Abstract
1. Spontaneous rhythmic activity in hypoglossal (XII) rootlets is generated at all postnatal stages from postnatal day (P) 0 to P22 in the transverse brainstem slice of mice containing the pre-Bötzinger complex (PBC). The PBC is known to be a region essential for respiratory rhythm generation. It contains neurones generating periodic bursts that occur in synchrony with rhythmic XII activity. This synchrony indicates that the rhythmic PBC activity generated by the transverse slice is the central respiratory rhythm. 2. The strength of coupling between XII bursts and PBC bursts decreased during early postnatal development. In younger mice (P0-4) each burst in XII rootlets corresponded to one burst in the PBC. In older mice (P5-18) one burst in XII rootlets occurred only every third to fourth burst in PBC neurones. 3. Cycle length and burst duration of rhythmic XII activity did not change significantly during the first three postnatal weeks. However, the pattern of XII bursts changed from decrementing (P0-7) to bell shaped (P8-18) while the rate of rise of XII bursts decreased significantly. 4. The rate of rise of rhythmic depolarizations in neurones of the PBC discharging in phase with XII bursts ('inspiratory neurones') decreased with postnatal development. During interburst intervals, membrane potentials of neurones of older mice (P6-18) were characterized by waves of synaptic input that were not observed in neonatal animals (P0-5). 5. Blockade of glycine receptors by strychnine increased the frequency of rhythmic XII activity in neonatal and older mice (P0-22). Although in expiratory PBC neurones glycinergic transmission was blocked at 10 microM strychnine, in inspiratory PBC neurones and XII rootlets even higher concentrations of up to 50 microM strychnine failed to abolish rhythmic activity.
Full text
PDF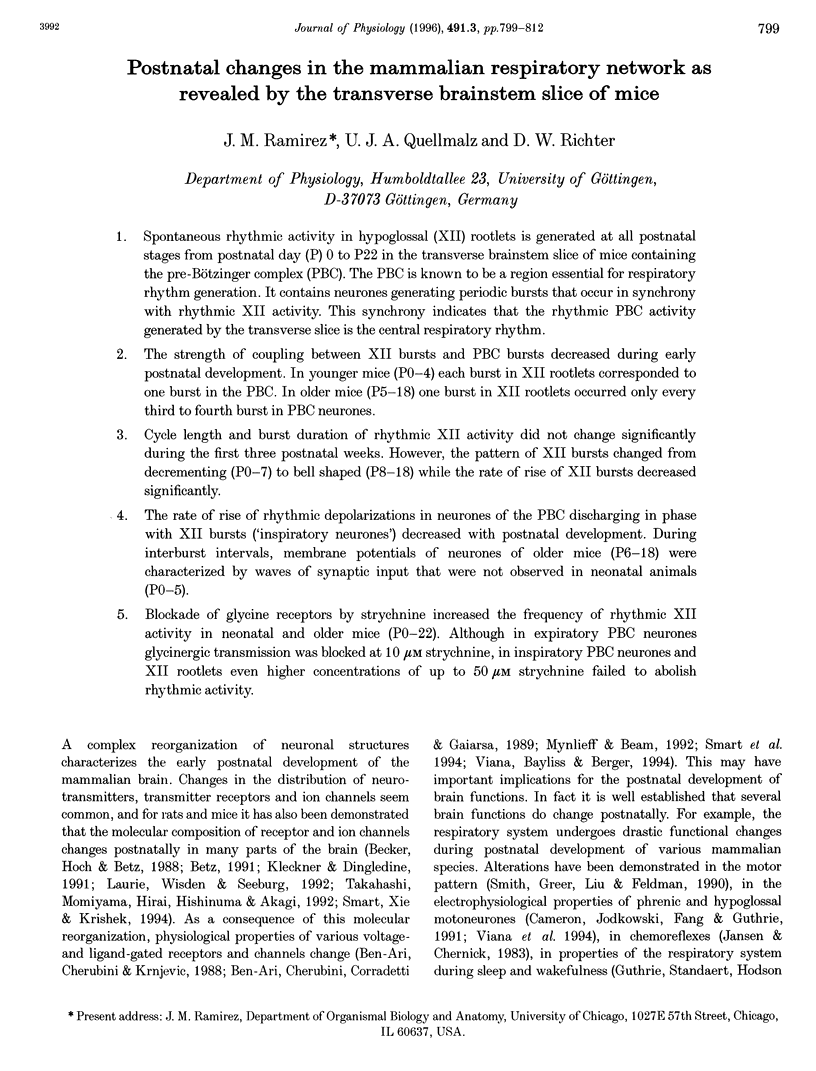
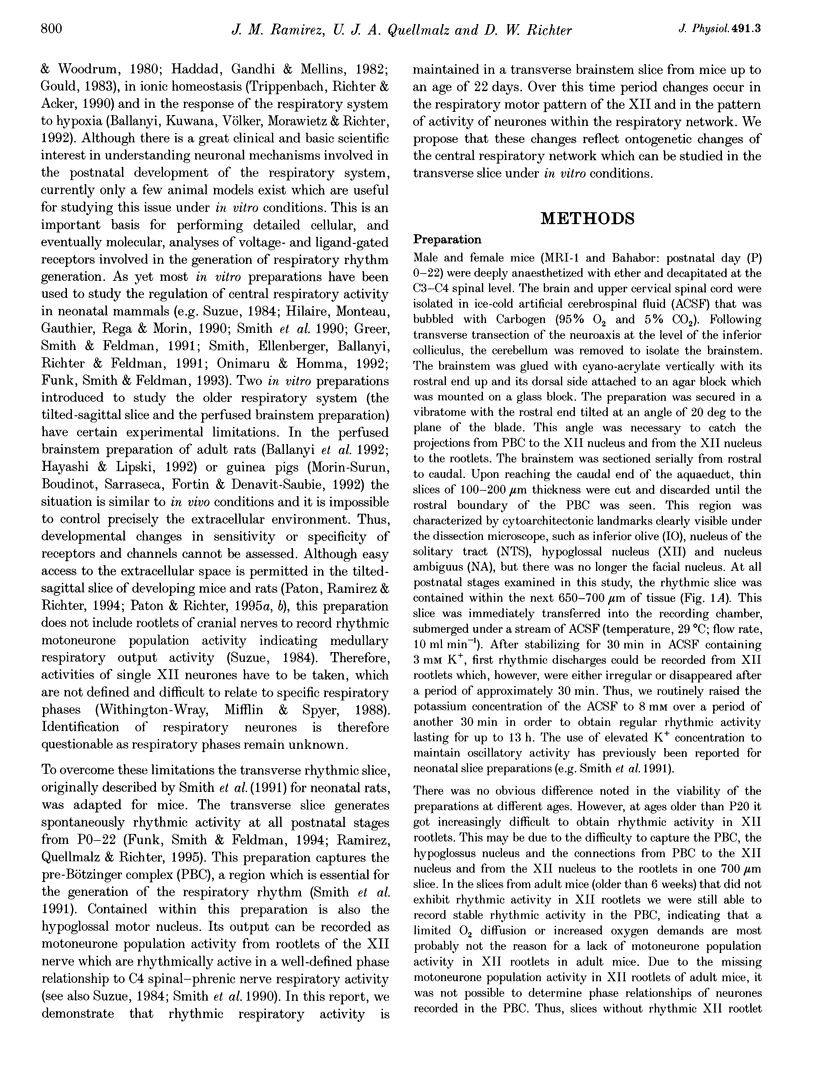
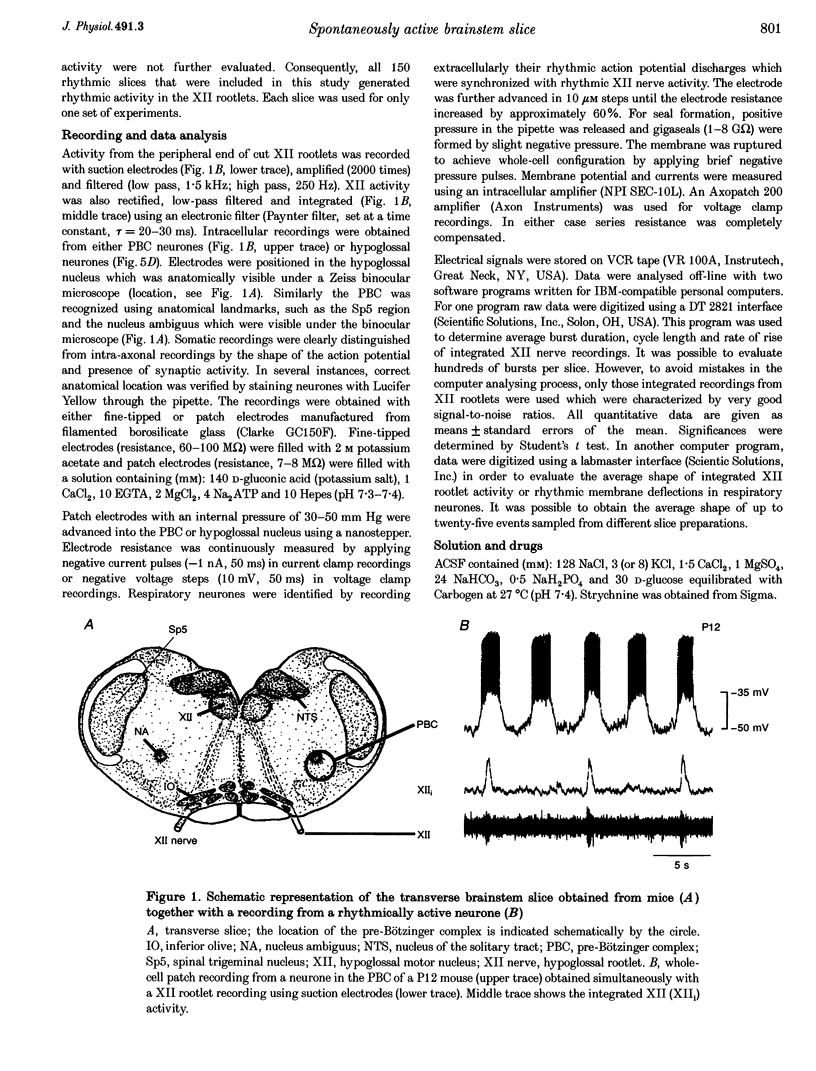
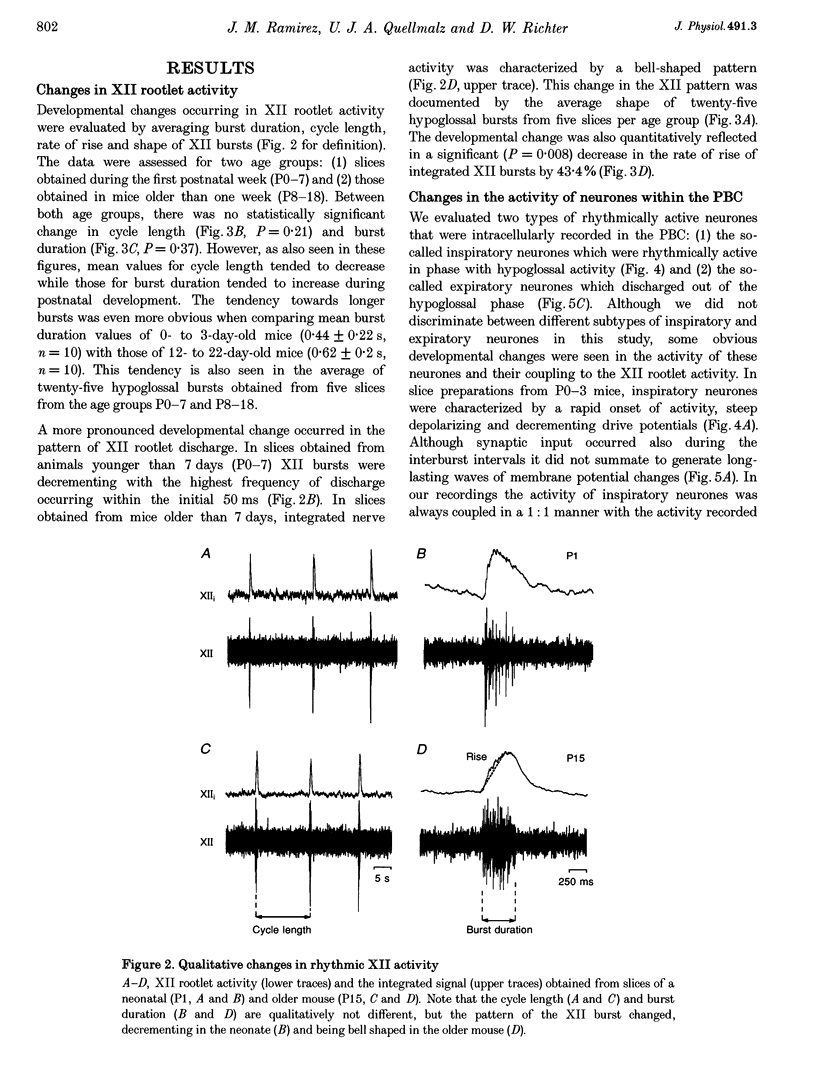
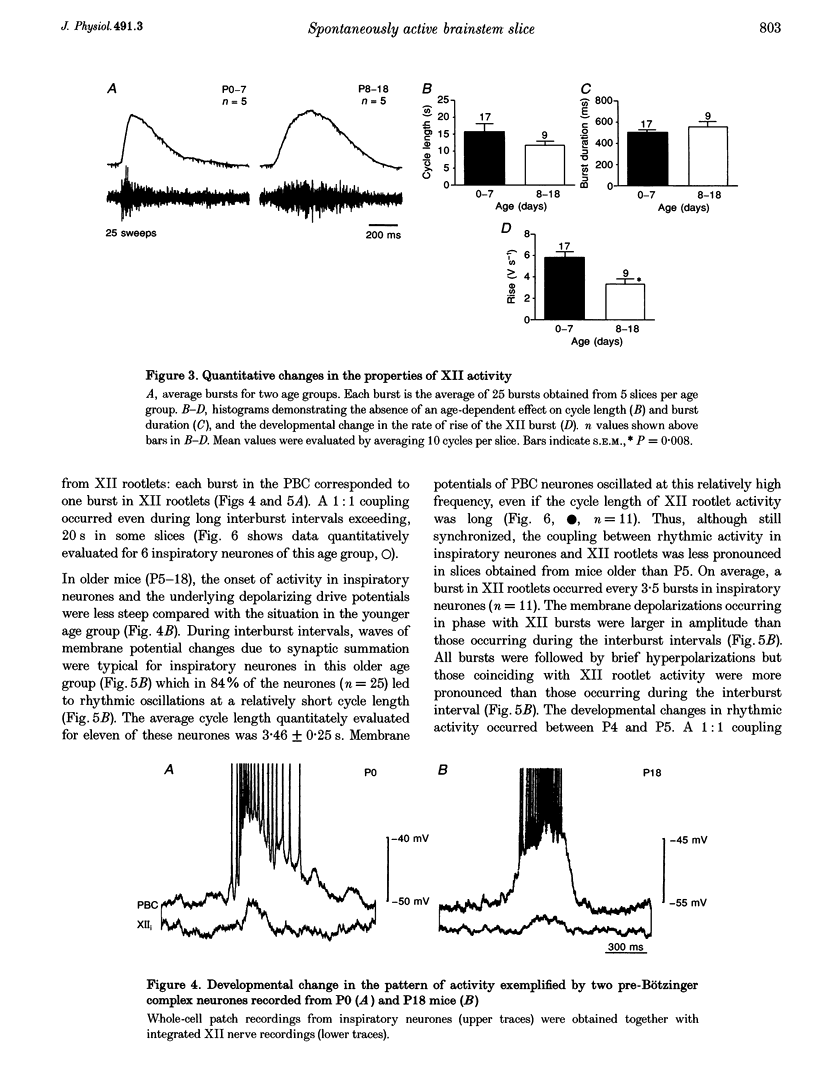
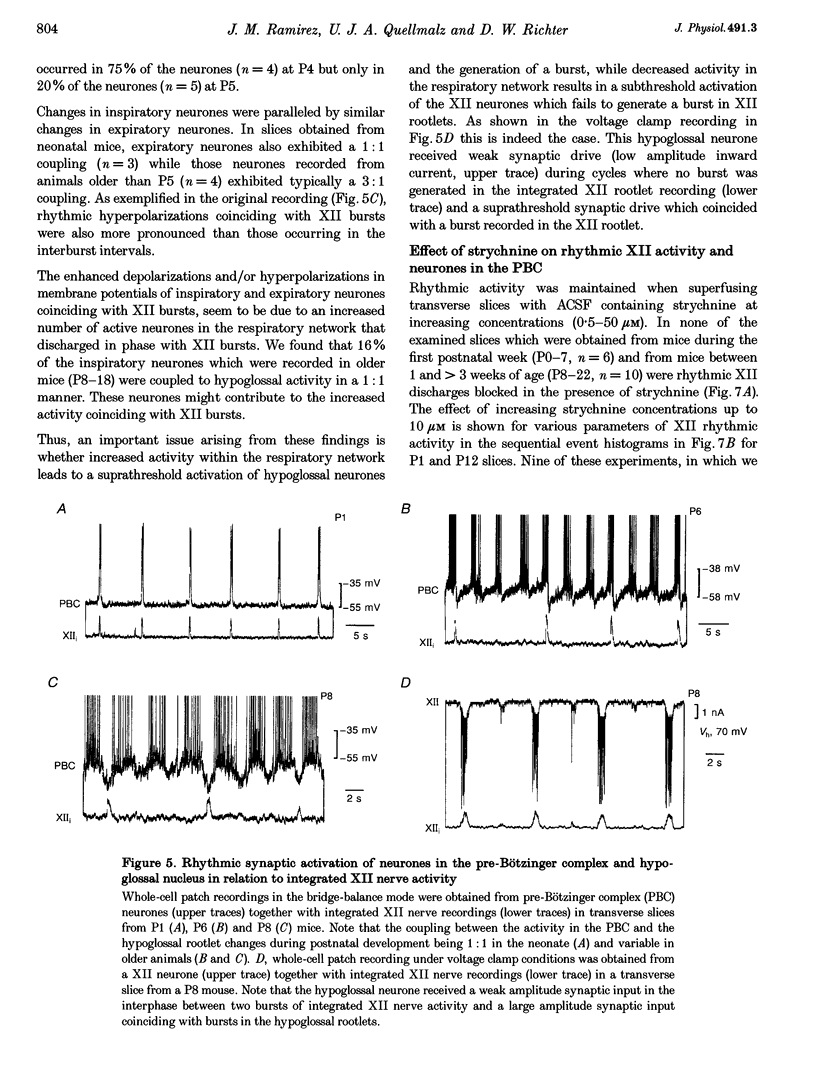
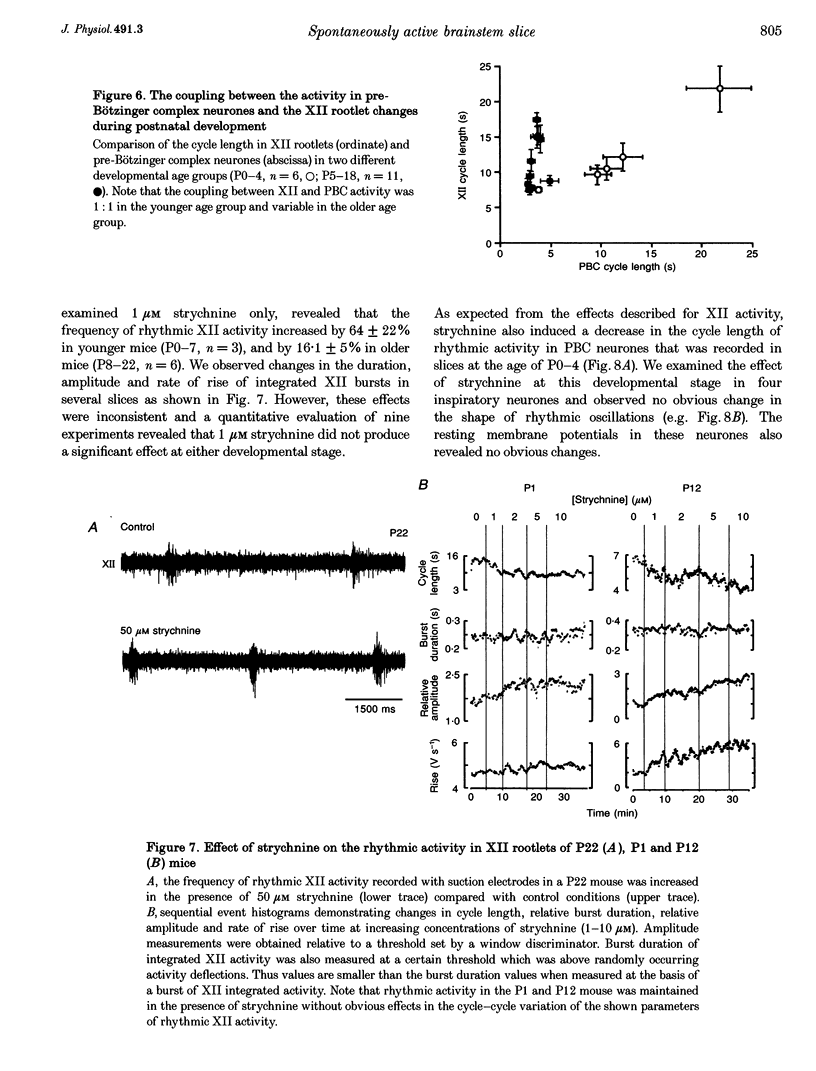
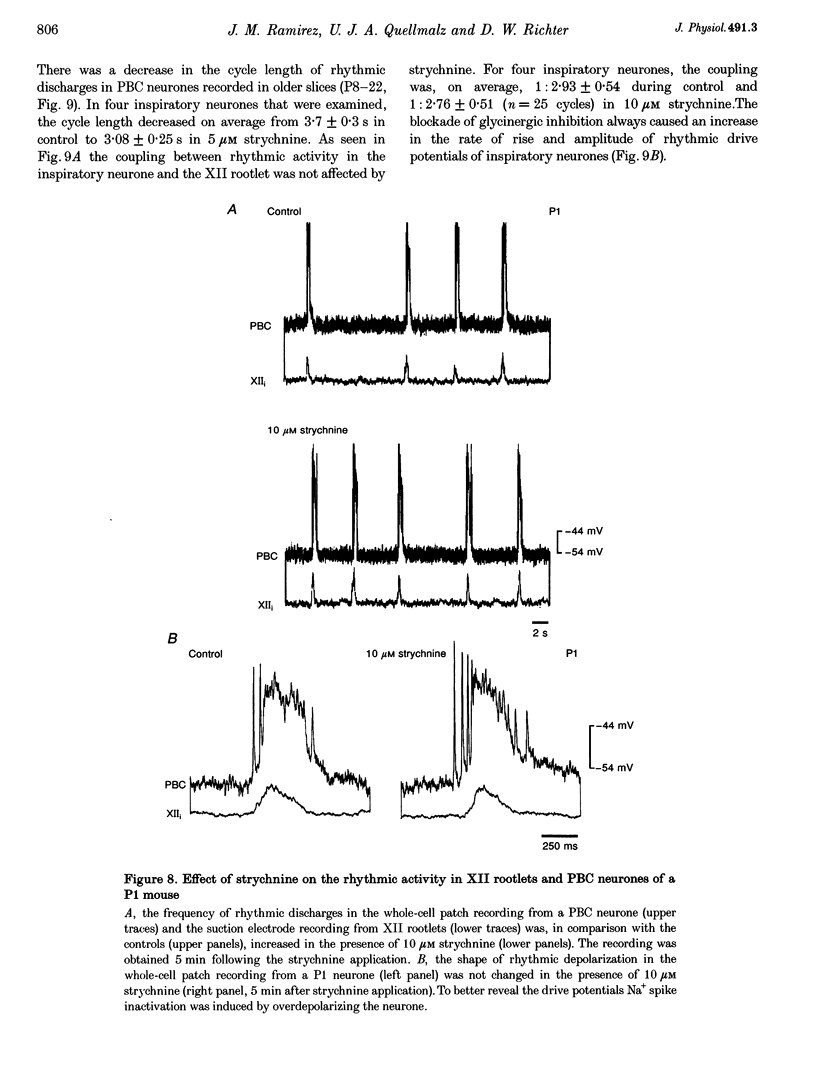
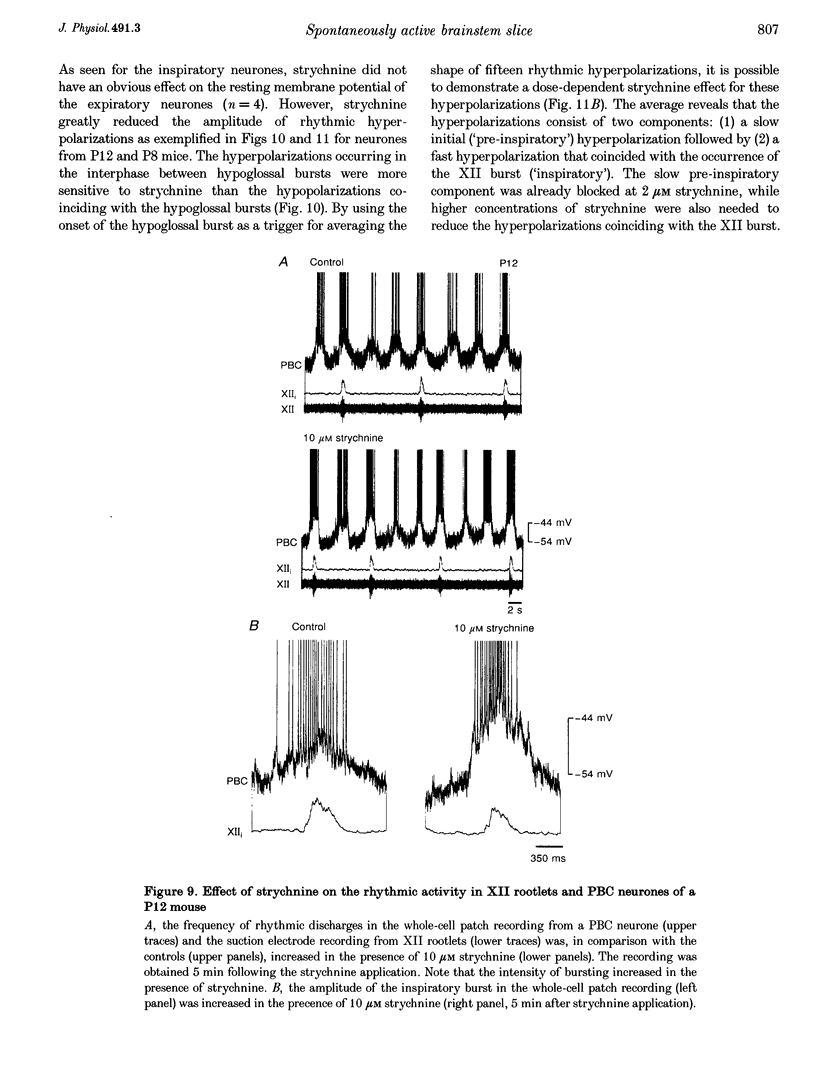
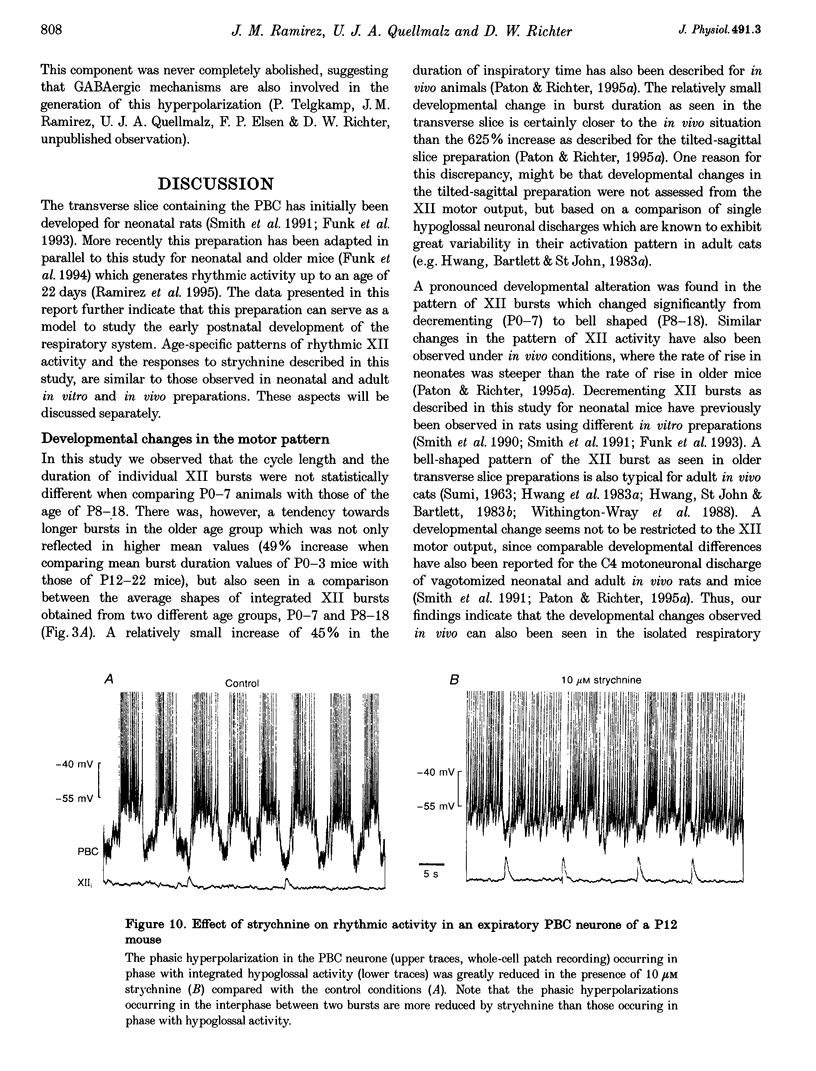
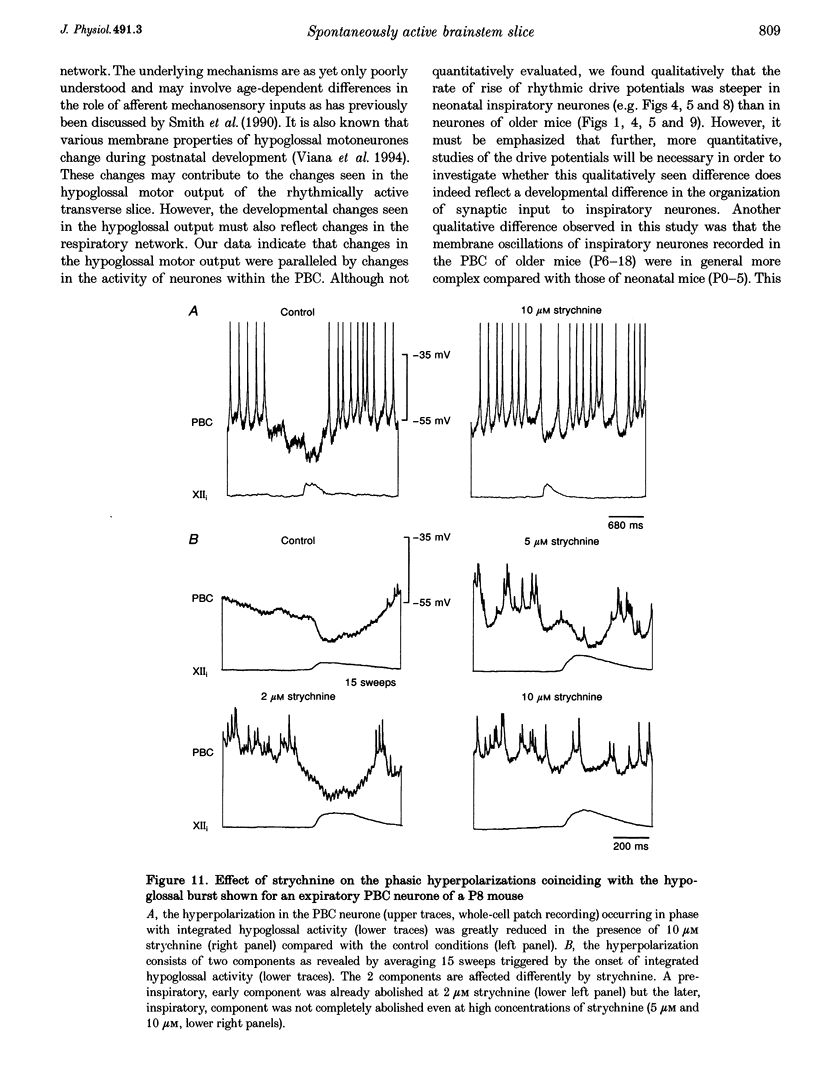
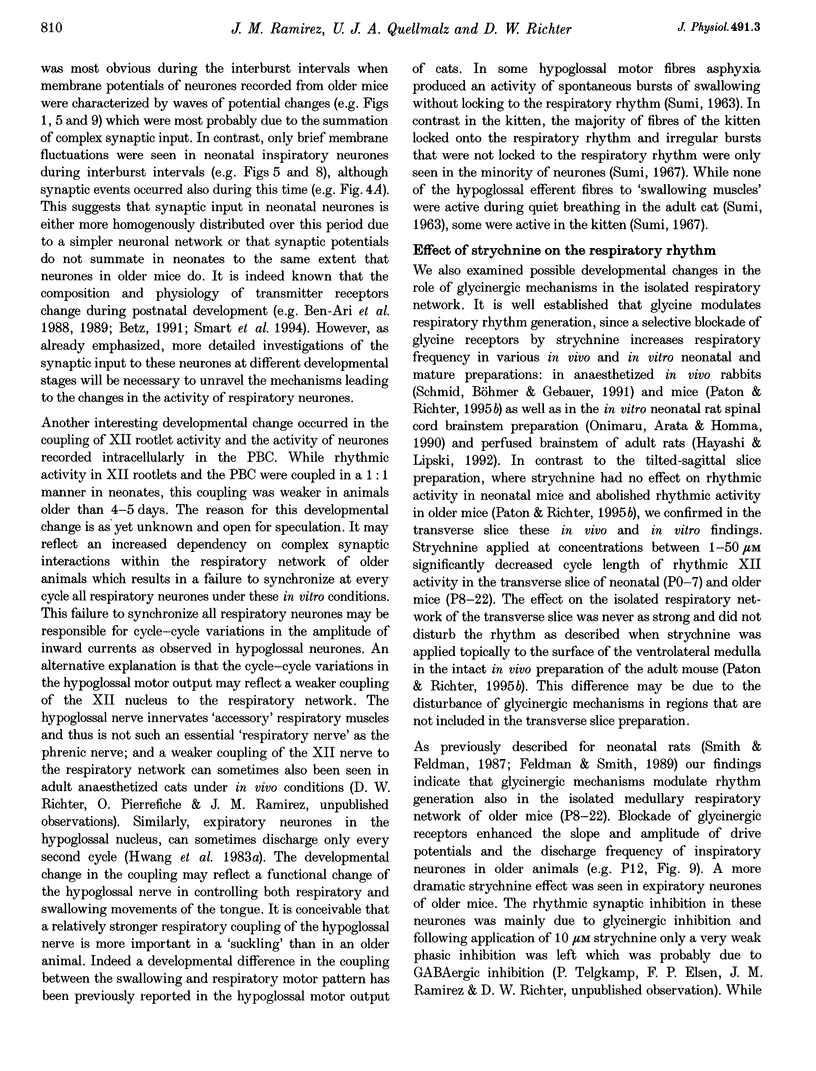
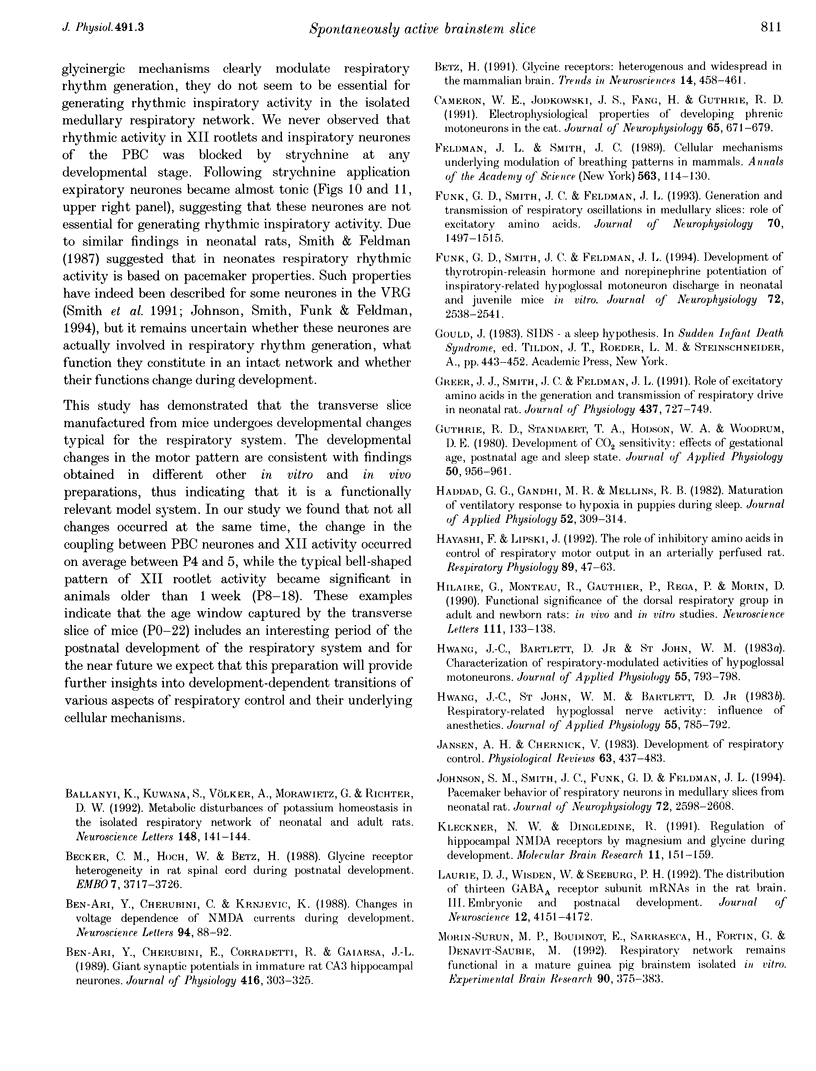
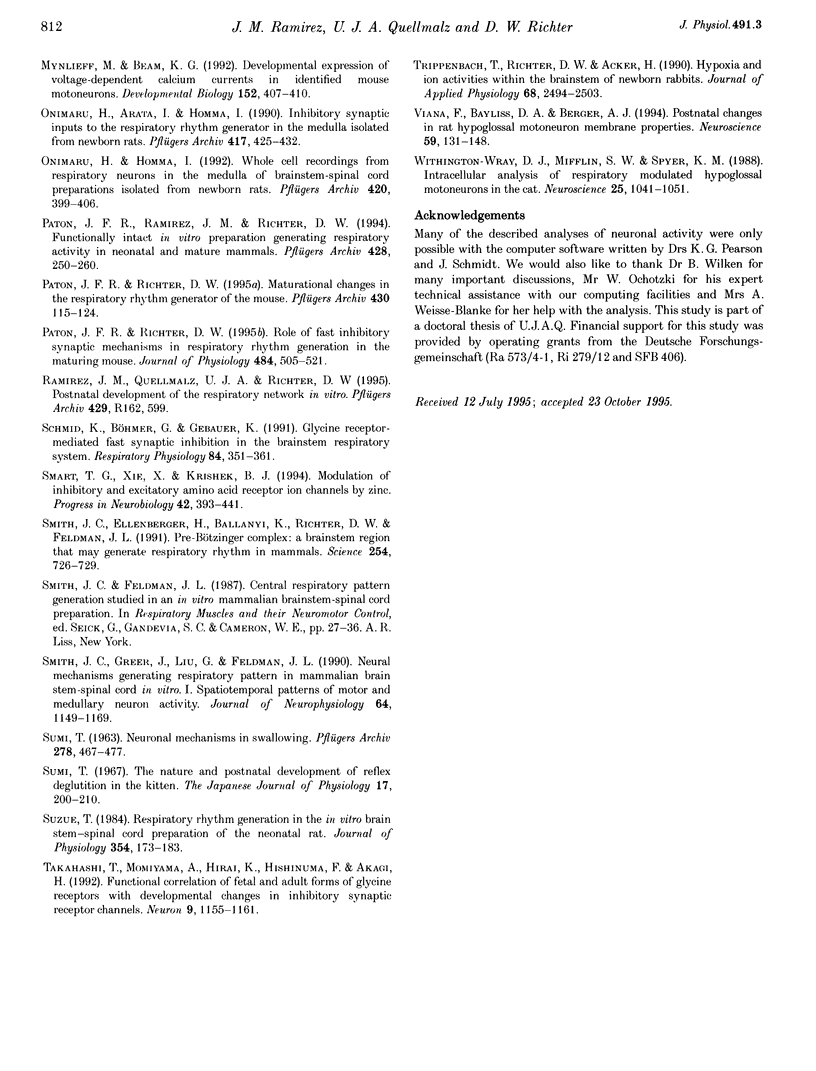
Selected References
These references are in PubMed. This may not be the complete list of references from this article.
