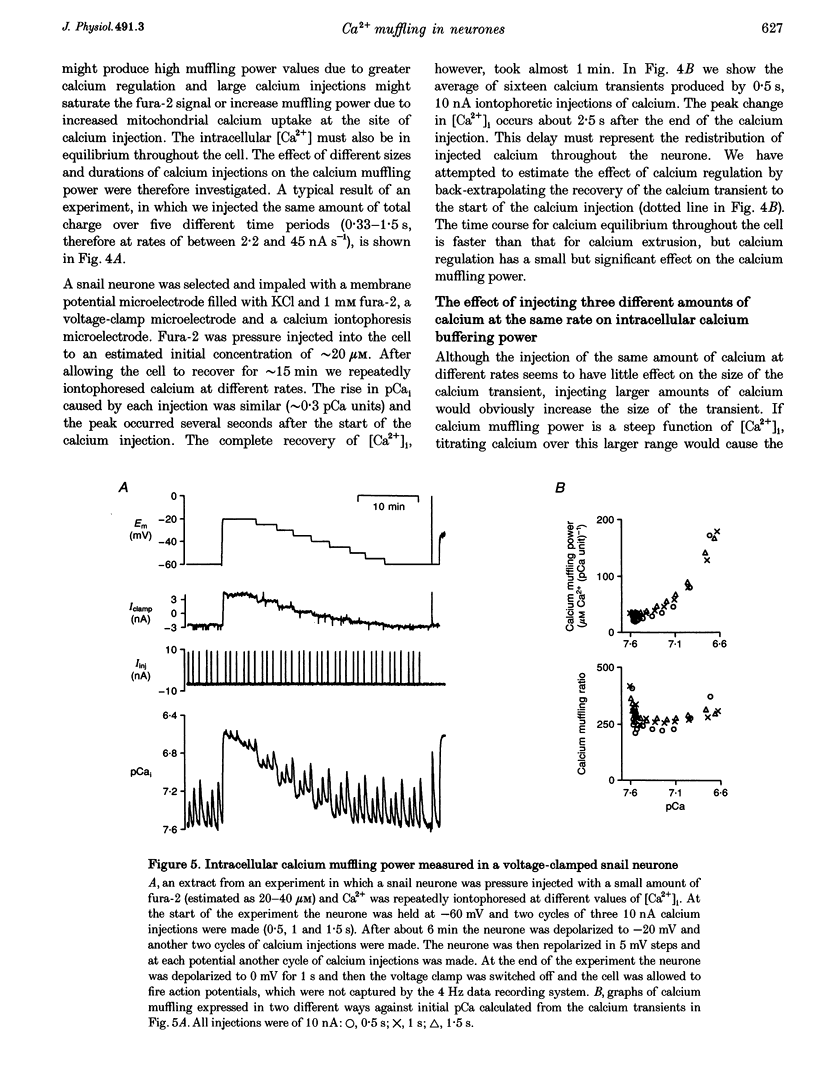
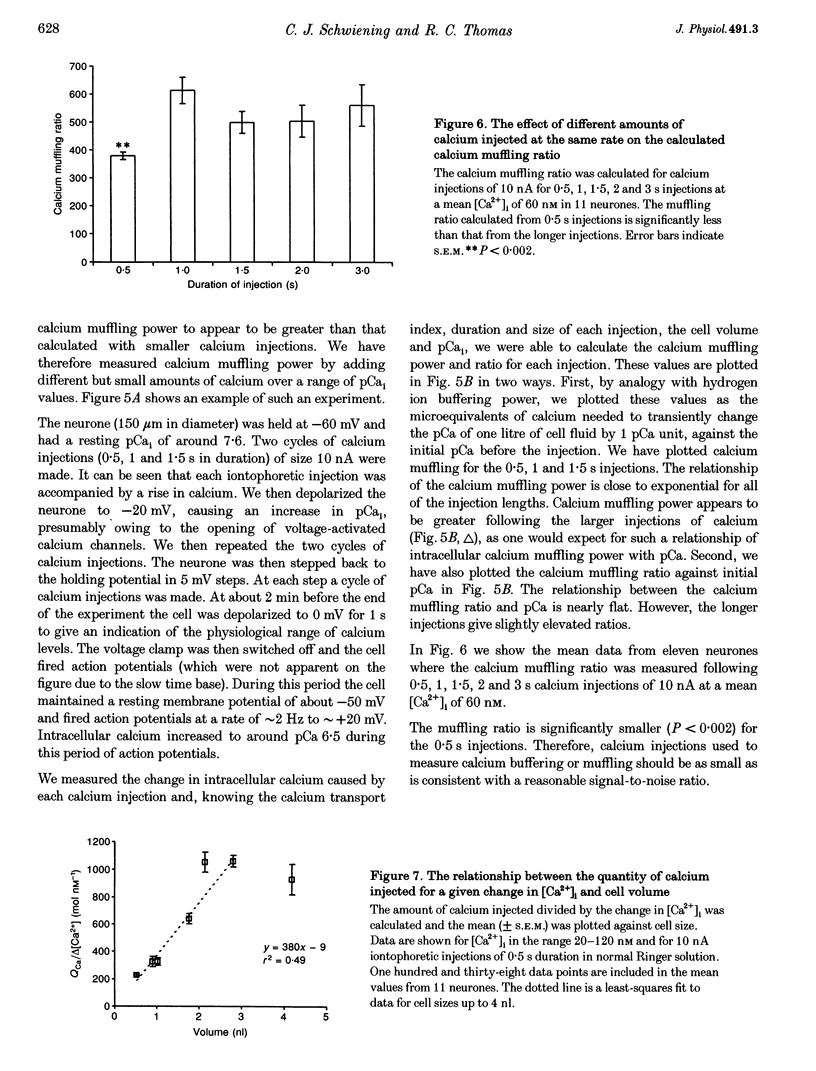
