Abstract
1. The voltage dependence of phasic contraction was assessed in rabbit ventricular myocytes. Phasic contraction at all potentials was abolished by exposure to ryanodine-thapsigargin, showing that it was due primarily to Ca2+ release from the sarcoplasmic reticulum (SR). Experiments were performed at 35 degrees C, cells were whole-cell patch clamped and contraction was measured optically as unloaded shortening. Cells were held at -40 mV to inactivate the Na+ current (INa) and T-type Ca2+ current. A standard cellular Ca2+ load was established by applying a train of conditioning pulses at 0.5 Hz before each test pulse. The effect of replacing K+ with Cs+ in the dialysing pipette solution, and the effect of altering dialysing [Na+] between 0 and 20 mM, was assessed on contraction. 2. Cells dialysed with a K(+)-based, Na(+)-free solution exhibited a 'bell-shaped' voltage dependence of the L-type Ca2+ channel current (ICa,L), with a maximum ICa,L at +10 mV. Replacing internal K+ with Cs+, or altering pipette [Na+], did not affect the voltage dependence of ICa,L. 3. The voltage dependence of phasic contraction in cells dialysed with a K(+)-based solution was modulated by pipette [Na+]. The voltage dependence of phasic contraction was bell-shaped with 0 Na+, became much loss bell-shaped with 10 mM Na+ and with 20 mM Na+ the phasic contraction elicited at +100 mV was 1.6-fold larger than that at +10 mV. 4. Replacing 80% of K+ with Cs+ in the pipette dialysis solution led to a significant reduction in contraction amplitude and a more rapid decline in contraction amplitude after beginning the dialysis of the cell. 5. Cells dialysed with a Cs(+)-based solution displayed a voltage dependence of phasic