- Becker C. M., Hoch W., Betz H. Glycine receptor heterogeneity in rat spinal cord during postnatal development. EMBO J. 1988 Dec 1;7(12):3717–3726. doi: 10.1002/j.1460-2075.1988.tb03255.x. [DOI] [PMC free article] [PubMed] [Google Scholar]
- Ben-Ari Y., Cherubini E., Corradetti R., Gaiarsa J. L. Giant synaptic potentials in immature rat CA3 hippocampal neurones. J Physiol. 1989 Sep;416:303–325. doi: 10.1113/jphysiol.1989.sp017762. [DOI] [PMC free article] [PubMed] [Google Scholar]
- Ben-Ari Y., Cherubini E., Krnjevic K. Changes in voltage dependence of NMDA currents during development. Neurosci Lett. 1988 Nov 22;94(1-2):88–92. doi: 10.1016/0304-3940(88)90275-3. [DOI] [PubMed] [Google Scholar]
- Betz H. Glycine receptors: heterogeneous and widespread in the mammalian brain. Trends Neurosci. 1991 Oct;14(10):458–461. doi: 10.1016/0166-2236(91)90045-v. [DOI] [PubMed] [Google Scholar]
- Feldman J. L., Smith J. C. Cellular mechanisms underlying modulation of breathing pattern in mammals. Ann N Y Acad Sci. 1989;563:114–130. doi: 10.1111/j.1749-6632.1989.tb42194.x. [DOI] [PubMed] [Google Scholar]
- Funk G. D., Smith J. C., Feldman J. L. Development of thyrotropin-releasing hormone and norepinephrine potentiation of inspiratory-related hypoglossal motoneuron discharge in neonatal and juvenile mice in vitro. J Neurophysiol. 1994 Nov;72(5):2538–2541. doi: 10.1152/jn.1994.72.5.2538. [DOI] [PubMed] [Google Scholar]