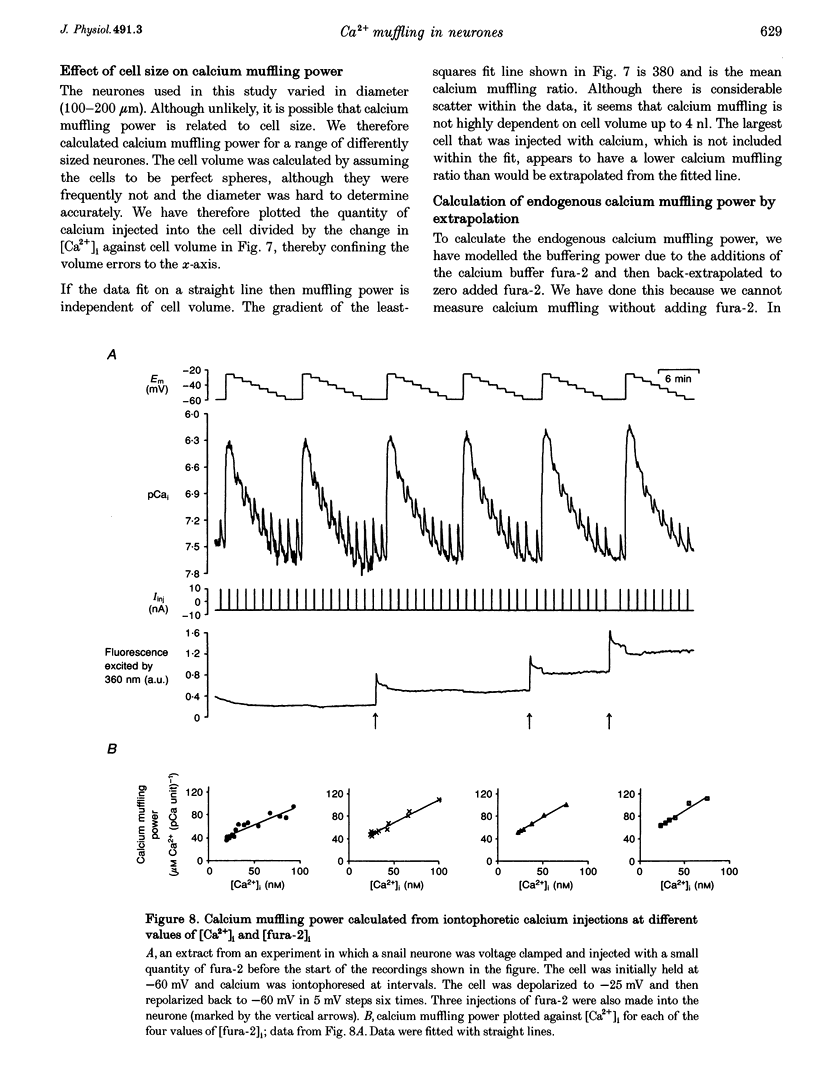
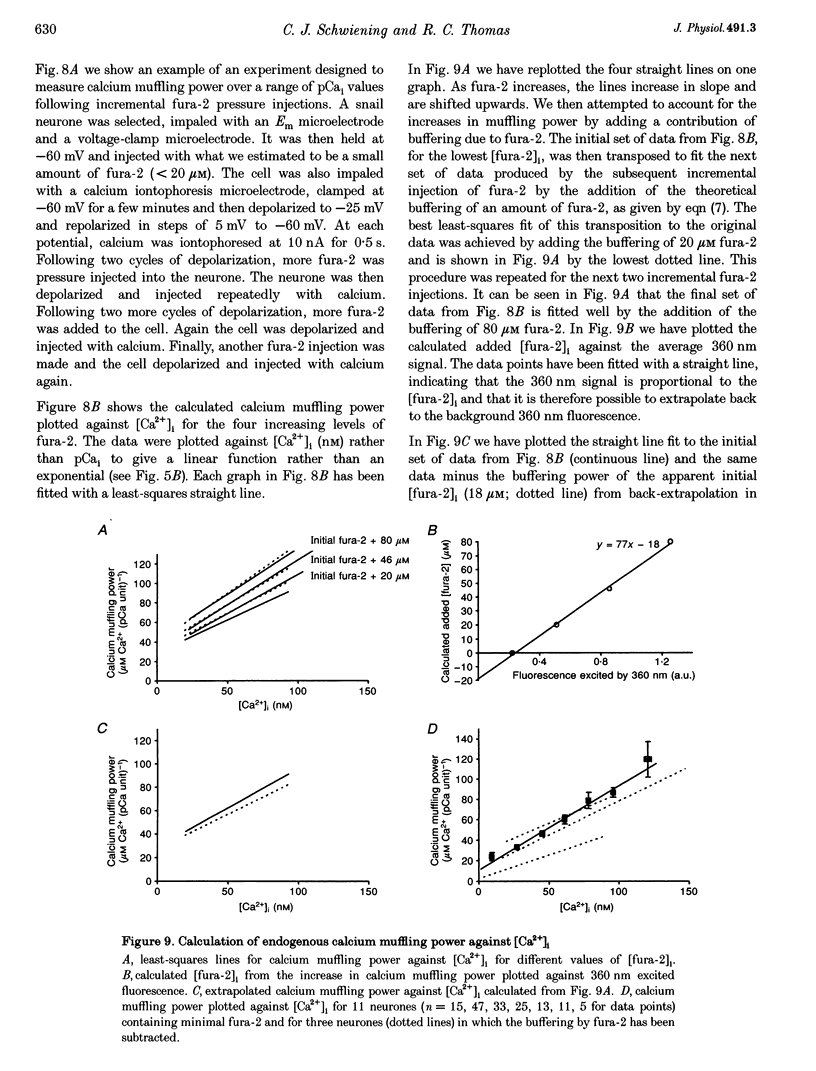