contraction which was more bell-shaped (i.e. more similar to that of ICa,L) than that obtained with the corresponding K(+)-based dialysis solution. The level of pipette [Na+] still modulated the voltage dependence of phasic contraction in cells dialysed with a Cs(+)-based solution. 6. Time-to-peak contraction (tpk) also displayed voltage dependence; it had a minimum value between 0 and +20 mV (the voltage range for maximum ICa,L), but increased at more negative and positive potentials. Alteration of tpk contraction is discussed in relation to the stochastic behaviour of L-type Ca2+ channels and SR Ca2+ release channels. 7. The shape of the voltage dependence of contraction in rabbit myocytes at 35 degrees C is modulated by dialysing [Na+] over the tested range, 0-20 mM. Modulation of voltage dependence of contraction by dialysing [Na+] is consistent with an influence of reverse Na(+)-Ca2+ exchange in triggering intracellular Ca2+ release, in addition to the trigger Ca2+ which enters via ICa,L. 8. The marked effect of dialysing Cs+ on contraction amplitude, and on the voltage dependence of phasic contraction, does not appear to have been reported previously. Internal dialysis with Cs+ is a commonly used technique for blocking interfering outward K+ currents, in order to measure ICa,L more selectively. The present study suggests that Cs+ might also interfere with processes involved in excitation-contraction coupling and indicates that it might be wise to exercise caution with the use of internal Cs+ in experiments investigating excitation-contraction coupling.
Full text
PDF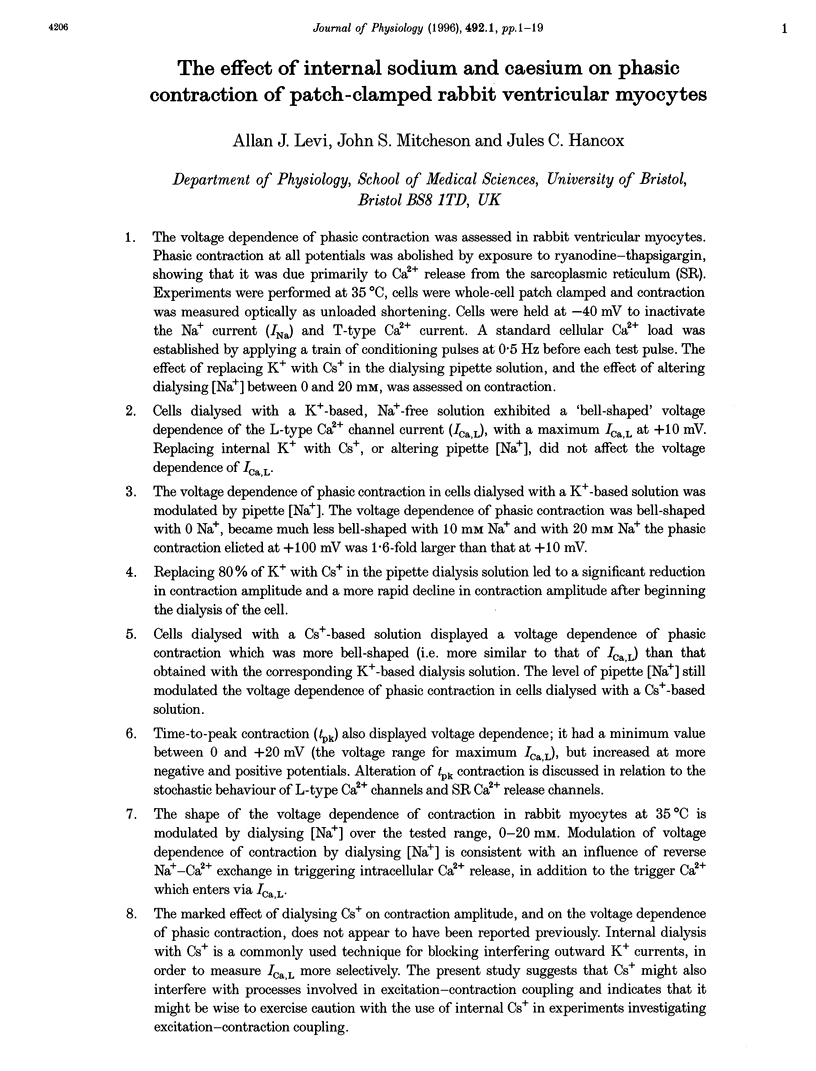
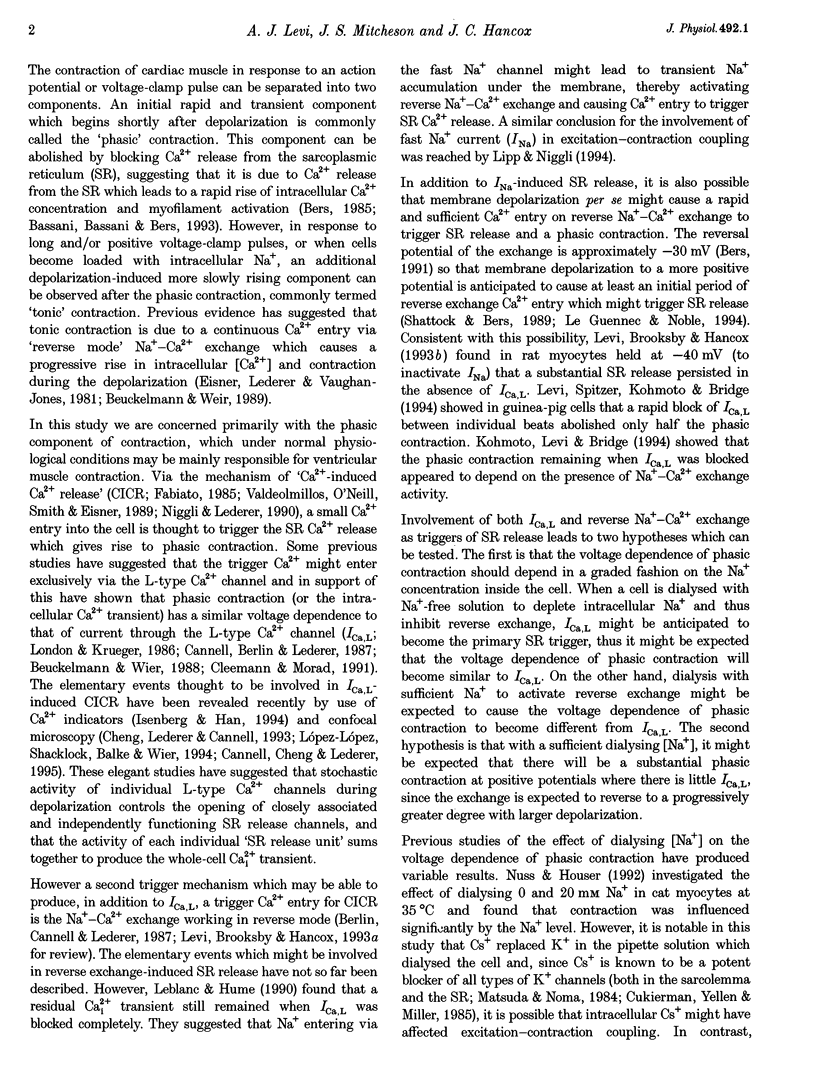
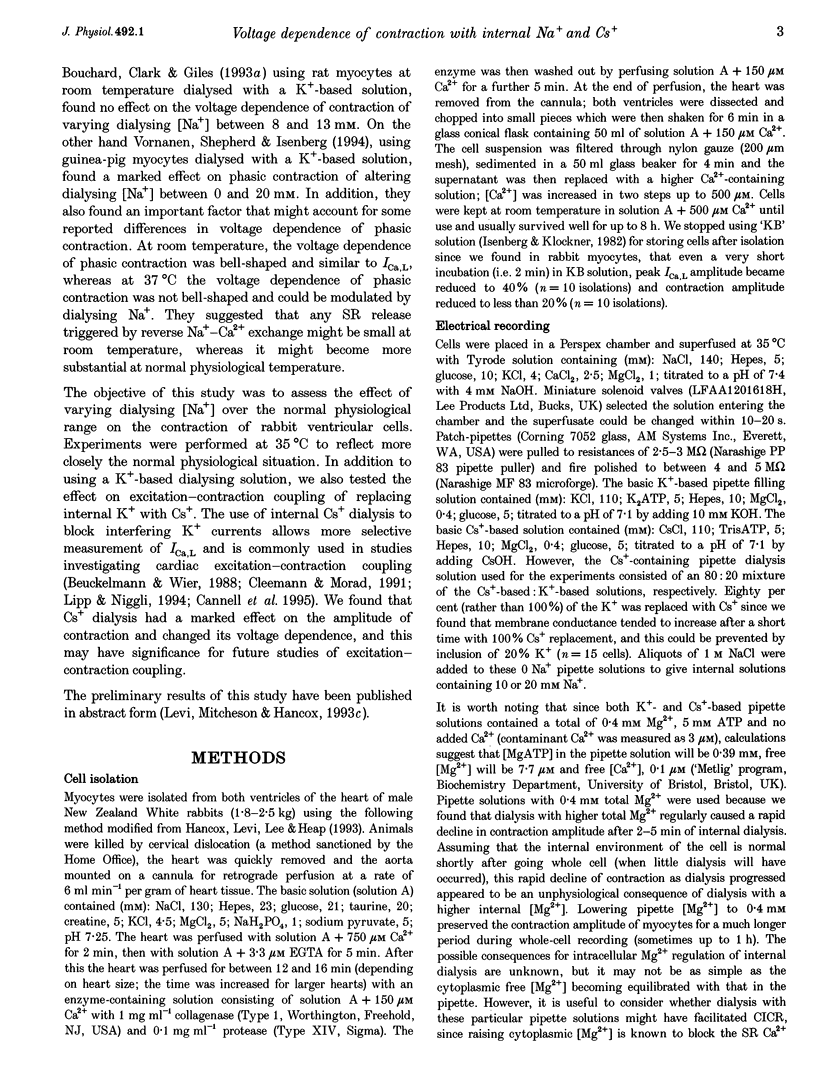
