Abstract
1. In studies of the central neural control of breathing, little advantage has been taken of comparative approaches. We have developed an in vitro brainstem preparation using larval Rana catesbeiana which generates two rhythmic neural activities characteristic of lung and gill ventilation. Based on the pattern of the facial (VII) nerve activity both lung and gill rhythm-related respiratory cycles were divided into three distinct phases. The purpose of this study was to characterize and classify membrane potential trajectories of respiratory motoneurons in the VII nucleus at intermediate stages (XII-XVII) of development. 2. Seventy-five respiratory-modulated neurons were recorded intracellularly within the facial motor nucleus region. Their resting membrane potential was between -40 and -80 mV. Sixty of them were identified as VII motoneurons and fifteen were non-antidromically activated. Membrane potentials of fifty-six of the seventy-five neurons were modulated with both lung (5-27 mV) and gill rhythms (3-15 mV) and the remaining nineteen neurons had only a modulation with lung rhythmicity (6-23 mV). No cells with gill modulation alone were observed. 3. All of the cells modulated with lung rhythmicity had only phase-bound depolarizing or hyperpolarizing membrane potential swings which could be categorized into four distinct patterns. In contrast, of the fifty-six cells modulated with gill rhythmicity, thirty-two were phasically depolarized during distinct phases of the gill cycle (four patterns were distinguished), whereas the remaining twenty-four were phase spanning with two distinct patterns. The magnitudes of lung and gill modulations were proportionally related to each other in the cells modulated with both rhythms. 4. In all sixteen neurons studied, a reduction or a reversal of phasic inhibitory inputs during a portion of the lung or gill respiratory cycle was observed following a negative current or