- Funk G. D., Smith J. C., Feldman J. L. Generation and transmission of respiratory oscillations in medullary slices: role of excitatory amino acids. J Neurophysiol. 1993 Oct;70(4):1497–1515. doi: 10.1152/jn.1993.70.4.1497. [DOI] [PubMed] [Google Scholar]
- Greer J. J., Smith J. C., Feldman J. L. Role of excitatory amino acids in the generation and transmission of respiratory drive in neonatal rat. J Physiol. 1991 Jun;437:727–749. doi: 10.1113/jphysiol.1991.sp018622. [DOI] [PMC free article] [PubMed] [Google Scholar]
- Guthrie R. D., Standaert T. A., Hodson W. A., Woodrum D. E. Development of CO2 sensitivity: effects of gestational age, postnatal age, and sleep state. J Appl Physiol Respir Environ Exerc Physiol. 1981 May;50(5):956–961. doi: 10.1152/jappl.1981.50.5.956. [DOI] [PubMed] [Google Scholar]
- Hayashi F., Lipski J. The role of inhibitory amino acids in control of respiratory motor output in an arterially perfused rat. Respir Physiol. 1992 Jul;89(1):47–63. doi: 10.1016/0034-5687(92)90070-d. [DOI] [PubMed] [Google Scholar]