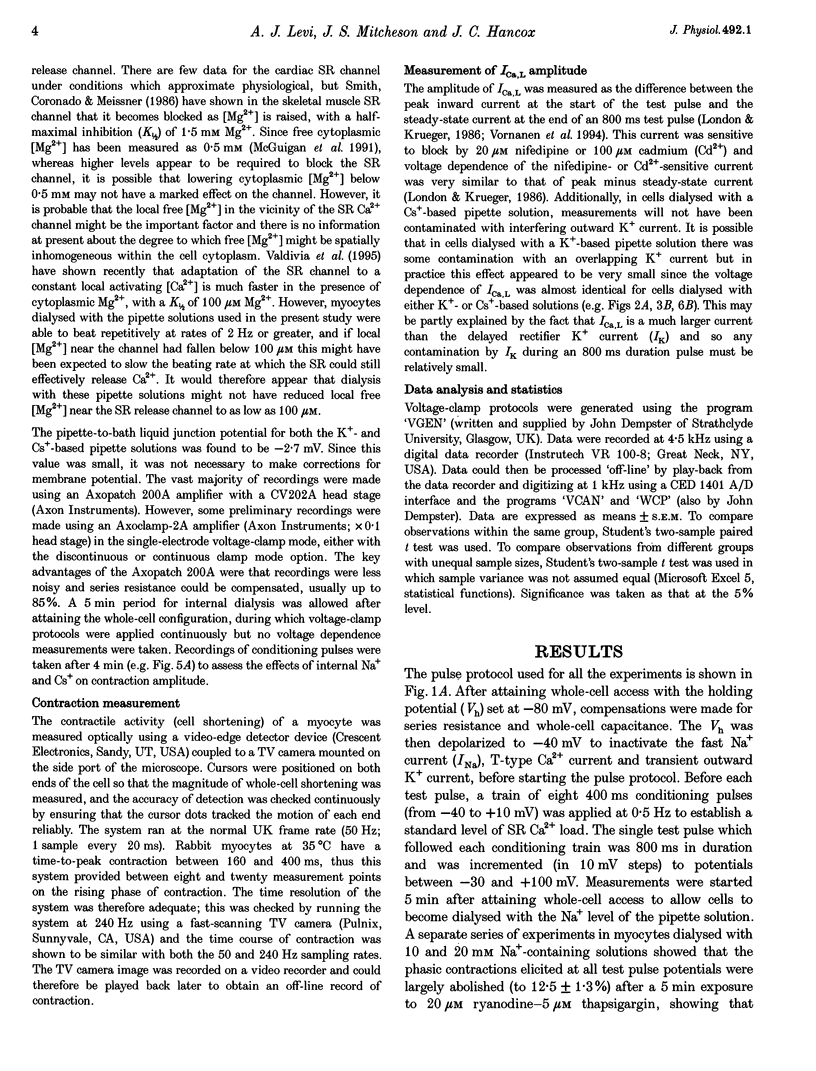
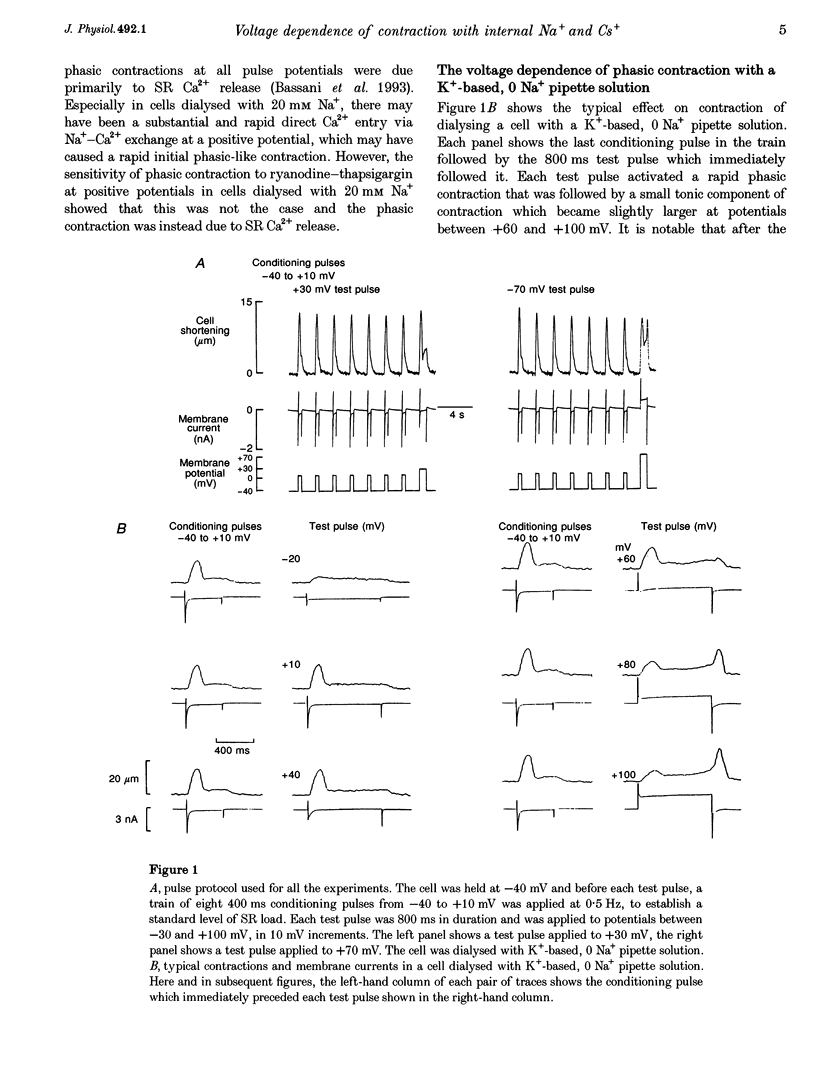
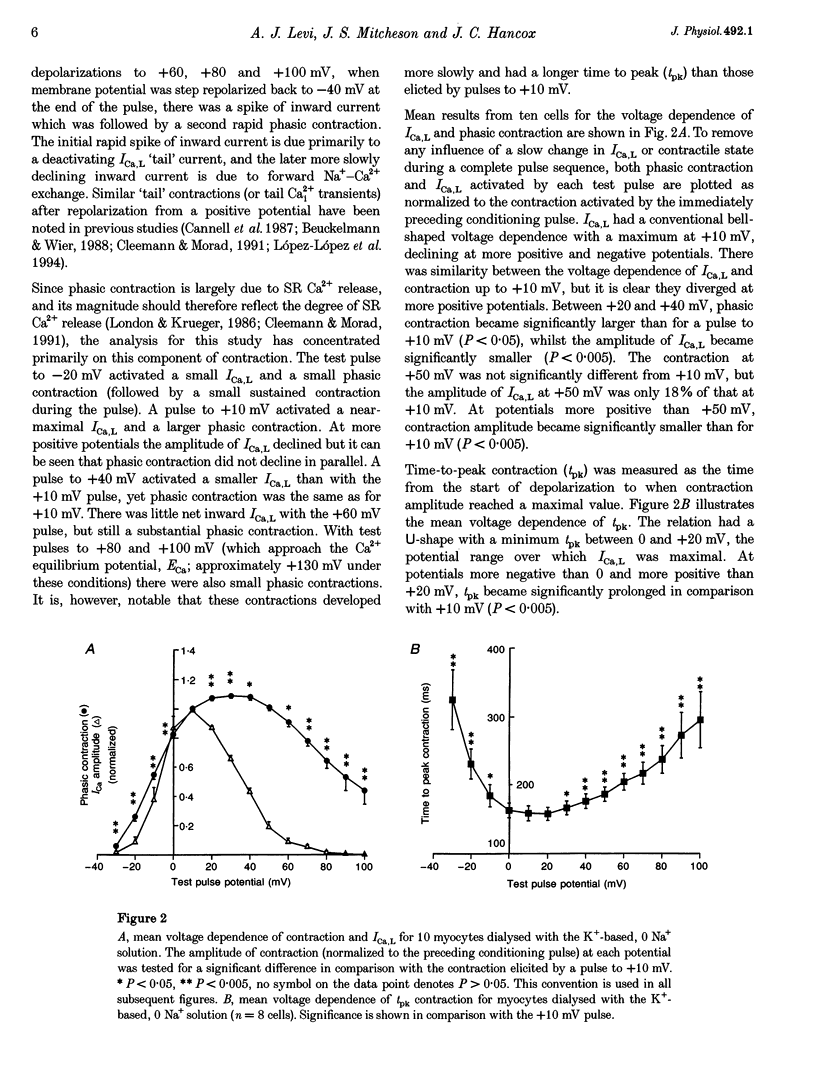
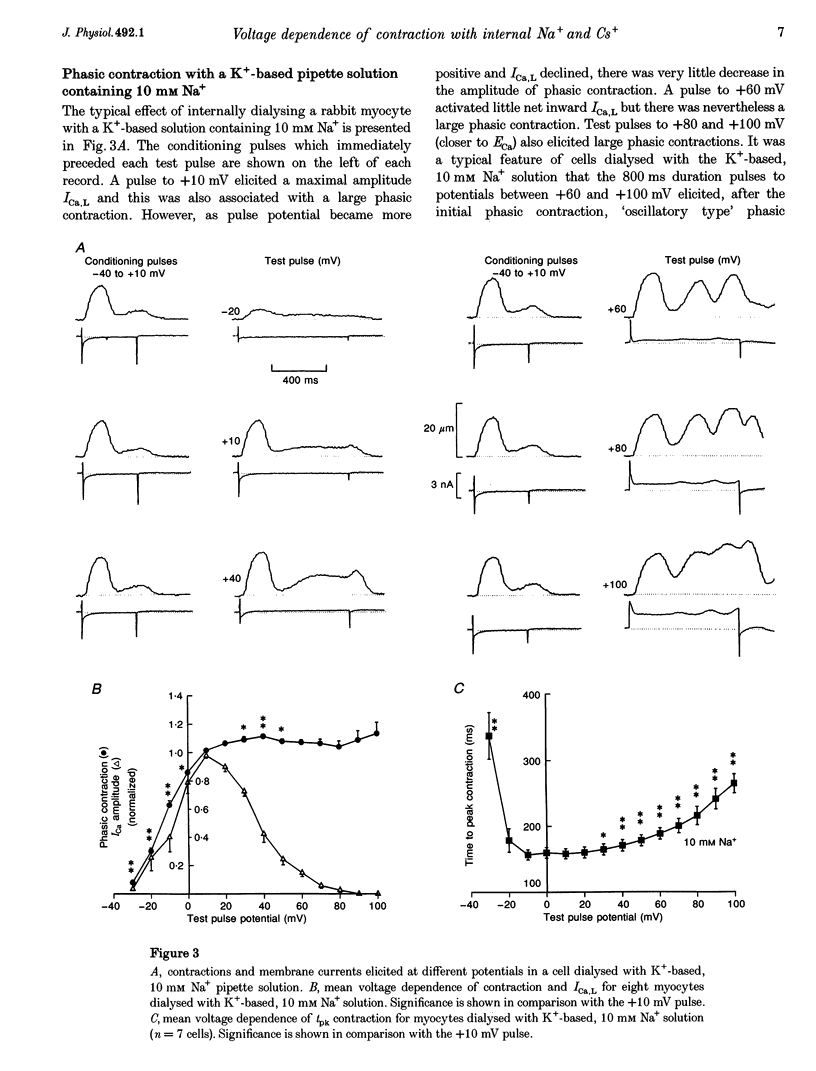
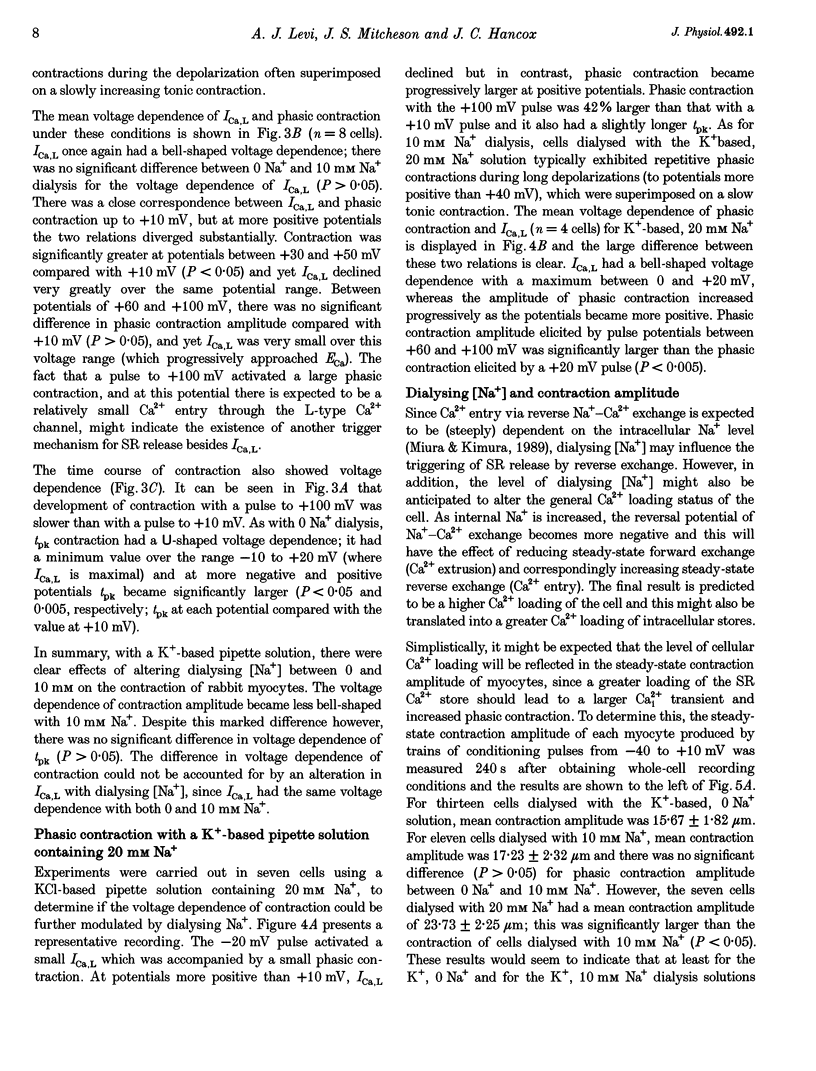
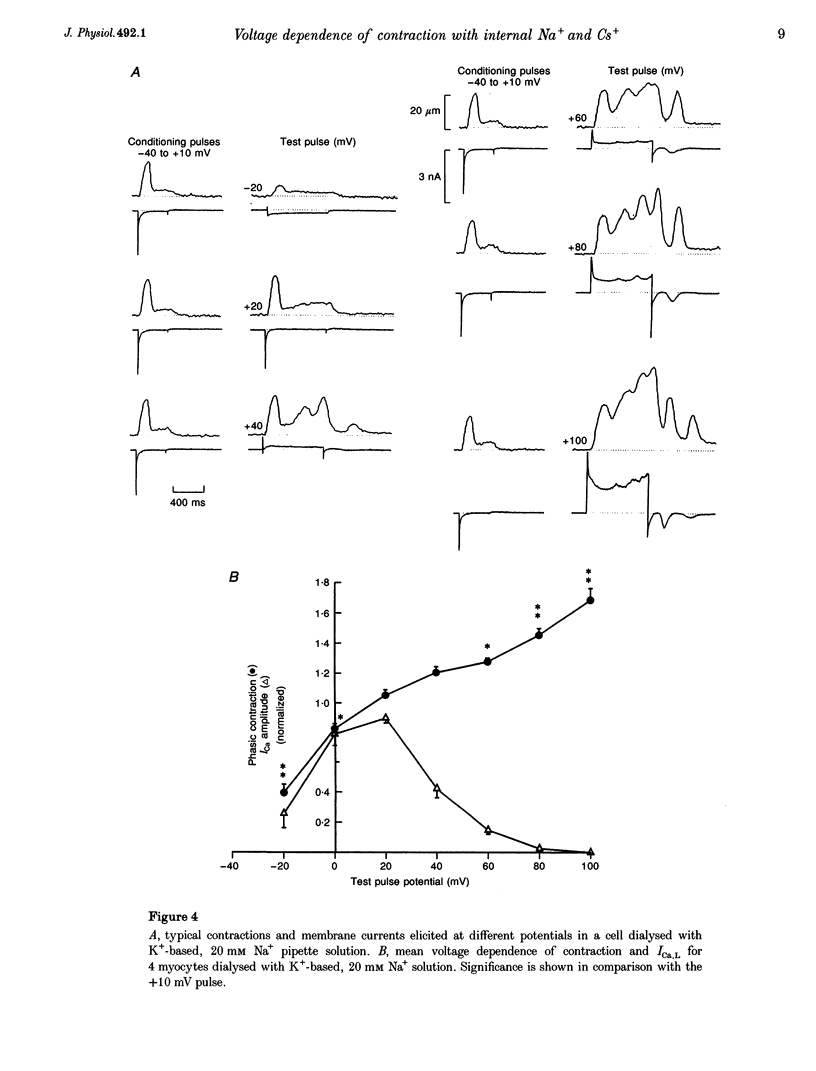
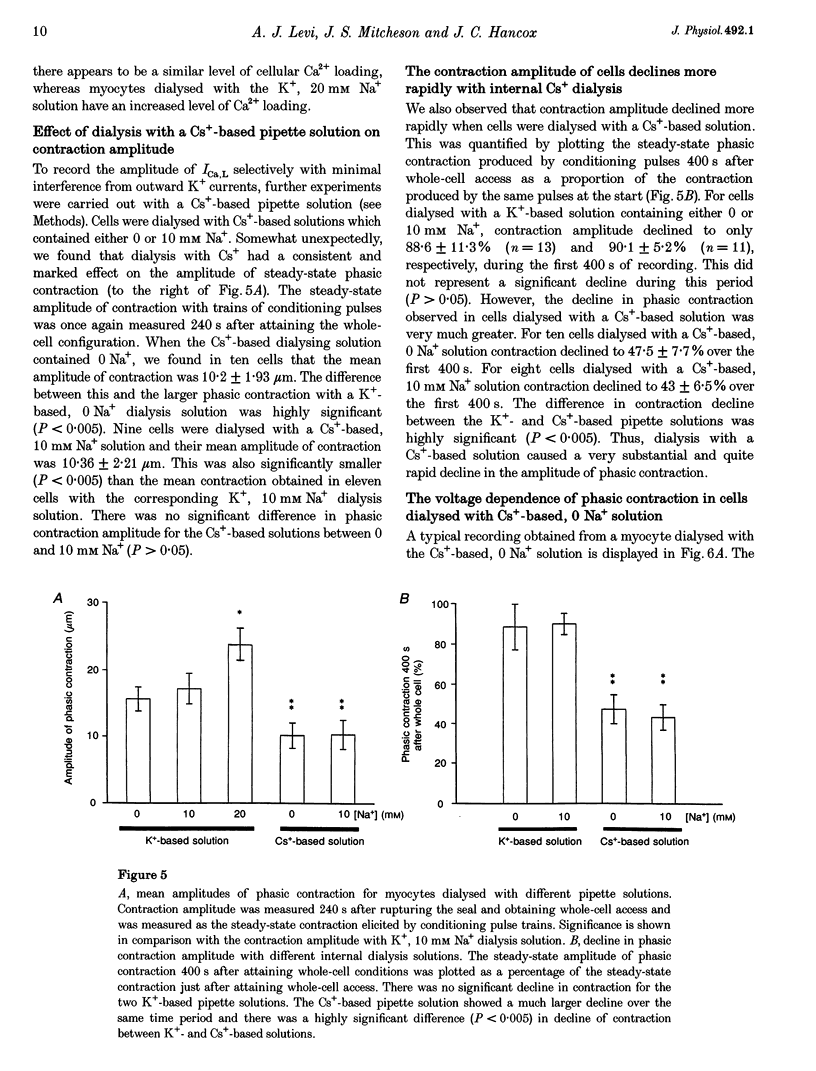
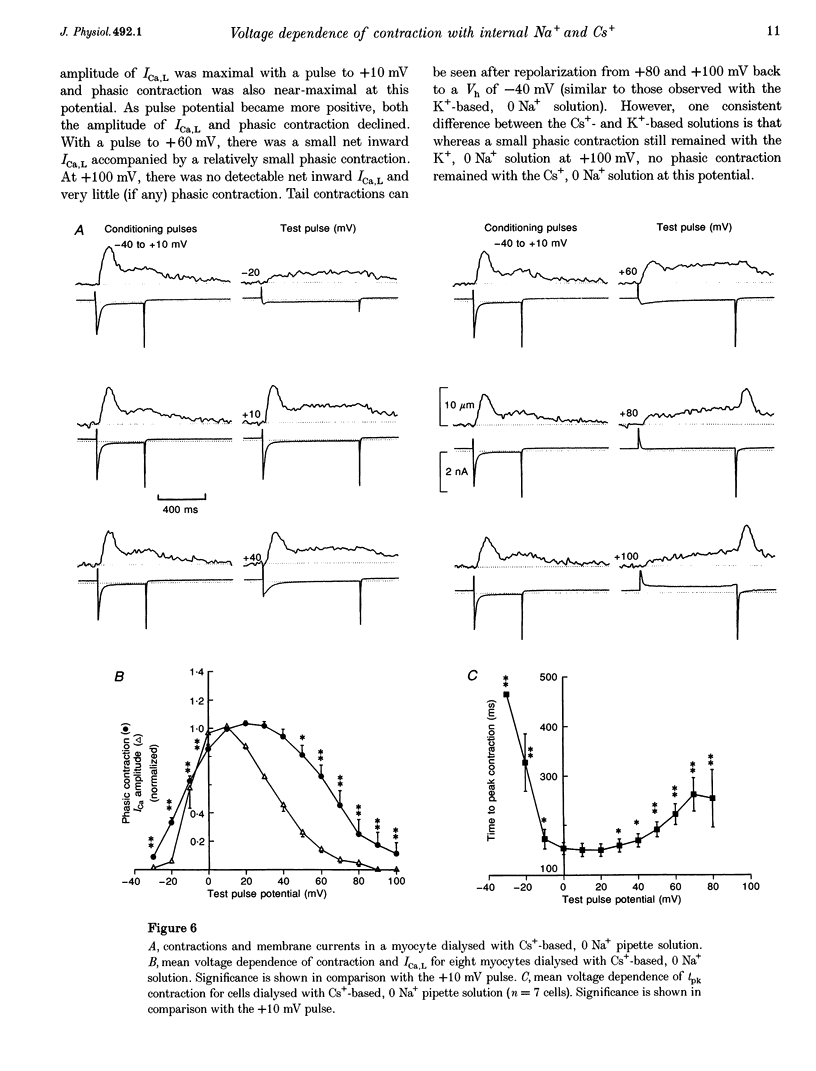
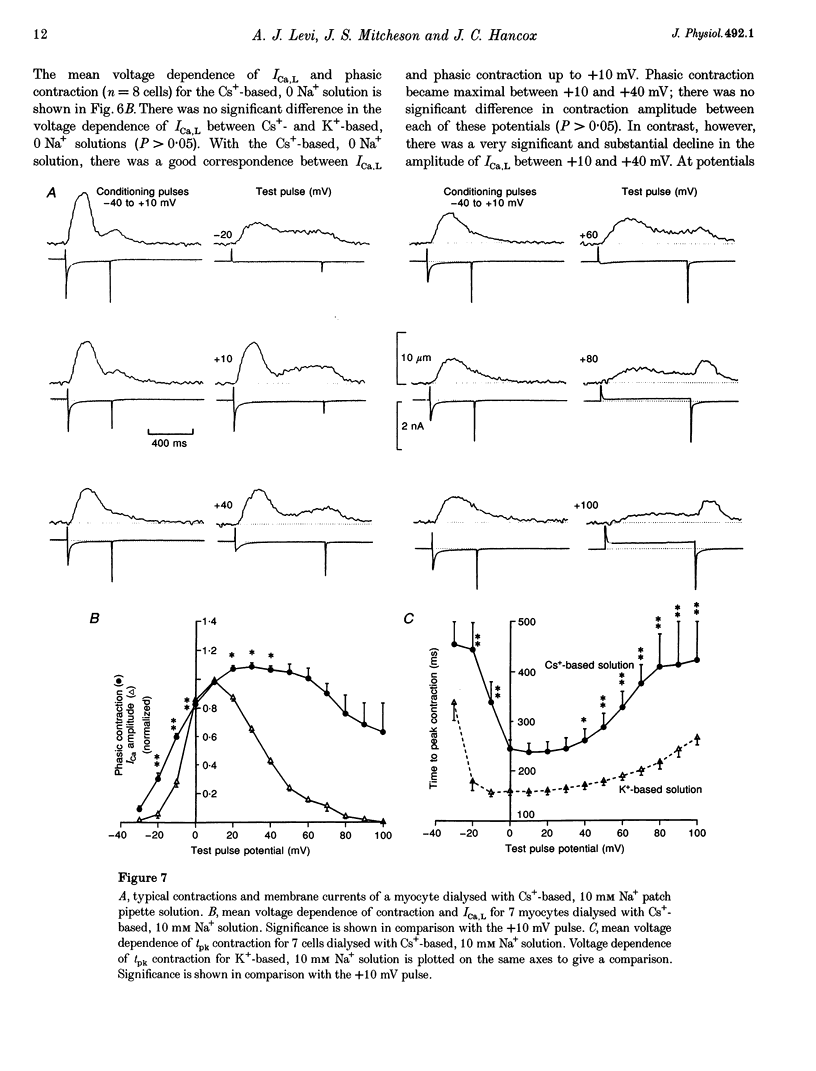
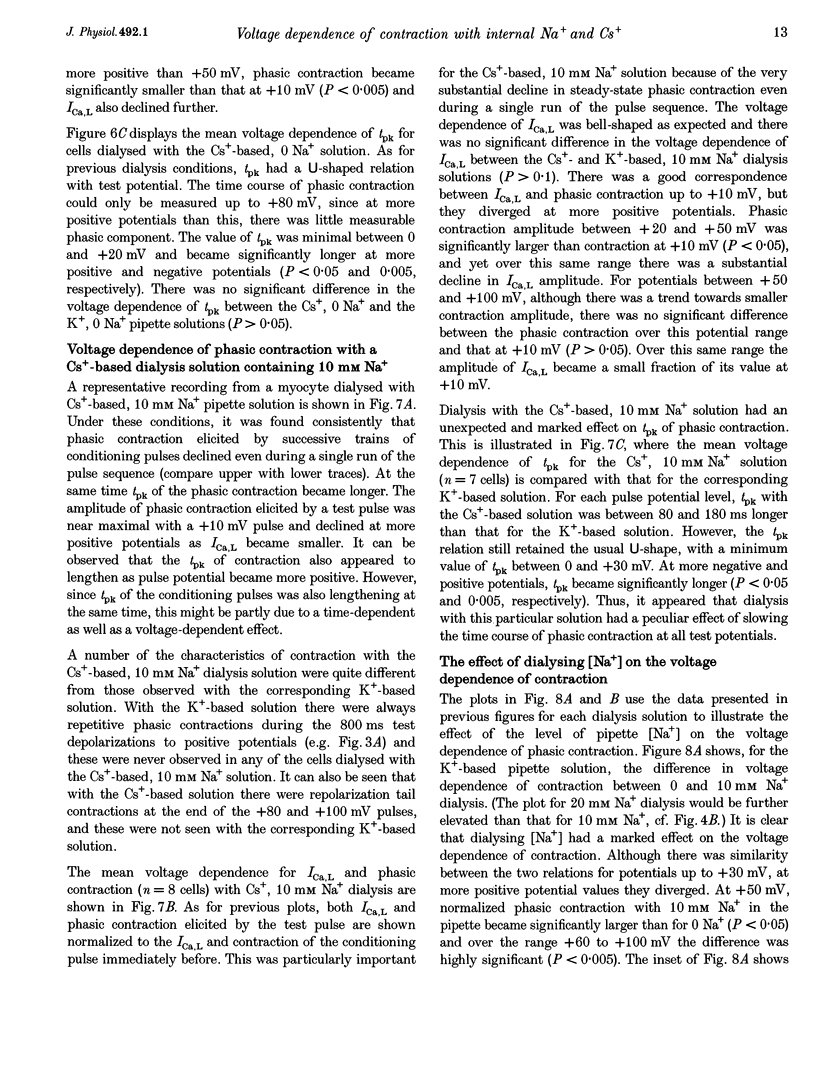
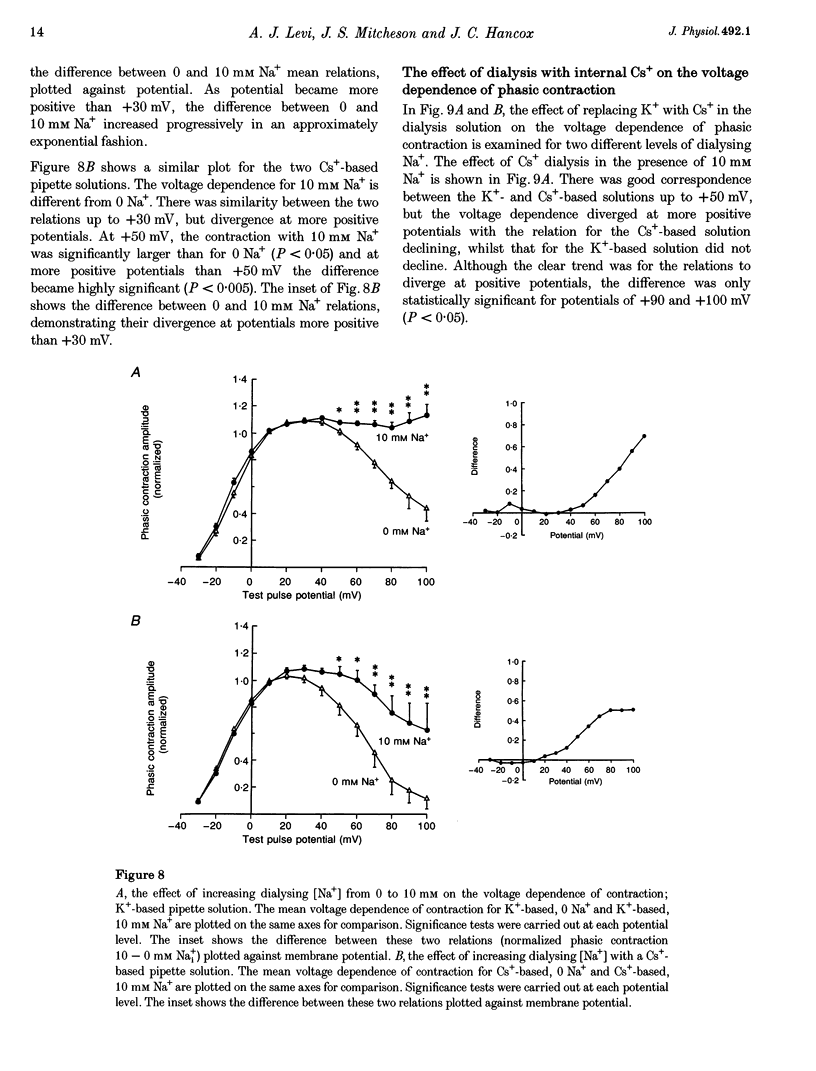
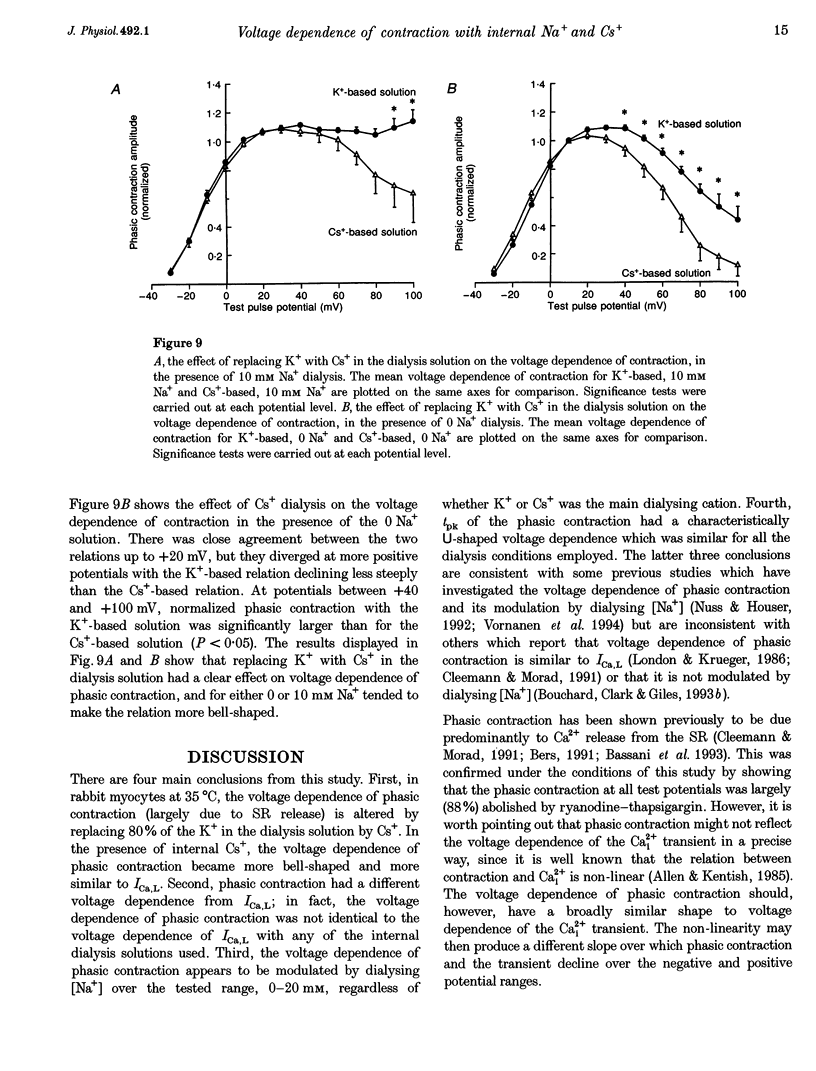
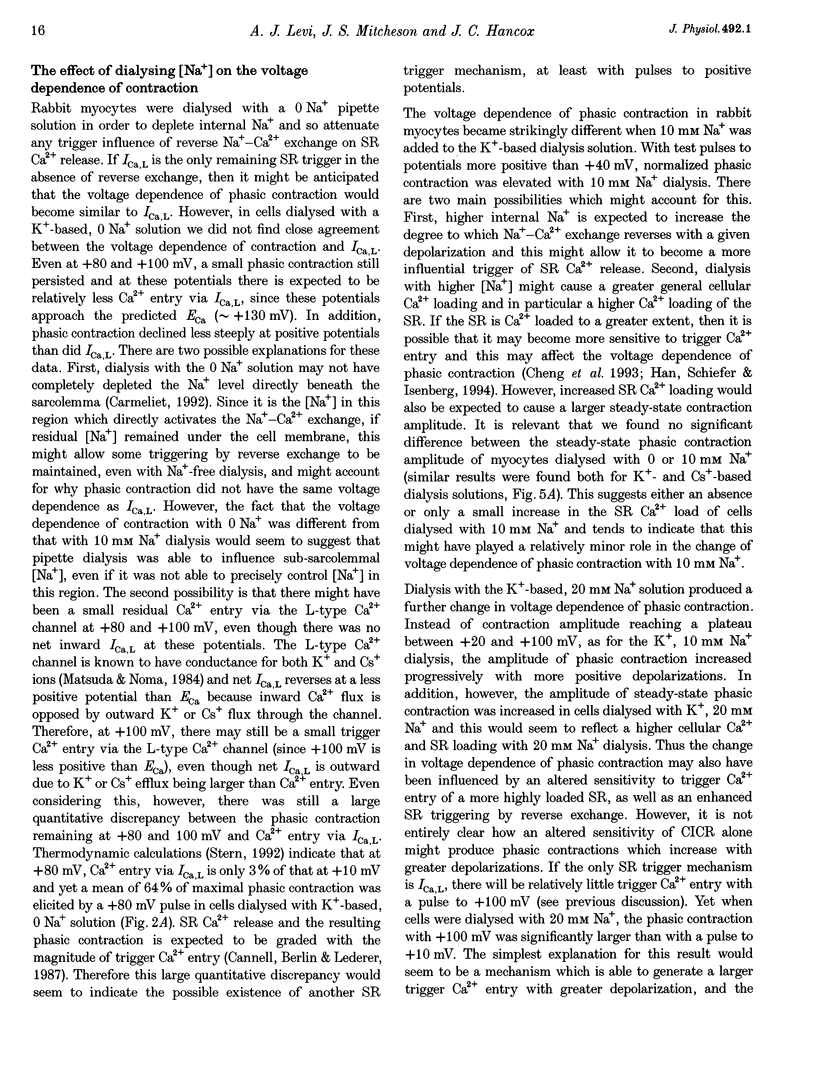
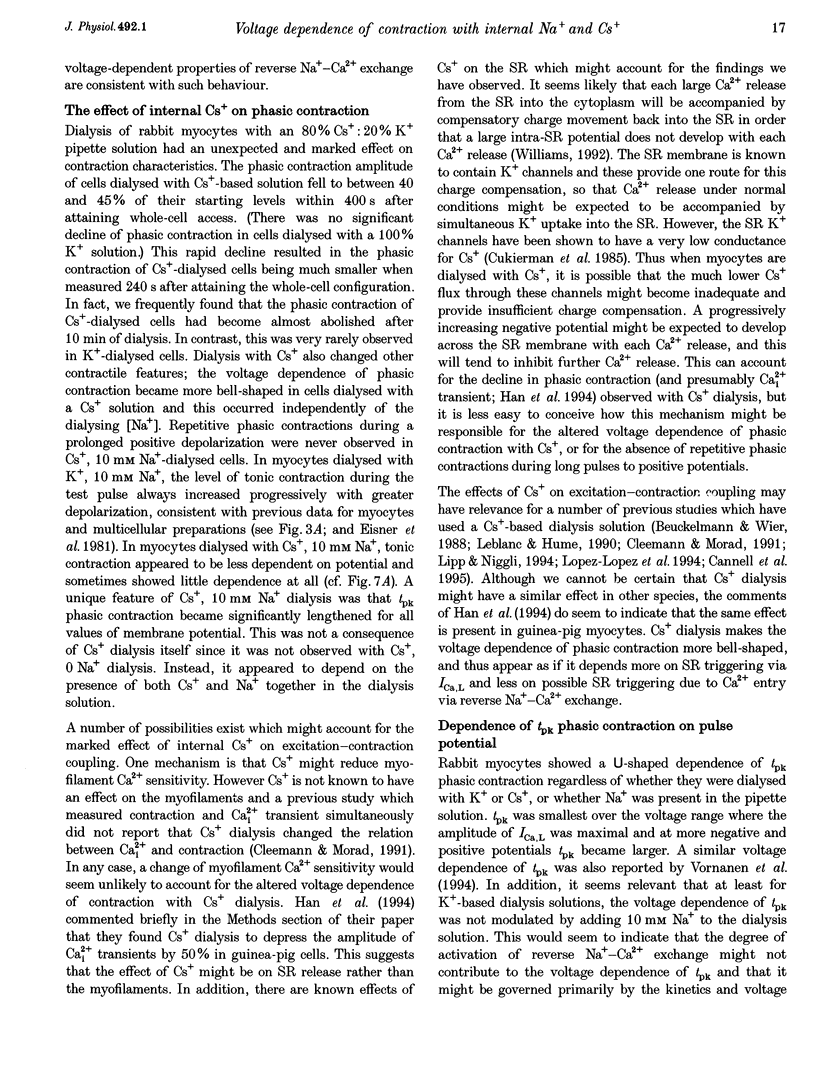
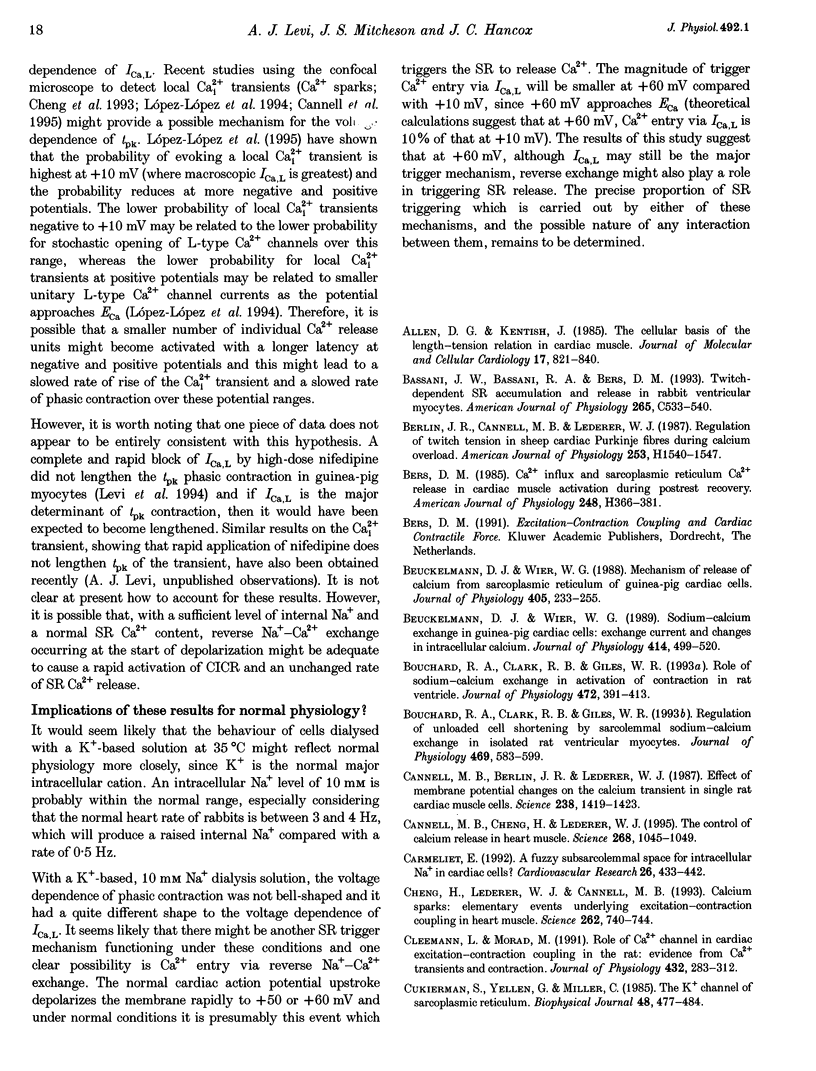
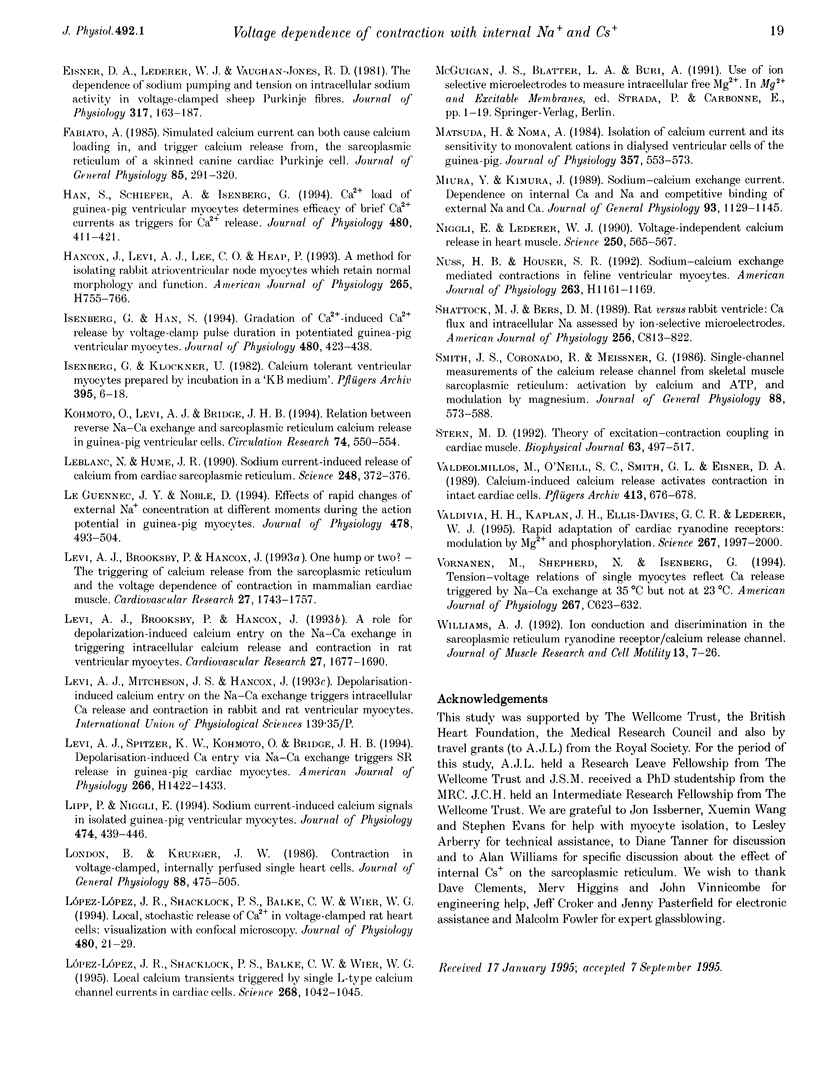
Selected References
These references are in PubMed. This may not be the complete list of references from this article.
- Allen D. G., Kentish J. C. The cellular basis of the length-tension relation in cardiac muscle. J Mol Cell Cardiol. 1985 Sep;17(9):821–840. doi: 10.1016/s0022-2828(85)80097-3. [DOI] [PubMed] [Google Scholar]
- Bassani J. W., Bassani R. A., Bers D. M. Twitch-dependent SR Ca accumulation and release in rabbit ventricular myocytes. Am J Physiol. 1993 Aug;265(2 Pt 1):C533–C540. doi: 10.1152/ajpcell.1993.265.2.C533. [DOI] [PubMed] [Google Scholar]
- Berlin J. R., Cannell M. B., Lederer W. J. Regulation of twitch tension in sheep cardiac Purkinje fibers during calcium overload. Am J Physiol. 1987 Dec;253(6 Pt 2):H1540–H1547. doi: 10.1152/ajpheart.1987.253.6.H1540. [DOI] [PubMed] [Google Scholar]
- Bers D. M. Ca influx and sarcoplasmic reticulum Ca release in cardiac muscle activation during postrest recovery. Am J Physiol. 1985 Mar;248(3 Pt 2):H366–H381. doi: 10.1152/ajpheart.1985.248.3.H366. [DOI] [PubMed] [Google Scholar]