chloride ion (Cl-) injection. The phasic membrane resistance modulation in relation to the gill rhythm was analysed in six neurons and a relative decrease in the somatic membrane resistance (0.7-8.1 M omega) was detected during the periods of hyperpolarization. 5. We propose that, at these intermediate stages of development: (a) both gill and lung respiratory oscillations in motoneurons are generated by respiratory premotor neurons having only a few distinct activity patterns; (b) these patterns delineate distinct portions of the centrally generated respiratory cycles; and (c) phasic synaptic inhibition, mediated by Cl-, contributes to shaping the membrane potential trajectories of respiratory motoneurons.
Full text
PDF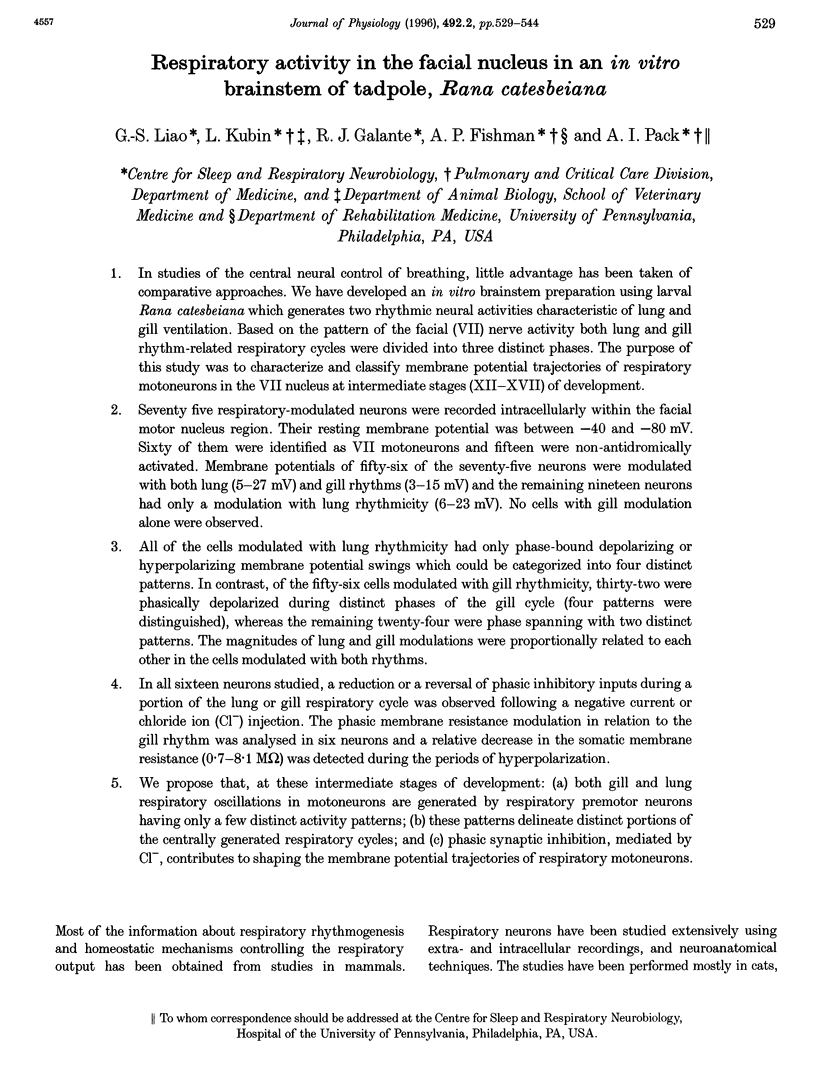
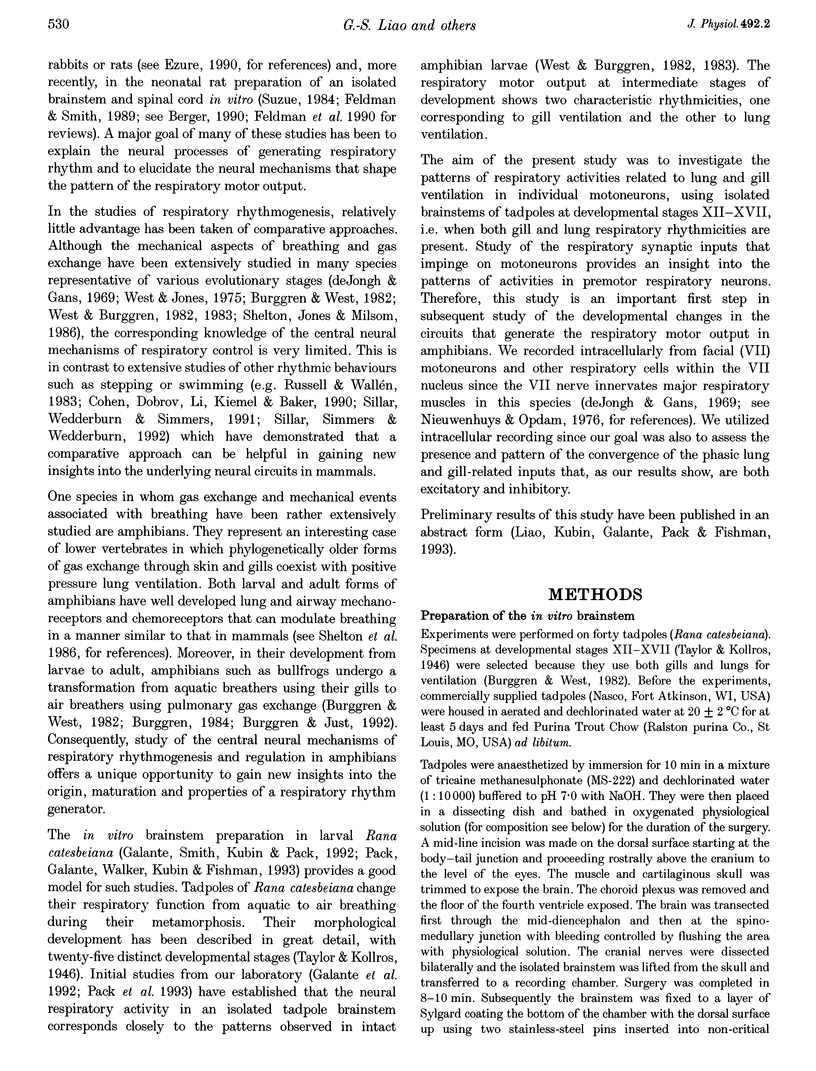
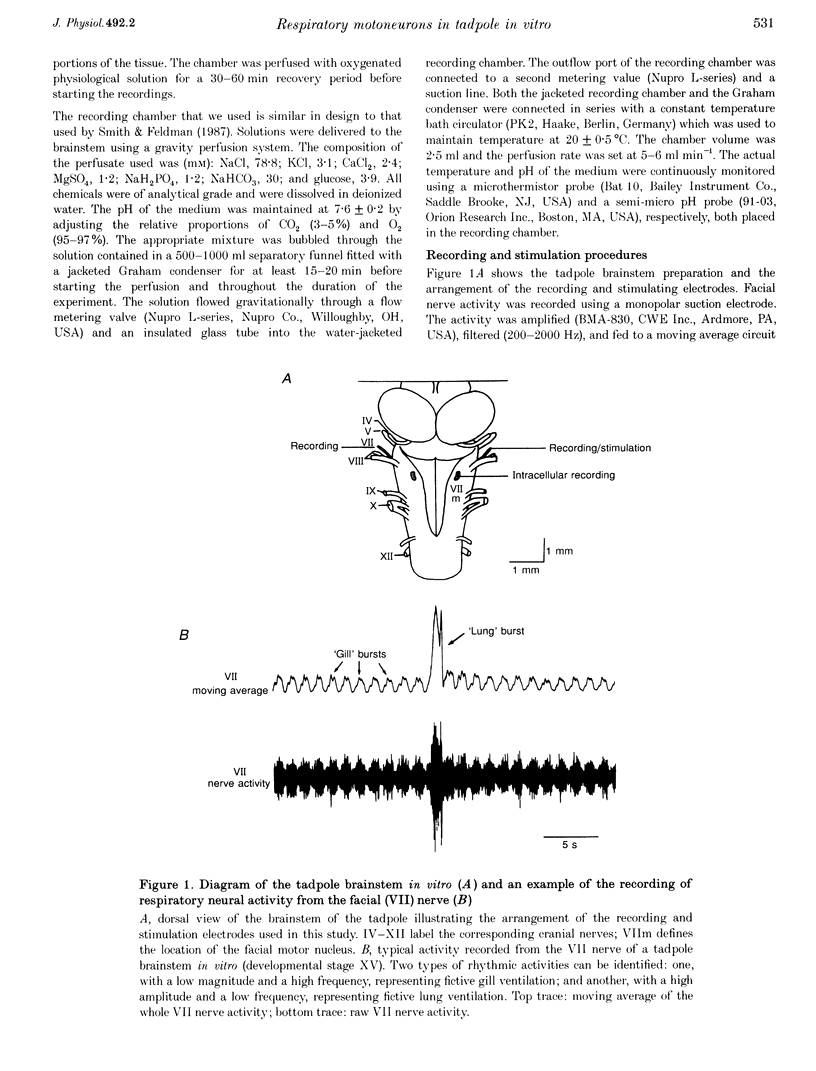
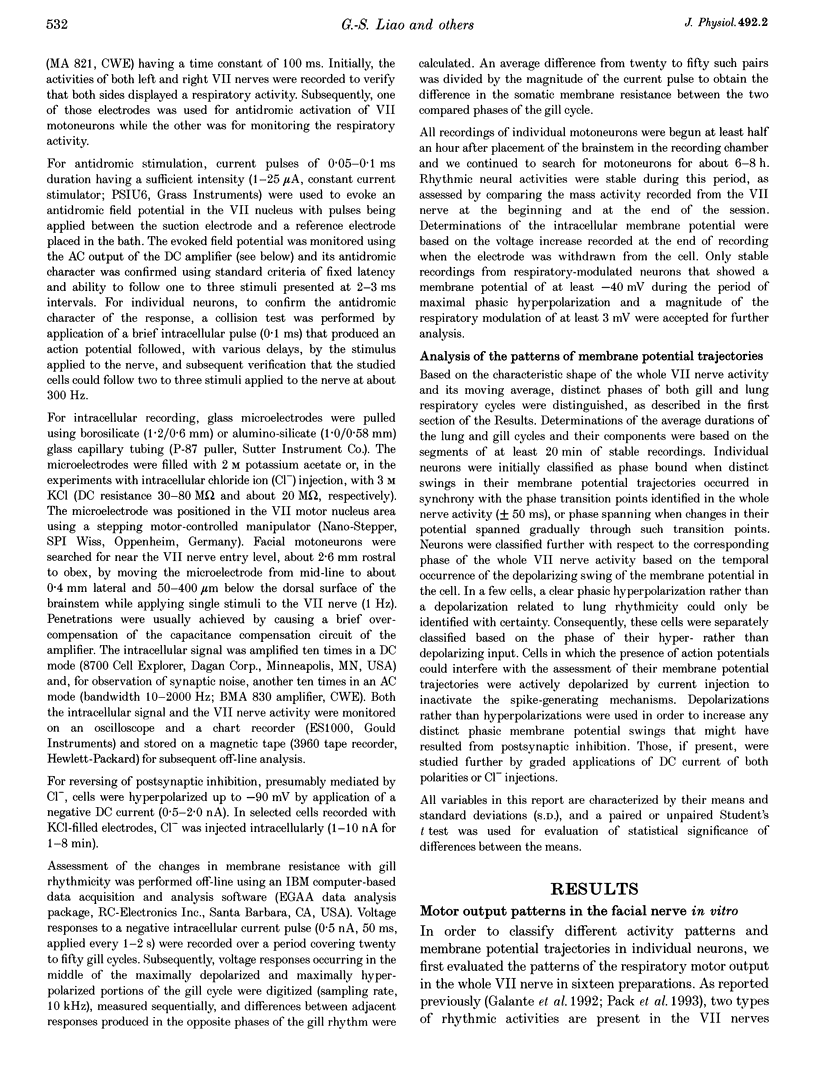
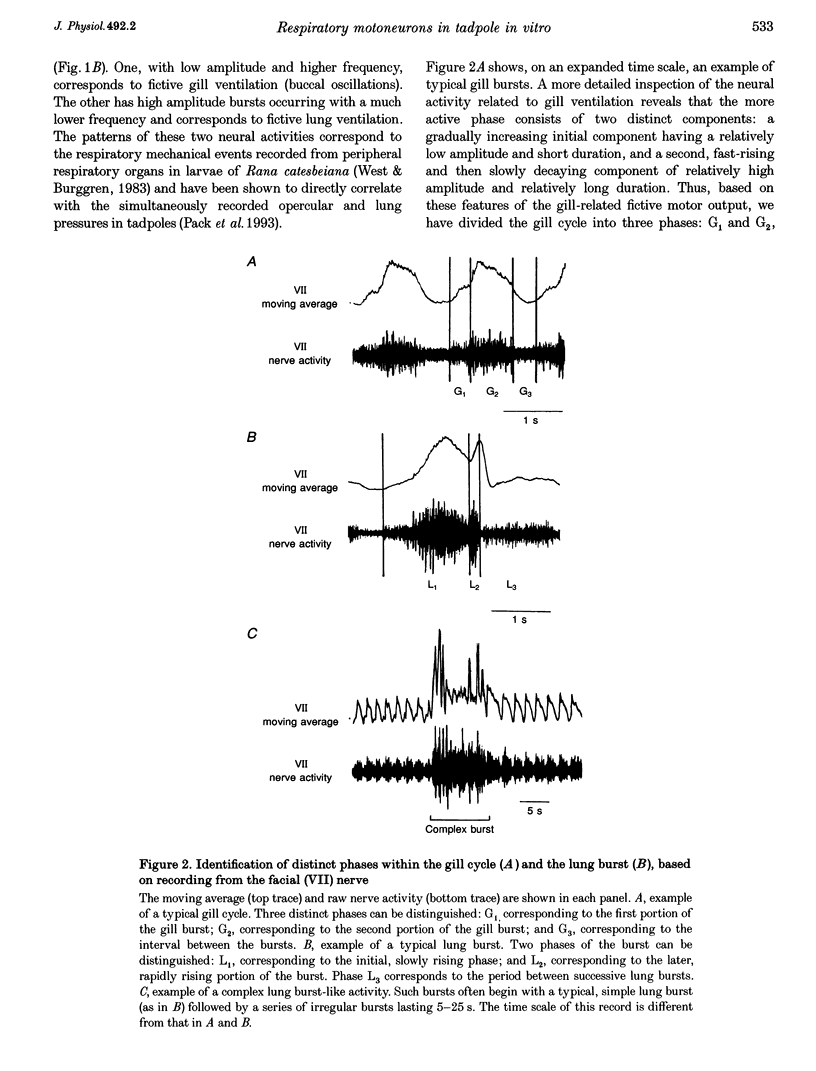

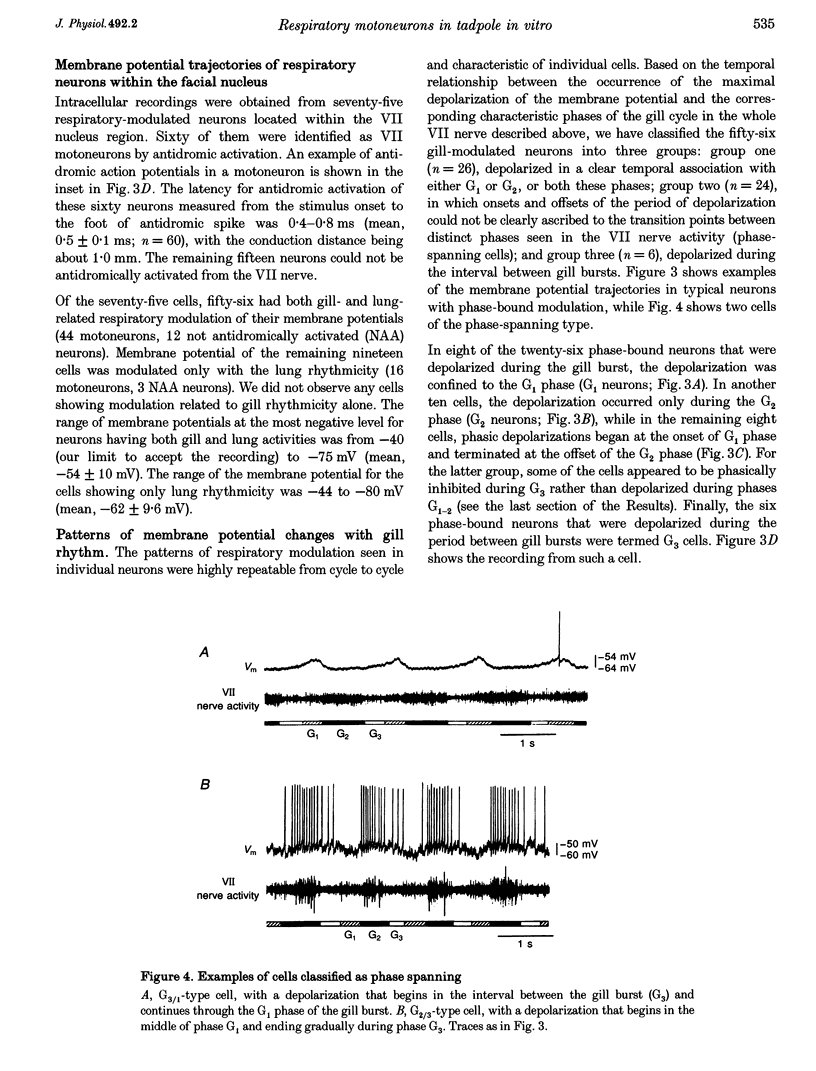