- Hilaire G., Monteau R., Gauthier P., Rega P., Morin D. Functional significance of the dorsal respiratory group in adult and newborn rats: in vivo and in vitro studies. Neurosci Lett. 1990 Mar 26;111(1-2):133–138. doi: 10.1016/0304-3940(90)90357-f. [DOI] [PubMed] [Google Scholar]
- Hwang J. C., Bartlett D., Jr, St John W. M. Characterization of respiratory-modulated activities of hypoglossal motoneurons. J Appl Physiol Respir Environ Exerc Physiol. 1983 Sep;55(3):793–798. doi: 10.1152/jappl.1983.55.3.793. [DOI] [PubMed] [Google Scholar]
- Hwang J. C., St John W. M., Bartlett D., Jr Respiratory-related hypoglossal nerve activity: influence of anesthetics. J Appl Physiol Respir Environ Exerc Physiol. 1983 Sep;55(3):785–792. doi: 10.1152/jappl.1983.55.3.785. [DOI] [PubMed] [Google Scholar]
- Jansen A. H., Chernick V. Development of respiratory control. Physiol Rev. 1983 Apr;63(2):437–483. doi: 10.1152/physrev.1983.63.2.437. [DOI] [PubMed] [Google Scholar]
- Johnson S. M., Smith J. C., Funk G. D., Feldman J. L. Pacemaker behavior of respiratory neurons in medullary slices from neonatal rat. J Neurophysiol. 1994 Dec;72(6):2598–2608. doi: 10.1152/jn.1994.72.6.2598. [DOI] [PubMed] [Google Scholar]
- Kleckner N. W., Dingledine R. Regulation of hippocampal NMDA receptors by magnesium and glycine during development. Brain Res Mol Brain Res. 1991 Sep;11(2):151–159. [PubMed] [Google Scholar]