- Beuckelmann D. J., Wier W. G. Mechanism of release of calcium from sarcoplasmic reticulum of guinea-pig cardiac cells. J Physiol. 1988 Nov;405:233–255. doi: 10.1113/jphysiol.1988.sp017331. [DOI] [PMC free article] [PubMed] [Google Scholar]
- Beuckelmann D. J., Wier W. G. Sodium-calcium exchange in guinea-pig cardiac cells: exchange current and changes in intracellular Ca2+. J Physiol. 1989 Jul;414:499–520. doi: 10.1113/jphysiol.1989.sp017700. [DOI] [PMC free article] [PubMed] [Google Scholar]
- Bouchard R. A., Clark R. B., Giles W. R. Regulation of unloaded cell shortening by sarcolemmal sodium-calcium exchange in isolated rat ventricular myocytes. J Physiol. 1993 Sep;469:583–599. doi: 10.1113/jphysiol.1993.sp019831. [DOI] [PMC free article] [PubMed] [Google Scholar]
- Bouchard R. A., Clark R. B., Giles W. R. Role of sodium-calcium exchange in activation of contraction in rat ventricle. J Physiol. 1993 Dec;472:391–413. doi: 10.1113/jphysiol.1993.sp019953. [DOI] [PMC free article] [PubMed] [Google Scholar]
- Cannell M. B., Berlin J. R., Lederer W. J. Effect of membrane potential changes on the calcium transient in single rat cardiac muscle cells. Science. 1987 Dec 4;238(4832):1419–1423. doi: 10.1126/science.2446391. [DOI] [PubMed] [Google Scholar]
- Cannell M. B., Cheng H., Lederer W. J. The control of calcium release in heart muscle. Science. 1995 May 19;268(5213):1045–1049. doi: 10.1126/science.7754384. [DOI] [PubMed] [Google Scholar]
- Carmeliet E. A fuzzy subsarcolemmal space for intracellular Na+ in cardiac cells? Cardiovasc Res. 1992 May;26(5):433–442. doi: 10.1093/cvr/26.5.433. [DOI] [PubMed] [Google Scholar]