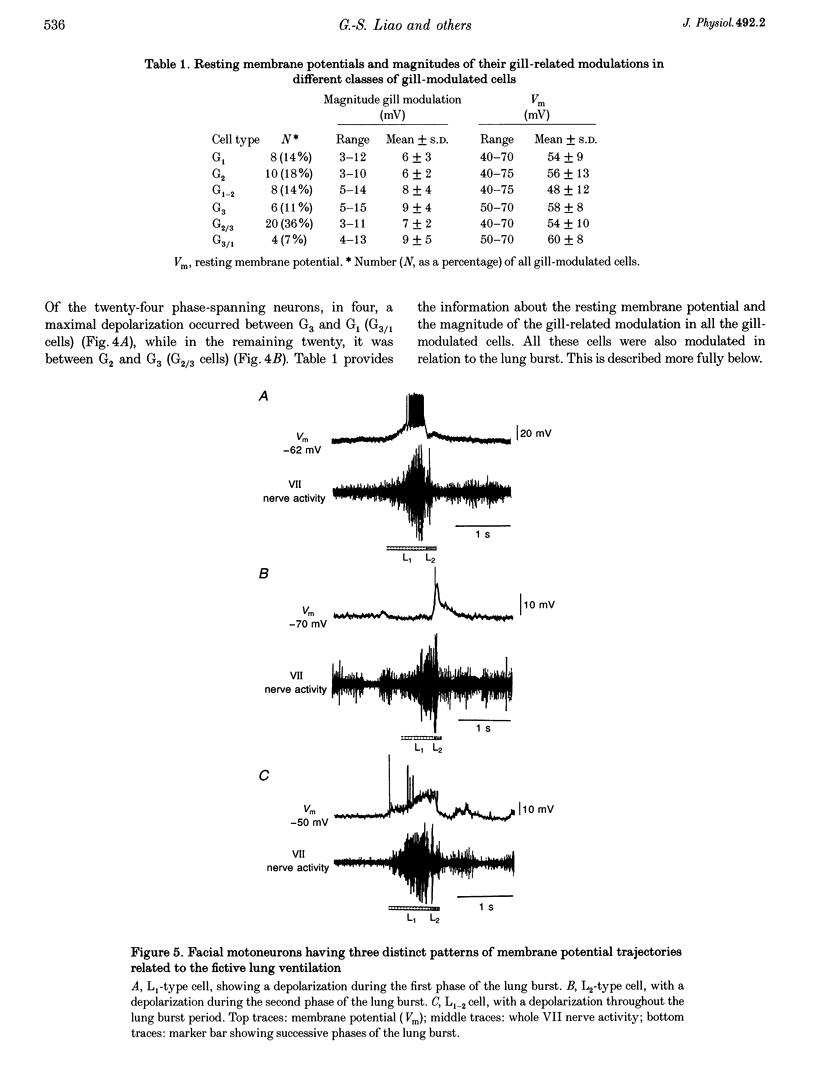
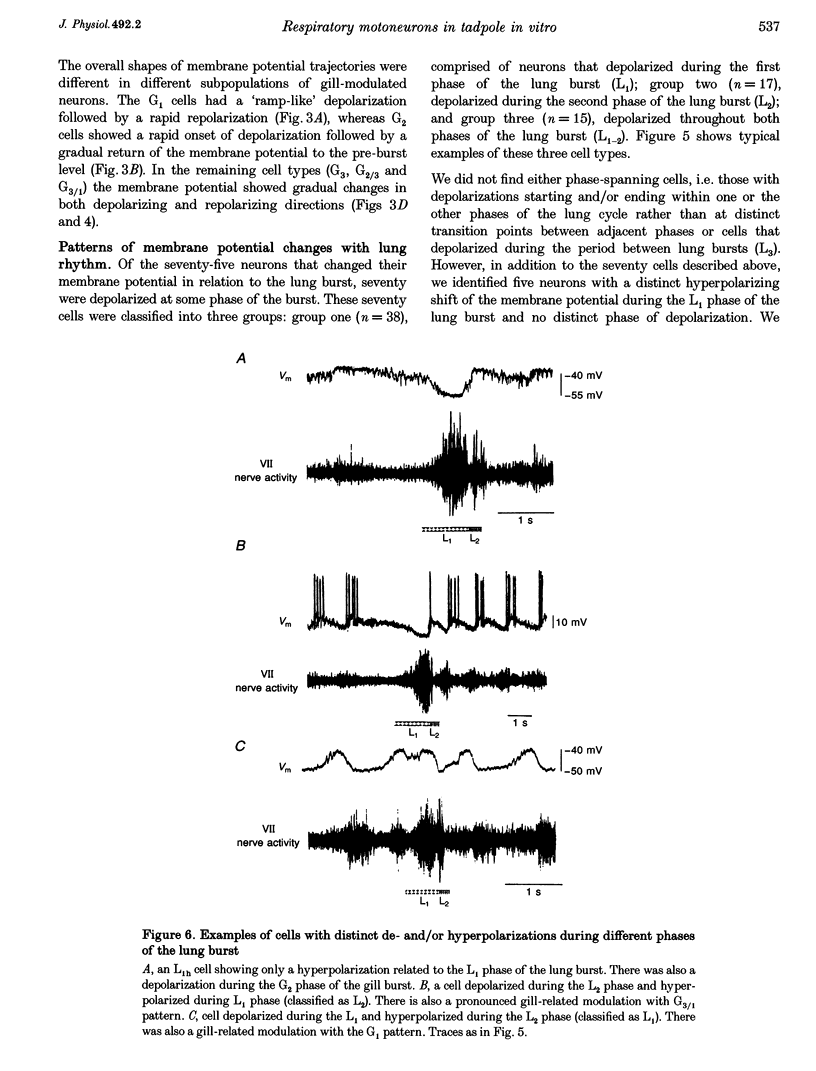
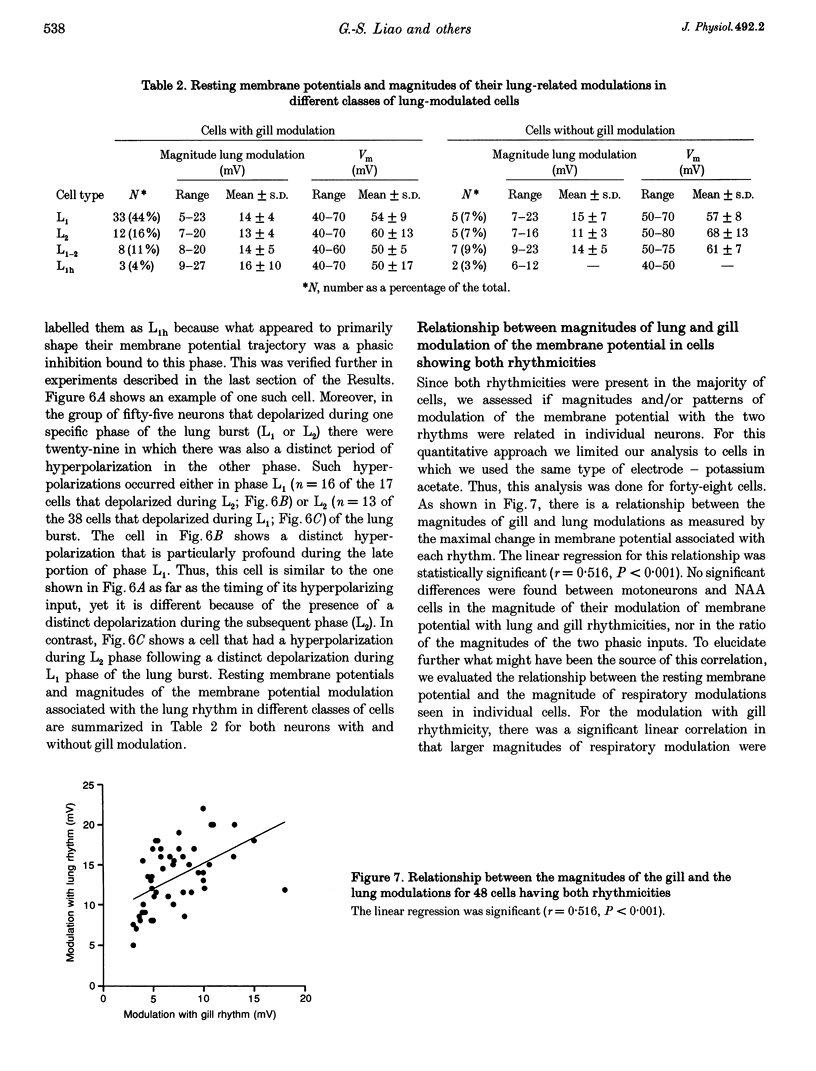
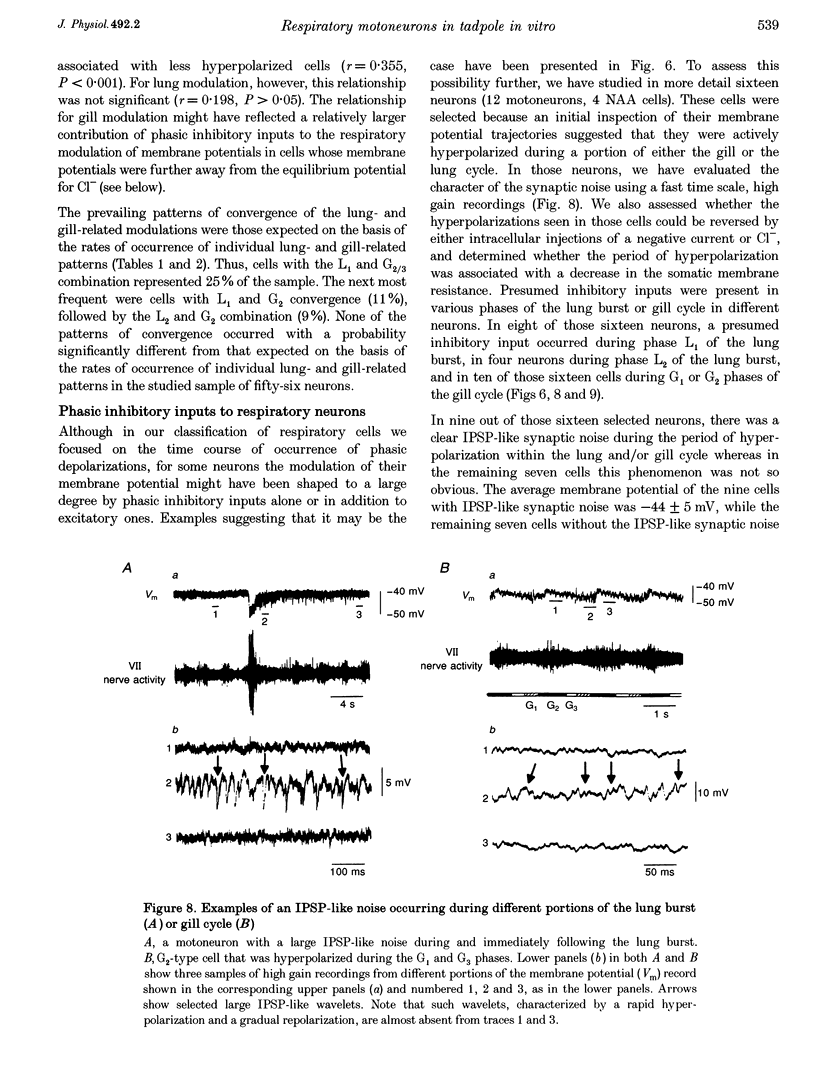
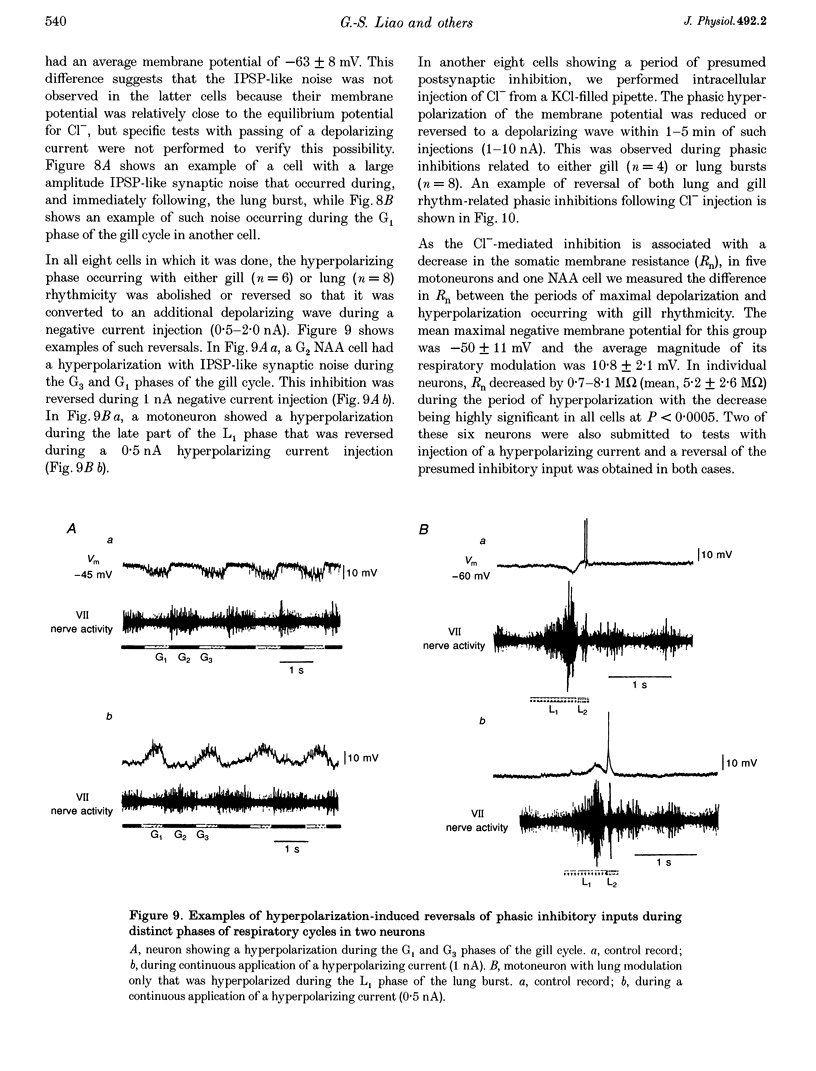
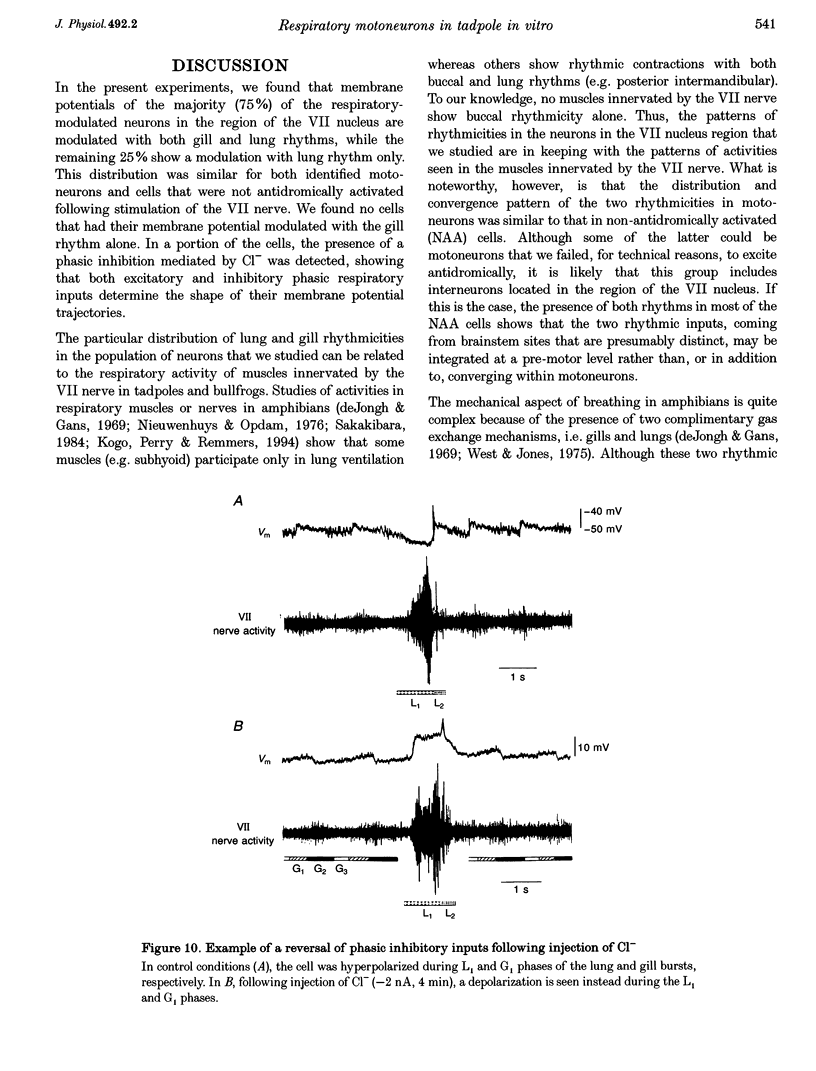
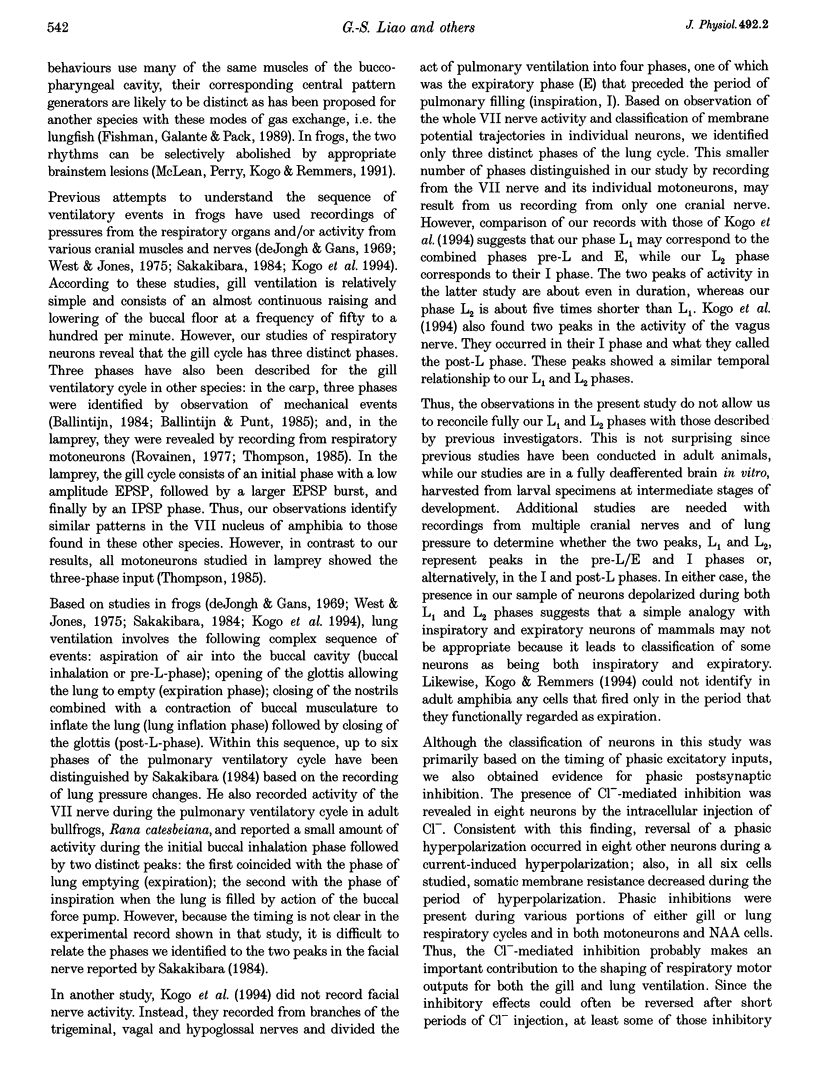
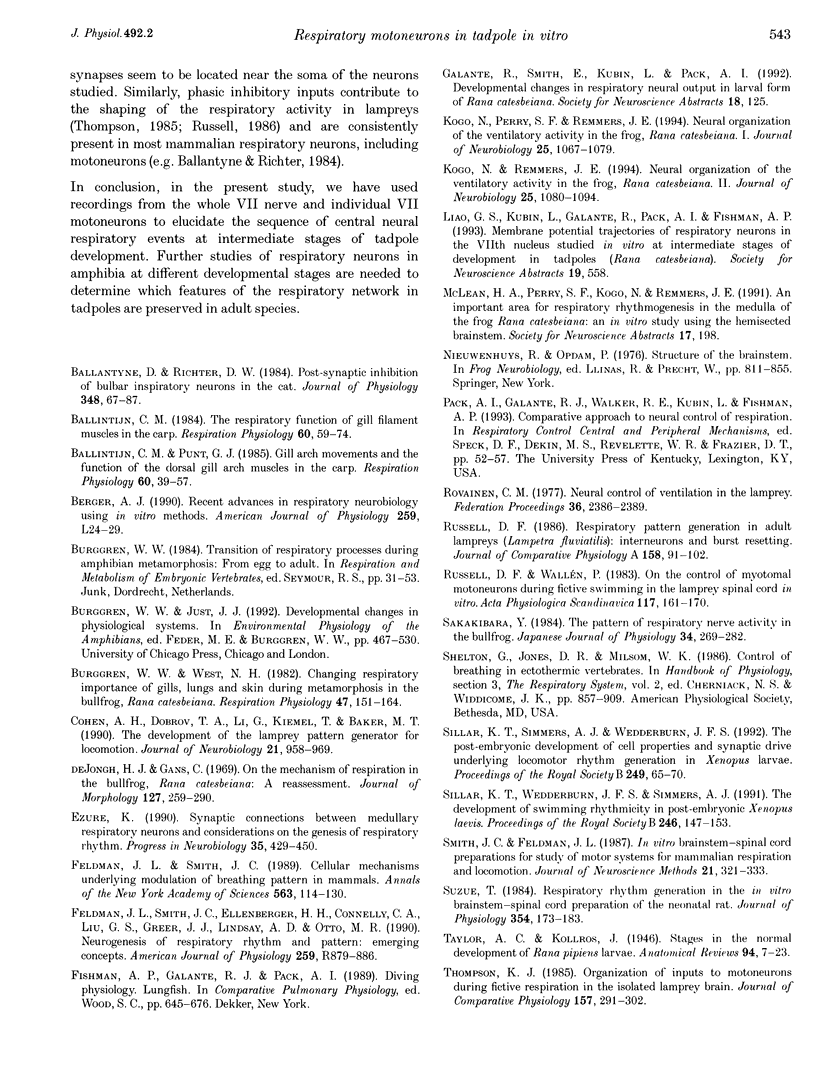
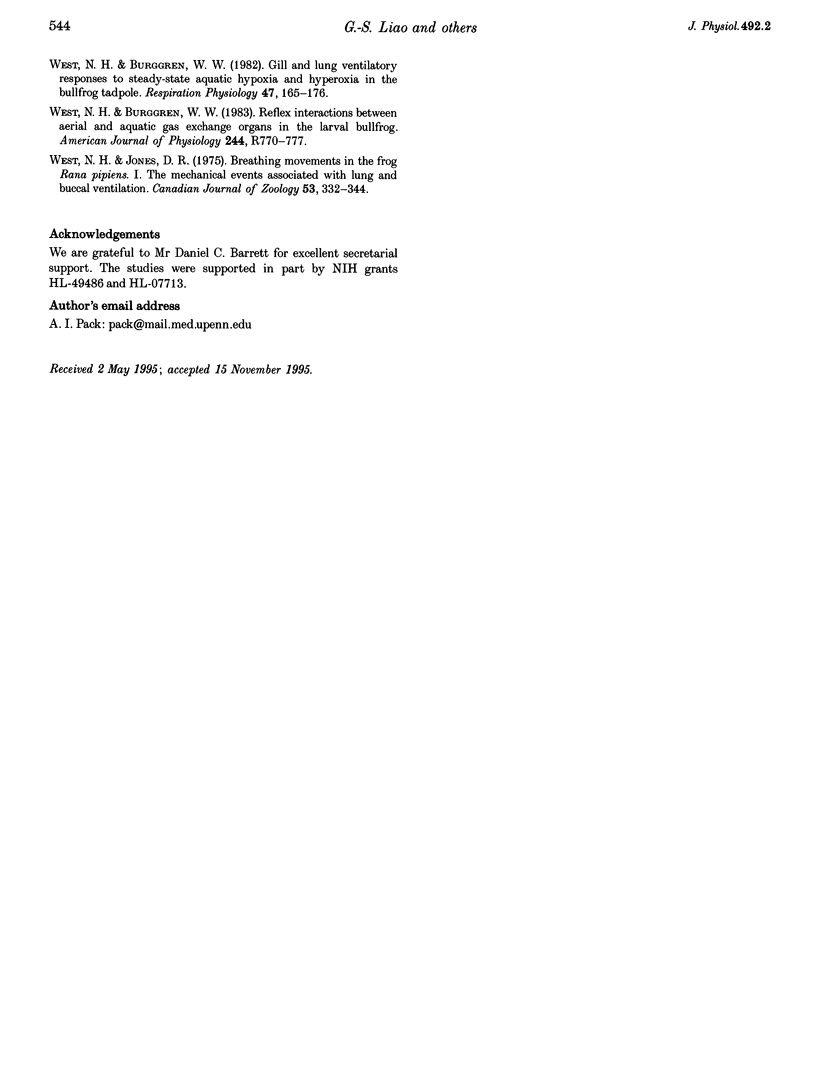
Selected References
These references are in PubMed. This may not be the complete list of references from this article.