- Laurie D. J., Wisden W., Seeburg P. H. The distribution of thirteen GABAA receptor subunit mRNAs in the rat brain. III. Embryonic and postnatal development. J Neurosci. 1992 Nov;12(11):4151–4172. doi: 10.1523/JNEUROSCI.12-11-04151.1992. [DOI] [PMC free article] [PubMed] [Google Scholar]
- Onimaru H., Arata A., Homma I. Inhibitory synaptic inputs to the respiratory rhythm generator in the medulla isolated from newborn rats. Pflugers Arch. 1990 Dec;417(4):425–432. doi: 10.1007/BF00370663. [DOI] [PubMed] [Google Scholar]
- Onimaru H., Homma I. Whole cell recordings from respiratory neurons in the medulla of brainstem-spinal cord preparations isolated from newborn rats. Pflugers Arch. 1992 Mar;420(3-4):399–406. doi: 10.1007/BF00374476. [DOI] [PubMed] [Google Scholar]
- Paton J. F., Ramirez J. M., Richter D. W. Functionally intact in vitro preparation generating respiratory activity in neonatal and mature mammals. Pflugers Arch. 1994 Oct;428(3-4):250–260. doi: 10.1007/BF00724504. [DOI] [PubMed] [Google Scholar]
- Paton J. F., Richter D. W. Maturational changes in the respiratory rhythm generator of the mouse. Pflugers Arch. 1995 May;430(1):115–124. doi: 10.1007/BF00373846. [DOI] [PubMed] [Google Scholar]