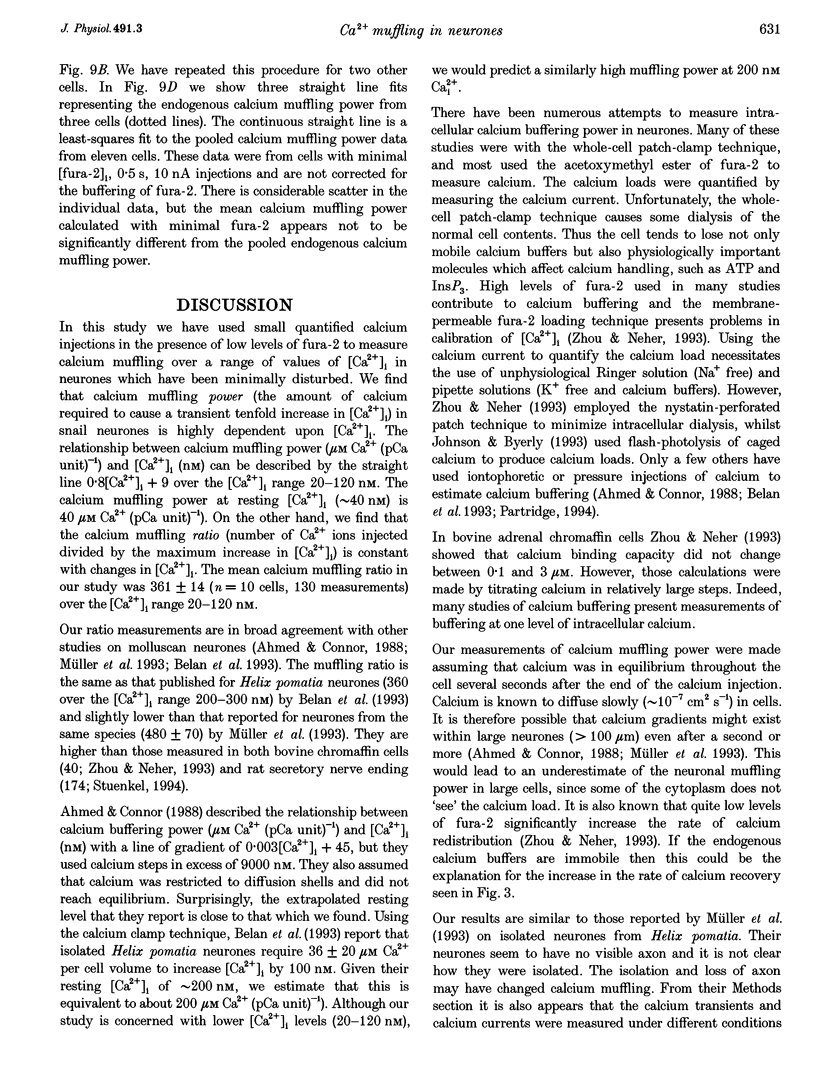
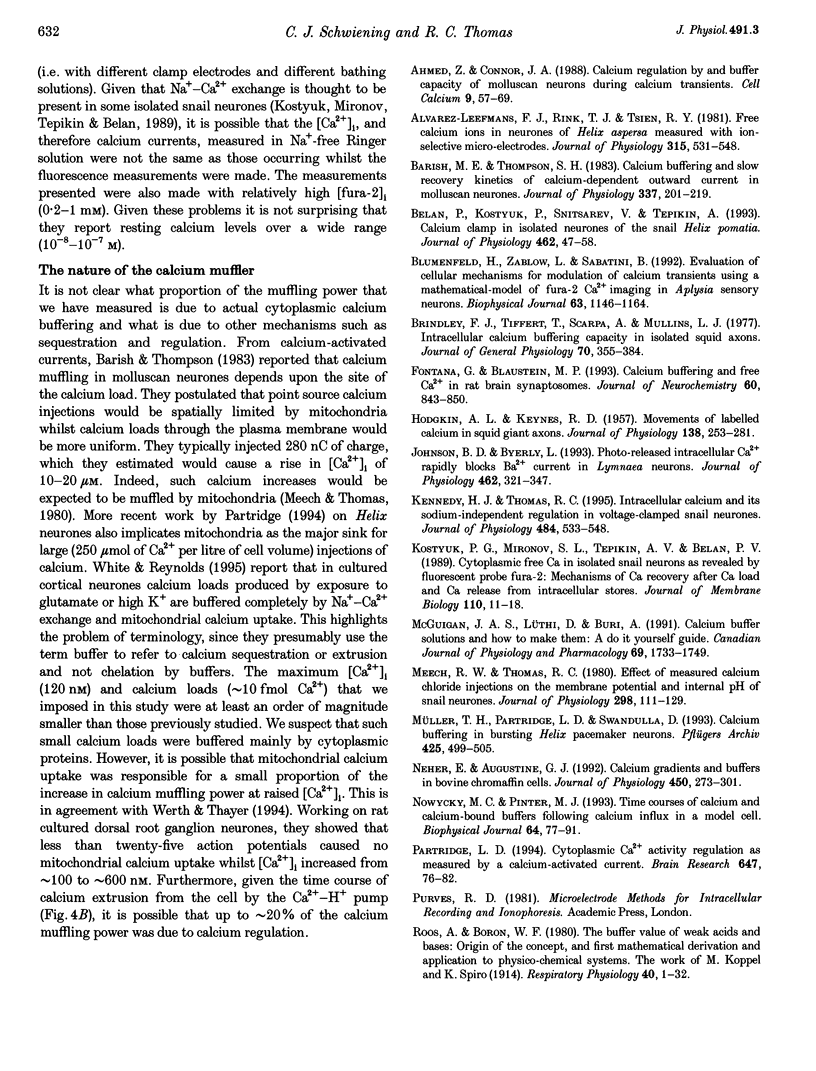