- Ballantyne D., Richter D. W. Post-synaptic inhibition of bulbar inspiratory neurones in the cat. J Physiol. 1984 Mar;348:67–87. doi: 10.1113/jphysiol.1984.sp015100. [DOI] [PMC free article] [PubMed] [Google Scholar]
- Ballintijn C. M., Punt G. J. Gill arch movements and the function of the dorsal gill arch muscles in the carp. Respir Physiol. 1985 Apr;60(1):39–57. doi: 10.1016/0034-5687(85)90038-6. [DOI] [PubMed] [Google Scholar]
- Ballintijn C. M. The respiratory function of gill filament muscles in the carp. Respir Physiol. 1985 Apr;60(1):59–74. doi: 10.1016/0034-5687(85)90039-8. [DOI] [PubMed] [Google Scholar]
- Cohen A. H., Dobrov T. A., Li G., Kiemel T., Baker M. T. The development of the lamprey pattern generator for locomotion. J Neurobiol. 1990 Oct;21(7):958–969. doi: 10.1002/neu.480210703. [DOI] [PubMed] [Google Scholar]
- Feldman J. L., Smith J. C. Cellular mechanisms underlying modulation of breathing pattern in mammals. Ann N Y Acad Sci. 1989;563:114–130. doi: 10.1111/j.1749-6632.1989.tb42194.x. [DOI] [PubMed] [Google Scholar]
- Feldman J. L., Smith J. C., Ellenberger H. H., Connelly C. A., Liu G. S., Greer J. J., Lindsay A. D., Otto M. R. Neurogenesis of respiratory rhythm and pattern: emerging concepts. Am J Physiol. 1990 Nov;259(5 Pt 2):R879–R886. doi: 10.1152/ajpregu.1990.259.5.R879. [DOI] [PubMed] [Google Scholar]
- Kogo N., Perry S. F., Remmers J. E. Neural organization of the ventilatory activity in the frog, Rana catesbeiana. I. J Neurobiol. 1994 Sep;25(9):1067–1079. doi: 10.1002/neu.480250904. [DOI] [PubMed] [Google Scholar]
- Kogo N., Remmers J. E. Neural organization of the ventilatory activity in the frog, Rana catesbeiana. II. J Neurobiol. 1994 Sep;25(9):1080–1094. doi: 10.1002/neu.480250905. [DOI] [PubMed] [Google Scholar]
- Rovainen C. M. Neural control of ventilation in the lamprey. Fed Proc. 1977 Sep;36(10):2386–2389. [PubMed] [Google Scholar]
- Russell D. F. Respiratory pattern generation in adult lampreys (Lampetra fluviatilis): interneurons and burst resetting. J Comp Physiol A. 1986 Jan;158(1):91–102. doi: 10.1007/BF00614523. [DOI] [PubMed] [Google Scholar]
- Russell D. F., Wallén P. On the control of myotomal motoneurones during "fictive swimming" in the lamprey spinal cord in vitro. Acta Physiol Scand. 1983 Feb;117(2):161–170. doi: 10.1111/j.1748-1716.1983.tb07193.x. [DOI] [PubMed] [Google Scholar]
- Sillar K. T., Simmers A. J., Wedderburn J. F. The post-embryonic development of cell properties and synaptic drive underlying locomotor rhythm generation in Xenopus larvae. Proc Biol Sci. 1992 Jul 22;249(1324):65–70. doi: 10.1098/rspb.1992.0084. [DOI] [PubMed] [Google Scholar]
- Sillar K. T., Wedderburn J. F., Simmers A. J. The development of swimming rhythmicity in post-embryonic Xenopus laevis. Proc Biol Sci. 1991 Nov 22;246(1316):147–153. doi: 10.1098/rspb.1991.0137. [DOI] [PubMed] [Google Scholar]
- Suzue T. Respiratory rhythm generation in the in vitro brain stem-spinal cord preparation of the neonatal rat. J Physiol. 1984 Sep;354:173–183. doi: 10.1113/jphysiol.1984.sp015370. [DOI] [PMC free article] [PubMed] [Google Scholar]
- Thompson K. J. Organization of inputs to motoneurons during fictive respiration in the isolated lamprey brain. J Comp Physiol A. 1985 Oct;157(3):291–302. doi: 10.1007/BF00618119. [DOI] [PubMed] [Google Scholar]
- West N. H., Burggren W. W. Gill and lung ventilation responses to steady-state aquatic hypoxia and hyperoxia in the bullfrog tadpole. Respir Physiol. 1982 Feb;47(2):165–176. doi: 10.1016/0034-5687(82)90109-8. [DOI] [PubMed] [Google Scholar]
- West N. H., Burggren W. W. Reflex interactions between aerial and aquatic gas exchange organs in larval bullfrogs. Am J Physiol. 1983 Jun;244(6):R770–R777. doi: 10.1152/ajpregu.1983.244.6.R770. [DOI] [PubMed] [Google Scholar]
- West N. H., Jones D. R. Breathing movements in the frog Rana pipiens. I. The mechanical events associated with lung and buccal ventilation. Can J Zool. 1975 Mar;53(3):332–344. doi: 10.1139/z75-042. [DOI] [PubMed] [Google Scholar]