- Paton J. F., Richter D. W. Role of fast inhibitory synaptic mechanisms in respiratory rhythm generation in the maturing mouse. J Physiol. 1995 Apr 15;484(Pt 2):505–521. doi: 10.1113/jphysiol.1995.sp020682. [DOI] [PMC free article] [PubMed] [Google Scholar]
- Schmid K., Böhmer G., Gebauer K. Glycine receptor-mediated fast synaptic inhibition in the brainstem respiratory system. Respir Physiol. 1991 Jun;84(3):351–361. doi: 10.1016/0034-5687(91)90129-7. [DOI] [PubMed] [Google Scholar]
- Smart T. G., Xie X., Krishek B. J. Modulation of inhibitory and excitatory amino acid receptor ion channels by zinc. Prog Neurobiol. 1994 Feb;42(3):393–441. doi: 10.1016/0301-0082(94)90082-5. [DOI] [PubMed] [Google Scholar]
- Smith J. C., Ellenberger H. H., Ballanyi K., Richter D. W., Feldman J. L. Pre-Bötzinger complex: a brainstem region that may generate respiratory rhythm in mammals. Science. 1991 Nov 1;254(5032):726–729. doi: 10.1126/science.1683005. [DOI] [PMC free article] [PubMed] [Google Scholar]
- Smith J. C., Greer J. J., Liu G. S., Feldman J. L. Neural mechanisms generating respiratory pattern in mammalian brain stem-spinal cord in vitro. I. Spatiotemporal patterns of motor and medullary neuron activity. J Neurophysiol. 1990 Oct;64(4):1149–1169. doi: 10.1152/jn.1990.64.4.1149. [DOI] [PubMed] [Google Scholar]