- Cheng H., Lederer W. J., Cannell M. B. Calcium sparks: elementary events underlying excitation-contraction coupling in heart muscle. Science. 1993 Oct 29;262(5134):740–744. doi: 10.1126/science.8235594. [DOI] [PubMed] [Google Scholar]
- Cleemann L., Morad M. Role of Ca2+ channel in cardiac excitation-contraction coupling in the rat: evidence from Ca2+ transients and contraction. J Physiol. 1991 Jan;432:283–312. doi: 10.1113/jphysiol.1991.sp018385. [DOI] [PMC free article] [PubMed] [Google Scholar]
- Cukierman S., Yellen G., Miller C. The K+ channel of sarcoplasmic reticulum. A new look at Cs+ block. Biophys J. 1985 Sep;48(3):477–484. doi: 10.1016/S0006-3495(85)83803-0. [DOI] [PMC free article] [PubMed] [Google Scholar]
- Eisner D. A., Lederer W. J., Vaughan-Jones R. D. The dependence of sodium pumping and tension on intracellular sodium activity in voltage-clamped sheep Purkinje fibres. J Physiol. 1981 Aug;317:163–187. doi: 10.1113/jphysiol.1981.sp013819. [DOI] [PMC free article] [PubMed] [Google Scholar]
- Fabiato A. Simulated calcium current can both cause calcium loading in and trigger calcium release from the sarcoplasmic reticulum of a skinned canine cardiac Purkinje cell. J Gen Physiol. 1985 Feb;85(2):291–320. doi: 10.1085/jgp.85.2.291. [DOI] [PMC free article] [PubMed] [Google Scholar]
- Han S., Schiefer A., Isenberg G. Ca2+ load of guinea-pig ventricular myocytes determines efficacy of brief Ca2+ currents as trigger for Ca2+ release. J Physiol. 1994 Nov 1;480(Pt 3):411–421. doi: 10.1113/jphysiol.1994.sp020371. [DOI] [PMC free article] [PubMed] [Google Scholar]
- Hancox J. C., Levi A. J., Lee C. O., Heap P. A method for isolating rabbit atrioventricular node myocytes which retain normal morphology and function. Am J Physiol. 1993 Aug;265(2 Pt 2):H755–H766. doi: 10.1152/ajpheart.1993.265.2.H755. [DOI] [PubMed] [Google Scholar]