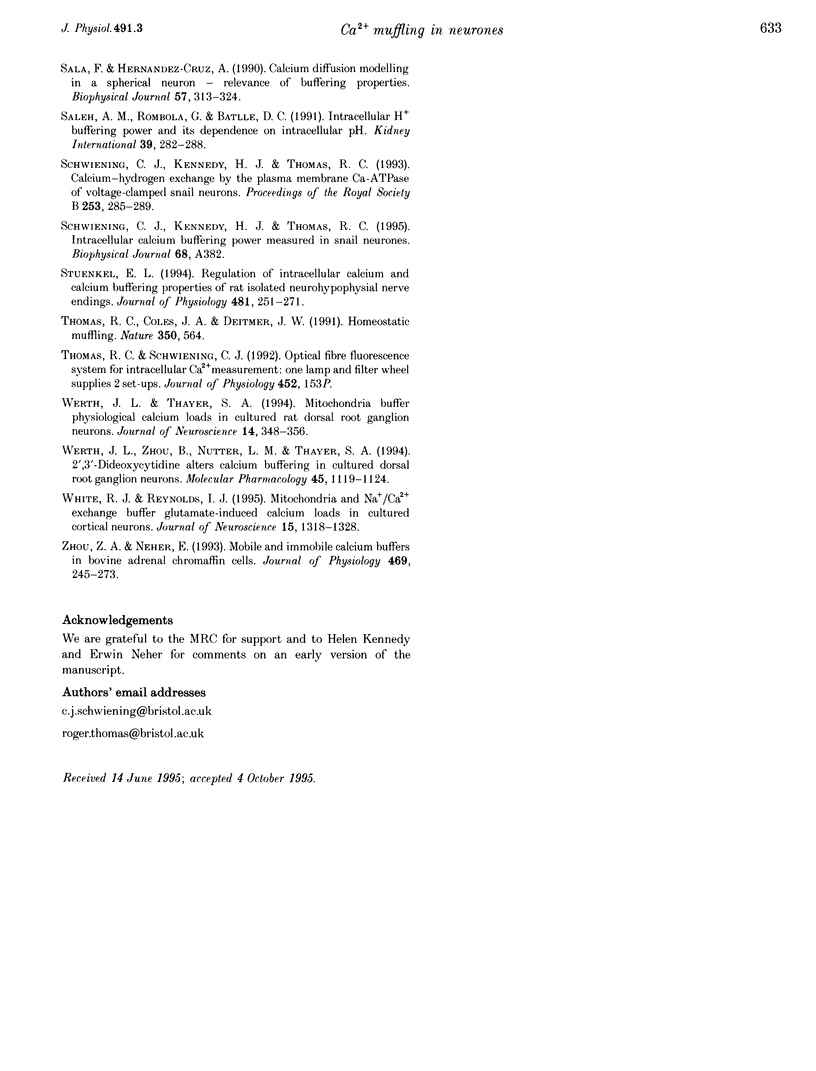
Selected References
These references are in PubMed. This may not be the complete list of references from this article.