- Suzue T. Respiratory rhythm generation in the in vitro brain stem-spinal cord preparation of the neonatal rat. J Physiol. 1984 Sep;354:173–183. doi: 10.1113/jphysiol.1984.sp015370. [DOI] [PMC free article] [PubMed] [Google Scholar]
- Takahashi T., Momiyama A., Hirai K., Hishinuma F., Akagi H. Functional correlation of fetal and adult forms of glycine receptors with developmental changes in inhibitory synaptic receptor channels. Neuron. 1992 Dec;9(6):1155–1161. doi: 10.1016/0896-6273(92)90073-m. [DOI] [PubMed] [Google Scholar]
- Trippenbach T., Richter D. W., Acker H. Hypoxia and ion activities within the brain stem of newborn rabbits. J Appl Physiol (1985) 1990 Jun;68(6):2494–2503. doi: 10.1152/jappl.1990.68.6.2494. [DOI] [PubMed] [Google Scholar]
- Viana F., Bayliss D. A., Berger A. J. Postnatal changes in rat hypoglossal motoneuron membrane properties. Neuroscience. 1994 Mar;59(1):131–148. doi: 10.1016/0306-4522(94)90105-8. [DOI] [PubMed] [Google Scholar]
- Withington-Wray D. J., Mifflin S. W., Spyer K. M. Intracellular analysis of respiratory-modulated hypoglossal motoneurons in the cat. Neuroscience. 1988 Jun;25(3):1041–1051. doi: 10.1016/0306-4522(88)90057-7. [DOI] [PubMed] [Google Scholar]