- Isenberg G., Han S. Gradation of Ca(2+)-induced Ca2+ release by voltage-clamp pulse duration in potentiated guinea-pig ventricular myocytes. J Physiol. 1994 Nov 1;480(Pt 3):423–438. doi: 10.1113/jphysiol.1994.sp020372. [DOI] [PMC free article] [PubMed] [Google Scholar]
- Kohomoto O., Levi A. J., Bridge J. H. Relation between reverse sodium-calcium exchange and sarcoplasmic reticulum calcium release in guinea pig ventricular cells. Circ Res. 1994 Mar;74(3):550–554. doi: 10.1161/01.res.74.3.550. [DOI] [PubMed] [Google Scholar]
- Le Guennec J. V., Noble D. Effects of rapid changes of external Na+ concentration at different moments during the action potential in guinea-pig myocytes. J Physiol. 1994 Aug 1;478(Pt 3):493–504. doi: 10.1113/jphysiol.1994.sp020268. [DOI] [PMC free article] [PubMed] [Google Scholar]
- Leblanc N., Hume J. R. Sodium current-induced release of calcium from cardiac sarcoplasmic reticulum. Science. 1990 Apr 20;248(4953):372–376. doi: 10.1126/science.2158146. [DOI] [PubMed] [Google Scholar]
- Levi A. J., Brooksby P., Hancox J. C. A role for depolarisation induced calcium entry on the Na-Ca exchange in triggering intracellular calcium release and contraction in rat ventricular myocytes. Cardiovasc Res. 1993 Sep;27(9):1677–1690. doi: 10.1093/cvr/27.9.1677. [DOI] [PubMed] [Google Scholar]
- Levi A. J., Brooksby P., Hancox J. C. One hump or two? The triggering of calcium release from the sarcoplasmic reticulum and the voltage dependence of contraction in mammalian cardiac muscle. Cardiovasc Res. 1993 Oct;27(10):1743–1757. doi: 10.1093/cvr/27.10.1743. [DOI] [PubMed] [Google Scholar]