- Ahmed Z., Connor J. A. Calcium regulation by and buffer capacity of molluscan neurons during calcium transients. Cell Calcium. 1988 Apr;9(2):57–69. doi: 10.1016/0143-4160(88)90025-5. [DOI] [PubMed] [Google Scholar]
- Alvarez-Leefmans F. J., Rink T. J., Tsien R. Y. Free calcium ions in neurones of Helix aspersa measured with ion-selective micro-electrodes. J Physiol. 1981 Jun;315:531–548. doi: 10.1113/jphysiol.1981.sp013762. [DOI] [PMC free article] [PubMed] [Google Scholar]
- Barish M. E., Thompson S. H. Calcium buffering and slow recovery kinetics of calcium-dependent outward current in molluscan neurones. J Physiol. 1983 Apr;337:201–219. doi: 10.1113/jphysiol.1983.sp014620. [DOI] [PMC free article] [PubMed] [Google Scholar]
- Belan P., Kostyuk P., Snitsarev V., Tepikin A. Calcium clamp in isolated neurones of the snail Helix pomatia. J Physiol. 1993 Mar;462:47–58. doi: 10.1113/jphysiol.1993.sp019542. [DOI] [PMC free article] [PubMed] [Google Scholar]
- Blumenfeld H., Zablow L., Sabatini B. Evaluation of cellular mechanisms for modulation of calcium transients using a mathematical model of fura-2 Ca2+ imaging in Aplysia sensory neurons. Biophys J. 1992 Oct;63(4):1146–1164. doi: 10.1016/S0006-3495(92)81670-3. [DOI] [PMC free article] [PubMed] [Google Scholar]
- Brinley F. J., Jr, Tiffert T., Scarpa A., Mullins L. J. Intracellular calcium buffering capacity in isolated squid axons. J Gen Physiol. 1977 Sep;70(3):355–384. doi: 10.1085/jgp.70.3.355. [DOI] [PMC free article] [PubMed] [Google Scholar]
- Fontana G., Blaustein M. P. Calcium buffering and free Ca2+ in rat brain synaptosomes. J Neurochem. 1993 Mar;60(3):843–850. doi: 10.1111/j.1471-4159.1993.tb03228.x. [DOI] [PubMed] [Google Scholar]
- HODGKIN A. L., KEYNES R. D. Movements of labelled calcium in squid giant axons. J Physiol. 1957 Sep 30;138(2):253–281. doi: 10.1113/jphysiol.1957.sp005850. [DOI] [PMC free article] [PubMed] [Google Scholar]
- Johnson B. D., Byerly L. Photo-released intracellular Ca2+ rapidly blocks Ba2+ current in Lymnaea neurons. J Physiol. 1993 Mar;462:321–347. doi: 10.1113/jphysiol.1993.sp019558. [DOI] [PMC free article] [PubMed] [Google Scholar]
- Kennedy H. J., Thomas R. C. Intracellular calcium and its sodium-independent regulation in voltage-clamped snail neurones. J Physiol. 1995 May 1;484(Pt 3):533–548. doi: 10.1113/jphysiol.1995.sp020684. [DOI] [PMC free article] [PubMed] [Google Scholar]
- McGuigan J. A., Lüthi D., Buri A. Calcium buffer solutions and how to make them: a do it yourself guide. Can J Physiol Pharmacol. 1991 Nov;69(11):1733–1749. doi: 10.1139/y91-257. [DOI] [PubMed] [Google Scholar]
- Meech R. W., Thomas R. C. Effect of measured calcium chloride injections on the membrane potential and internal pH of snail neurones. J Physiol. 1980 Jan;298:111–129. doi: 10.1113/jphysiol.1980.sp013070. [DOI] [PMC free article] [PubMed] [Google Scholar]
- Müller T. H., Partridge L. D., Swandulla D. Calcium buffering in bursting Helix pacemaker neurons. Pflugers Arch. 1993 Dec;425(5-6):499–505. doi: 10.1007/BF00374877. [DOI] [PubMed] [Google Scholar]