- Levi A. J., Spitzer K. W., Kohmoto O., Bridge J. H. Depolarization-induced Ca entry via Na-Ca exchange triggers SR release in guinea pig cardiac myocytes. Am J Physiol. 1994 Apr;266(4 Pt 2):H1422–H1433. doi: 10.1152/ajpheart.1994.266.4.H1422. [DOI] [PubMed] [Google Scholar]
- Lipp P., Niggli E. Sodium current-induced calcium signals in isolated guinea-pig ventricular myocytes. J Physiol. 1994 Feb 1;474(3):439–446. doi: 10.1113/jphysiol.1994.sp020035. [DOI] [PMC free article] [PubMed] [Google Scholar]
- López-López J. R., Shacklock P. S., Balke C. W., Wier W. G. Local calcium transients triggered by single L-type calcium channel currents in cardiac cells. Science. 1995 May 19;268(5213):1042–1045. doi: 10.1126/science.7754383. [DOI] [PubMed] [Google Scholar]
- López-López J. R., Shacklock P. S., Balke C. W., Wier W. G. Local, stochastic release of Ca2+ in voltage-clamped rat heart cells: visualization with confocal microscopy. J Physiol. 1994 Oct 1;480(Pt 1):21–29. doi: 10.1113/jphysiol.1994.sp020337. [DOI] [PMC free article] [PubMed] [Google Scholar]
- Matsuda H., Noma A. Isolation of calcium current and its sensitivity to monovalent cations in dialysed ventricular cells of guinea-pig. J Physiol. 1984 Dec;357:553–573. doi: 10.1113/jphysiol.1984.sp015517. [DOI] [PMC free article] [PubMed] [Google Scholar]
- Miura Y., Kimura J. Sodium-calcium exchange current. Dependence on internal Ca and Na and competitive binding of external Na and Ca. J Gen Physiol. 1989 Jun;93(6):1129–1145. doi: 10.1085/jgp.93.6.1129. [DOI] [PMC free article] [PubMed] [Google Scholar]
- Niggli E., Lederer W. J. Voltage-independent calcium release in heart muscle. Science. 1990 Oct 26;250(4980):565–568. doi: 10.1126/science.2173135. [DOI] [PubMed] [Google Scholar]
- Shattock M. J., Bers D. M. Rat vs. rabbit ventricle: Ca flux and intracellular Na assessed by ion-selective microelectrodes. Am J Physiol. 1989 Apr;256(4 Pt 1):C813–C822. doi: 10.1152/ajpcell.1989.256.4.C813. [DOI] [PubMed] [Google Scholar]
- Smith J. S., Coronado R., Meissner G. Single channel measurements of the calcium release channel from skeletal muscle sarcoplasmic reticulum. Activation by Ca2+ and ATP and modulation by Mg2+. J Gen Physiol. 1986 Nov;88(5):573–588. doi: 10.1085/jgp.88.5.573. [DOI] [PMC free article] [PubMed] [Google Scholar]
- Stern M. D. Theory of excitation-contraction coupling in cardiac muscle. Biophys J. 1992 Aug;63(2):497–517. doi: 10.1016/S0006-3495(92)81615-6. [DOI] [PMC free article] [PubMed] [Google Scholar]
- Valdeolmillos M., O'Neill S. C., Smith G. L., Eisner D. A. Calcium-induced calcium release activates contraction in intact cardiac cells. Pflugers Arch. 1989 Apr;413(6):676–678. doi: 10.1007/BF00581820. [DOI] [PubMed] [Google Scholar]
- Valdivia H. H., Kaplan J. H., Ellis-Davies G. C., Lederer W. J. Rapid adaptation of cardiac ryanodine receptors: modulation by Mg2+ and phosphorylation. Science. 1995 Mar 31;267(5206):1997–2000. doi: 10.1126/science.7701323. [DOI] [PMC free article] [PubMed] [Google Scholar]
- Vornanen M., Shepherd N., Isenberg G. Tension-voltage relations of single myocytes reflect Ca release triggered by Na/Ca exchange at 35 degrees C but not 23 degrees C. Am J Physiol. 1994 Aug;267(2 Pt 1):C623–C632. doi: 10.1152/ajpcell.1994.267.2.C623. [DOI] [PubMed] [Google Scholar]
- Williams A. J. Ion conduction and discrimination in the sarcoplasmic reticulum ryanodine receptor/calcium-release channel. J Muscle Res Cell Motil. 1992 Feb;13(1):7–26. doi: 10.1007/BF01738423. [DOI] [PubMed] [Google Scholar]