- Neher E., Augustine G. J. Calcium gradients and buffers in bovine chromaffin cells. J Physiol. 1992 May;450:273–301. doi: 10.1113/jphysiol.1992.sp019127. [DOI] [PMC free article] [PubMed] [Google Scholar]
- Nowycky M. C., Pinter M. J. Time courses of calcium and calcium-bound buffers following calcium influx in a model cell. Biophys J. 1993 Jan;64(1):77–91. doi: 10.1016/S0006-3495(93)81342-0. [DOI] [PMC free article] [PubMed] [Google Scholar]
- Partridge L. D. Cytoplasmic Ca2+ activity regulation as measured by a calcium-activated current. Brain Res. 1994 May 30;647(1):76–82. doi: 10.1016/0006-8993(94)91400-1. [DOI] [PubMed] [Google Scholar]
- Roos A., Boron W. F. The buffer value of weak acids and bases: origin of the concept, and first mathematical derivation and application to physico-chemical systems. The work of M. Koppel and K. Spiro (1914). Respir Physiol. 1980 Apr;40(1):1–32. doi: 10.1016/0034-5687(80)90002-x. [DOI] [PubMed] [Google Scholar]
- Sala F., Hernández-Cruz A. Calcium diffusion modeling in a spherical neuron. Relevance of buffering properties. Biophys J. 1990 Feb;57(2):313–324. doi: 10.1016/S0006-3495(90)82533-9. [DOI] [PMC free article] [PubMed] [Google Scholar]
- Saleh A. M., Rombola G., Batlle D. C. Intracellular H+ buffering power and its dependency on intracellular pH. Kidney Int. 1991 Feb;39(2):282–288. doi: 10.1038/ki.1991.34. [DOI] [PubMed] [Google Scholar]
- Schwiening C. J., Kennedy H. J., Thomas R. C. Calcium-hydrogen exchange by the plasma membrane Ca-ATPase of voltage-clamped snail neurons. Proc Biol Sci. 1993 Sep 22;253(1338):285–289. doi: 10.1098/rspb.1993.0115. [DOI] [PubMed] [Google Scholar]
- Stuenkel E. L. Regulation of intracellular calcium and calcium buffering properties of rat isolated neurohypophysial nerve endings. J Physiol. 1994 Dec 1;481(Pt 2):251–271. doi: 10.1113/jphysiol.1994.sp020436. [DOI] [PMC free article] [PubMed] [Google Scholar]
- Thomas R. C., Coles J. A., Deitmer J. W. Homeostatic muffling. Nature. 1991 Apr 18;350(6319):564–564. doi: 10.1038/350564b0. [DOI] [PubMed] [Google Scholar]
- Werth J. L., Thayer S. A. Mitochondria buffer physiological calcium loads in cultured rat dorsal root ganglion neurons. J Neurosci. 1994 Jan;14(1):348–356. doi: 10.1523/JNEUROSCI.14-01-00348.1994. [DOI] [PMC free article] [PubMed] [Google Scholar]
- Werth J. L., Zhou B., Nutter L. M., Thayer S. A. 2',3'-Dideoxycytidine alters calcium buffering in cultured dorsal root ganglion neurons. Mol Pharmacol. 1994 Jun;45(6):1119–1124. [PubMed] [Google Scholar]
- White R. J., Reynolds I. J. Mitochondria and Na+/Ca2+ exchange buffer glutamate-induced calcium loads in cultured cortical neurons. J Neurosci. 1995 Feb;15(2):1318–1328. doi: 10.1523/JNEUROSCI.15-02-01318.1995. [DOI] [PMC free article] [PubMed] [Google Scholar]
- Zhou Z., Neher E. Mobile and immobile calcium buffers in bovine adrenal chromaffin cells. J Physiol. 1993 Sep;469:245–273. doi: 10.1113/jphysiol.1993.sp019813. [DOI] [PMC free article] [PubMed] [Google Scholar]